A preliminary comparison of dendritic cell maturation by total cellular RNA to total cellular lysate derived from breast cancer stem cells
Abstract
Introduction: Dendritic cells (DCs) have been widely considered as the most potent antigen-presenting cells. As such, DC-based vaccines are regarded as a promising strategy in cancer vaccination and therapy. This study compared the maturation of DCs induced by total cellular RNA and cell lysate (i.e. nucleic acid and protein).
Methods: Both total RNA and cell lysate were isolated from breast cancer stem cells (BCSCs). The lysates were used to incubate with monocyte-derived immature DCs. To track the transfection efficiency, the BCSCs were stably transfected with green fluorescent protein (GFP). The maturation of DCs was evaluated by expression of costimulatory molecules including CD40, CD80, and CD86. Transfections were confirmed by evaluating GFP expression in DCs at 24 hours post transfection.
Results: The results of this study showed that GFP is expressed in DCs after both total RNA and lysate incubation. The expression of costimulatory molecules (CD40, CD80, and CD86) was significantly higher in RNA-transfected DCs than in cell lysate-primed DCs.
Conclusion: Our findings suggest that total RNA primed BCSCs can be a suitable platform for DC-based vaccine therapy of breast cancer.
Introduction
As the coordinator of innate and adaptive immune responses, dendritic cells (DCs) possess important regulatory functions. These include the unique ability to activate naïve T cells via cytokine production and to guide CD4+ as well as CD8+ T cell activities, via antigen presentation processes. Based on their ability to prime T cells, there have been many strategies developed to incorporate DCs in cancer vaccines, especially loading DCs with tumor antigens to prime T cell immunity. Due to difficulties in identifying the appropriate tumor antigens, which can be tumor specific antigens (TSA) or tumor-associated antigens (TAA), recent studies are turning to tumor cell lysates and other types of antigenic information (e.g. RNA), with which DCs can be loaded to trigger and to optimize antigen presentation to T cells Fields et al., 1998Nencioni et al., 2003.
Unlike peptide antigens, tumor cell lysates allows for targeting of all proteins expressed by the tumor cells, while effectively activating antigen processing facilitated by DCs. Research has shown that damaged and dying cells can facilitate antigen uptake by DCs, through the expression of molecules called Danger Associated Molecular Patterns (DAMPs). DAMPs consist of heat shock proteins (e.g. HSP 70/90), plasma membrane components, and degraded DNA and RNA, depending on the pathway of cell death. However, the use of tumor cell lysates comes with certain disadvantages. For instance, DAMPs can be either immunogenic or immunosuppressive, which means the induced maturation of DCs is just partial. In the case of immunogenic DAMPs, antigen presentation by MHC II is activated via receptors that cluster in clathrin-coated domains. Cross-presentation (MHC I pathway) also takes place when some antigens are endocytosed via mannose receptors of DCs. As a result, DCs process antigen from tumor cell lysates through both MHC I and MHC II pathways; however, it has been reported that CD8+ T cell responses are lower frequency compared to CD4+ T cell responses, which can be considered as a drawback Blum et al., 2013Chiang et al.,2015Herr et al., 2000Kalinski et al., 2009Nierkens and Janssen, 2011.
Loading DCs with mRNA is another strategy that has gained significant attention. The mRNA makes its cellular entry in an unknown mechanism, which some studies have suggested to be similar to scavengerreceptor endocytosis and macropinocytosis. Loading DCs with mRNA activates pattern recognition receptors, such as TLR3 and TLR7, as well as endogenous antigens expressed by DC. Taken together, these activation signals improve DC maturation. The antigenic material is then processed in an endogenous manner. While it is clear that DCs loaded with mRNA would preferentially process antigen via MHC I pathway, enormous efforts to couple antigenic epitopes to class II signaling sequences have shown robust stimulation of both CD4+ and CD8+ T cells Benteyn et al., 2014Schlake et al., 2012Van Lint et al., 2014.
Cell lysate and mRNA are both useful for the development and application DC-based vaccines, and each has advantages and disadvantages. This study was conducted to compare the effectiveness of inducing DC maturation using tumor cell lysate or RNA. The main endpoints evaluated include the efficacy of antigen uptake by DC and the expression level of specific molecules related to DC maturation (e.g. costimulatory molecules). To assesss the basic difference between strategies, no specific antigen was used; instead, total RNA and total protein were used as induction factors. Total protein was derived from necrotic cell lysate and pulsed into iDCs; total RNA was either pulsed or transfected into iDCs.
Materials and methods
Reagents
DMEM/F12 and TRI Reagent (Sigma-Aldrich, Missouri, US), Fetal Bovine Serum and Trypsin/EDTA (Gibco, Massachusetts, US), Ficoll-Paque PLUS (GE Healthcare, Little Chalfont, UK), RPMI 1640 (Sigma- Aldrich, Missouri, US), FuGENE HD transfection reagent (Promega, Wisconsin, US), and Opti-MEM (Life Technologies, California , US).
Antibodies and cytokines
FITC-conjugated mouse anti-human CD14, Mouse monoclonal anti-human CD40, Mouse monoclonal anti-human CD80, FITC-conjugated mouse antihuman CD86, FITC-conjugated rabbit anti-mouse IgG1 (Santa Cruz Biotechnology, California, US); GMCSF, IL-4, and TNF-α (Sigma-Aldrich, Missouri, US).
Cell lines
VNBCSC cells were isolated and previously established in our laboratory (Laboratory of Stem Cell Research and Application; University of Science; Vietnam National University; Ho Chi Minh city, Viet Nam); their cell markers and stem cell characteristics have been previously defined. VNBRCA-1-gfp cell line was established from VNBRCA cells which were stably transfected with GFP; cells were selected by 5μg/ml Puromycin and more than 90% of the cells were positive for GFP expression.
Isolation of monocyte from umbilical cord blood and preparation of immature DC (iDC)
Umbilical cord blood was collected at the hospital with informed consents from donors and processed for monocyte isolation and differentiation into DCs. Monocyte-derived DCs were generated by a 7-day process. Briefly, umbilical cord blood was diluted by 1:2 with PBS and layered on Ficoll-Paque PLUS with a volume ratio of 2:1 in a total volume of 12 mL. After 30 minutes of centrifugation at 800 g, monocytes were harvested from the interphase and treated with erythrocyte lysis buffer to eliminate erythrocytes. For removal of platelets, the cell pellet was resuspended in PBS and centrifugated at 600 g for 6 minutes twice. Isolated monocytes were later cultured in RPMI-1640 medium with IL-4 and GM-CSF for 7 days. The iDCs were harvested and plated in 24-well plates at 2x105 cells/well for experiments.
Transfection and passive pulsing of iDCs with total RNA from breast cancer stem cells
Total RNA was extracted from breast cancer stem cells with TRI Reagent and conserved in deionized water. Transfection was performed with FuGENE HD transfection reagent in Opti-MEM medium, following a procedure recommended by the manufacturer. Briefly, 300 ng of total RNA was transfected into 2x105 cells, using RNA: FuGENE ratio of 1:3. Subsequently, cells were washed after 1 hour and resuspended in RPMI 1640 medium, supplemented with IL-4 and GM-CSF. Cells then were incubated at 37°C. RNA passive pulsing was performed in the same condition as RNA transfection, with the substitution of FuGENE HD by Opti-MEM medium. GFP expression was assessed for DCs transfected with total RNA of VNBRCA-1-gfp cells at 24 h after transfection or pulsing; analysis was done by flow cytometry on an FACSCalibur (BD Bioscience, US).
Protein pulsing of iDCs
Necrotic antigen was harvested following necrotic cell lysis by freezing in 7 minutes/ thawing in 7 minutes of breast cancer stem cells; the necrotic cell lysate was collected in the cell pellet by centrifugation. The iDCs were co-incubated with 10 μg/ml necrotic cell lysate in RPMI 1640 medium supplemented with IL-4 and GMCSF at 37°C. GFP expression of DCs was analyzed by FACSCalibur for cells pulsed with necrotic cell lysate of VNBRCA-1-gfp at 24 h after pulsing.
Immunostaining
Cell staining was performed 1 day after induction of mature DCs, using FITC-conjugated mouse monoclonal antibodies against CD14 and CD86. Appropriate FITC-conjugated rabbit anti-mouse IgG isotypes were used to evaluate anti-human CD40 and anti-human CD80. Samples were analyzed by flow cytometry on an FACSCalibur.
Statistic Analysis
Data analysis was performed using two-way ANOVA with Tukey’s multiple comparisons test using GraphPad Prism 6.0 Software. All experiments were repeated three times and represented as mean ± SD.
Results
VNBRCA-1-gfp selection and cell culture
The VNBRCA-1-gfp cell line was selected by Puromycin at a concentration of 5μg/ml to obtain a a high GFP-expressing cell population to be used for assessment of antigen loading. After one week of selection, the VNBRCA-1-gfp cell line yielded a high rate of GFP expression, with over 90% of the cells expressing detectable GFP ( Figure 1 ).
After 7 days of culture in RPMI 1640 medium supplemented with 10 ng/mL IL-4 and 10ng/mL GMCSF (Gibco, Invitrogen), monocytes isolated from umbilical cord blood showed many morphological changes from the initial state. The round shape of monocytes had gradually developed into dendrites (tree-like branches) on day 7 ( Figure 2 ).
Analysis of immature DC markers was also performed by Flow Cytometry on the FACSCalibur. The iDCs showed a decrease in CD14 expression; only 20.2 ± 7.8% of the population were positive for CD14, while the monocyte population was about 43.5 ± 5.7% positive. Costimulatory molecule expression levels of CD40, CD80, and CD86 were expressed by 37.7 ± 7.8%, 21.8 ± 2.7% and 5.2 ± 1.5% of the cell population, respectively; there were slight increases in expression of these molecules in the iDCs compared to the monocyte population ( Figure 3 ).
GFP expression in DCs after transfection and pulsing
Immature DCs harvested on day 7 were seeded in 24- well plates at a density of 2 x 105 cells/plate to assess the preliminary efficacy of antigen loading by the methods (protein or RNA). Both types of antigenic information (protein, RNA) were obtained from VNBRCA-1-gfp cells; the GFP signal was useful to help determine the rate of antigen uptake induced by each method. Protein pulsing strategy was compared with RNA strategy (which involved passive pulsing or lipofection via the FuGENE HD transfection reagent). The volume of necrotic cell lysate coincubated with iDCs was defined by the ratio of volume of 300 ng of total RNA to total RNA volume extracted from the same number of cells in the beginning. Flow Cytometry was performed 18 hours after loading of antigen into DC to detect GFP expression. Of the DCs loaded with RNA by passive pulsing, approximately 4.4 ± 1.6% were GFP positive; of those loaded with necrotic cell lysate, 3.3 ± 0.8% were GFP positive, and of those transfected with RNA by FuGENE method, 2.7 ± 1.7% were GFP positive ( Figure 4 ). Statistic analyses, however, showed no significant differences among the samples.
Maturation of DCs induced by total RNA and protein loading
To investigate how effectively DCs could be induced to mature by varying methods, we assessed the expression of CD14 (a known marker of dendritic cell precursors and monocytes) and costimulatory receptors, CD40, CD80 and CD86 (known markers which increase during DC maturation). DC maturation was induced by the use of a standard cytokine cocktail (IL-4, GM-CSF, and TNF-α in RPMI 1640 medium), which has been used to induce maturation in many previous studies.
In Figure 5 , expression of CD14 did not vary significantly among the iDCs when induced by lysate or RNA. However, a significant decrease was observed in those samples in comparison to monocyte or iDC samples. This demonstrates that the DCs differentiated from a precursor or immature state. With regard to costimulatory receptors, there was an overall increased expression of costimulatory receptors on the primed DCs (by the varying methods), as compared to monocytes and iDCs. Of all the methods, RNA transfection by FuGENE HD yielded the highest expression of CD40 and a relatively high expression of CD80 and CD86. Protein pulsing with necrotic cell lysate was inferior to the RNA loading methods, yielding very low expression of costimulatory receptors. Therefore, RNA loading of DC was a superior strategy for antigen loading of DCs. Finally, as expected, the traditional method of inducing DC maturation (cytokine cocktail) yielded high expression of CD40, CD80 and CD86.
Discussion
This study aimed to compare the effectiveness of varying methods to induce DC maturation. The methods included the use of necrotic cell lysate (protein pulsing) and use of total RNA (passive pulsing or transfection). Understanding which methods are most effective at priming DC maturation is important for DC-based cancer vaccination strategies. The results of this study suggest that RNA loading of DCs potently induce DC maturation, as represented by high expression levels of costimulatory molecules, and is a better strategy than protein pulsing.
Nevertheless, GFP expressions in DCs loaded with necrotic cell lysate and total RNA from VNBRCA-1- gfp showed no statistically significant difference, which means antigen loading of DCs induced maturation at varying levels, although they were considerably similar. Analysis of the flow dot plot results showed variation in the signal of detectable GFP ( Figure 6 ); in samples where iDCs were loaded with RNA by FuGENE transfection, the intensity of the detected signal was higher than that of other samples, emphasizing the significant difference in GFP expression levels between the negative and positive populations. Meanwhile, the GFP signal detected in samples loaded with necrotic cell lysate or RNA, by passive pulsing, was close to the threshold of detection. Combining these observations, we can infer that the antigen loading rate varied among samples. Moreover, transfection of total RNA by FuGENE HD was the most effective method to load antigen onto DCs.
Our observations show strong correlation with previous studies on antigen loading of DC with cell lysate or RNA. When DCs are loaded with RNA, it allows them to be fully activated due to signal transduction signals via TLR3 and TLR7. Moreover, RNA loading circumvents potentially immunosuppressive signals of DAMPs in necrotic cell lysates. Thus, transfection of RNA by a transfection agent promotes DC maturation since it introduces RNA into the DC cytosol. FuGENE HD may also act to protect RNA molecules, in the extracellular environment, from ubiquitous RNase, and then gradually releases them into the cytosol Benteyn et al., 2014Schlake et al., 2012Van Lint et al., 2014.
In contrast to the high expression of CD40 and CD80, CD86 expression was relatively low, even in the case of samples induced with RNA transfection or with cytokine cocktails. Studies have suggested that during the maturation process, DCs express costimulatory receptor CD86 later than CD40 or CD80. Further research is needed to evaluate DC maturation through CD86 expression Fleischer et al., 1996.
Conclusion
The results of our study demonstrate the superiority of RNA loading, versus loading with necrotic cell lysate, on induction of DC maturation. Using the FuGENE HD transfection reagent, DCs loaded with total RNA, derived from breast cancer stem cells, exhibited high expression of costimulatory receptors CD40 and CD80. Moreover, CD86 expression was significantly higher for RNA-primed DCs than for DCs loaded with necrotic cell lysate. Further investigations are needed to assess the properties of the transduced DCs, including their MHCII epxression levels after RNA transfection, and to understand the functional polarization of induced mature DCs.
Abbreviations
CD: Cluster of differentiation
DAMPs: Danger Associated Molecular Patterns
DCs: Dendritic cells
GFP: Green fluorescent protein
GM-CSF: Granulocyte macrophage colony stimulating factor
iDC: Immature dendritic cells
IL-4: Interleukin 4
MHCII: Major histocompatibility complex class II
RNA: Ribonucleic acid
TLR: Toll like receptor
TNF: Tumor necrosis factor
Ethics Approval and Consent to Participate
All umbilical cord blood samples were donored with consent form, and approved by hospital local committee.
References
-
D.
Benteyn,
C.
Heirman,
A.
Bonehill,
K.
Thielemans,
K.
Breckpot.
mRNA-based dendritic cell vaccines. Expert Review of Vaccines.
2014;
14
:
161-176
.
-
J.S.
Blum,
P.A.
Wearsch,
P.
Cresswell.
Pathways of Antigen Processing. nnual Review of Immunology.
2013;
31
:
443-473
.
-
C.
Chiang,
G.
Coukos,
L.
Kandalaft.
Whole Tumor Antigen Vaccines: Where Are We?. Vaccines.
2015;
3
:
344-372
.
-
R.
Fields,
K.
Shimizu,
J.
Mule.
Murine dendritic cells pulsed with whole tumor lysates mediate potent antitumor immune responses in vitro and in vivo. roceedings of the National Academy of Sciences.
1998;
95
:
9482-9487
.
-
J.
Fleischer,
E.
Soeth,
N.
Reiling,
E.
Grage-Griebenow,
H.D.
Flad,
M.
Ernst.
Differential expression and function of CD80 (B7-1) and CD86 (B7-2) on human peripheral blood monocytes. Immunology.
1996;
89
:
592-598
.
-
W.
Herr,
E.
Ranieri,
W.
Olson,
H.
Zarour,
L.
Gesualdo,
W.J.
Storkus.
Mature dendritic cells pulsed with freeze- thaw cell lysates define an effective in vitro vaccine designed to elicit EBV-specific CD4+ and CD8+ T lymphocyte responses. Blood.
2000;
96
:
1857-1864
.
-
P.
Kalinski,
J.
Urban,
R.
Narang,
E.
Berk,
E.
Wieckowski,
R.
Muthuswamy.
Dendritic cell-based therapeutic cancer vaccines: what we have and what we need. uture Oncology.
2009;
5
:
379-390
.
-
A.
Nencioni,
M.R.
Müller,
F.
Grünebach,
A.
Garuti,
M.C.
Mingari,
F.
Patrone,
A.
Ballestrero,
P.
Brossart.
Dendritic cells transfected with tumor RNA for the induction of antitumor CTL in colorectal cancer. Cancer Gene Therapy.
2003;
10
:
209-214
.
-
S.
Nierkens,
E.M.
Janssen.
Harnessing Dendritic Cells for Tumor Antigen Presentation. Cancers.
2011;
3
:
2195-2213
.
-
T.
Schlake,
A.
Thess,
M.
Fotin-Mleczek,
K.-J.
Kallen.
Developing mRNA-vaccine technologies. RNA Biology.
2012;
9
:
1319-1330
.
-
S.
Van Lint,
D.
Renmans,
K.
Broos,
H.
Dewitte,
I.
Lentacker,
C.
Heirman,
K.
Breckpot,
K.
Thielemans.
The Re- NAissanCe of mRNA-based cancer therapy. xpert Review of Vaccines.
2014;
14
:
235-251
.
Comments
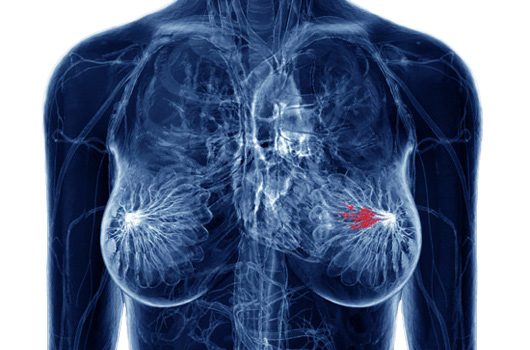
Downloads
Article Details
Volume & Issue : Vol 3 No 06 (2016)
Page No.: 679-686
Published on: 2016-06-26
Citations
Copyrights & License

This work is licensed under a Creative Commons Attribution 4.0 International License.
Search Panel
Pubmed
Google Scholar
Pubmed
Google Scholar
Pubmed
Google Scholar
Pubmed
Search for this article in:
Google Scholar
Researchgate
- HTML viewed - 6498 times
- Download PDF downloaded - 1739 times
- View Article downloaded - 8 times