Taraxacum officinale dandelion extract efficiently inhibited the breast cancer stem cell proliferation
Abstract
Background: Breast cancer stem cells (BCSCs) play an important role in breast cancer initiation, metastasis, recurrence, and drug resistance. Therefore, targeting BCSCs is an essential strategy to suppress cancer growth. This study aimed to evaluate the effects of dandelion Taraxacum officinale extracts on BCSC proliferation in vitro in 2D and 3D cell culture platforms.
Materials and Methods: The BCSCs were maintained under standard conditions, verified for expression of CD44 and CD24 surface markers, and transfected with GFP before use in experiments. In the 2D model, the BCSCs were cultured as adherent cells in standard culture plates; in the 3D model, the BCSCs were cultured on low-adherent plates to form spheroids. The effect of Dandelion extracts on proliferation of BCSC was assessed by evaluating induction of cell death, expression of genes of death receptor signaling pathways, and production of reactive oxygen species (ROS) by BCSCs.
Results: BCSCs formed spheroids as microtumors in vitro and exhibited some in vivo characteristics of tumors, such as increased expression of N-cadherin and Slug, decreased expression of E-cadherin, capacity to invade into the extracellular matrix (ECM), and presence of a hypoxic environment at the core of tumor spheroids. The dandelion extracts significantly inhibited BCSC proliferation in both two-dimensional (2D) and three-dimensional (3D) models of BCSCs. However, the IC50 value of dandelion extracts in BCSCs in the 3D model was much higher than that in the 2D model. The results also demonstrated that BCSCs treated with Dandelion extracts showed increased expression of tumor necrosis factor-related apoptosis-inducing ligand (TRAIL) and TRAIL receptor 2 (TRAILR2; i.e. death receptor 5; DR5). Moreover, treatment induced expression of DR4. Treatment with methanol dandelion extract enhanced production of ROS in BCSCs.
Conclusion: Dandelion extracts are promising extracts for the treatment of breast tumors. The effect of methanol extract (with dandelion extract) was better than that for ethanol extract. Importantly, BCSCs in 3D exhibited stronger drug resistance than those in 2D. In summary, our results indicate the strong potential of dandelion extracts as anti-cancer agents and rational use for drug development.
Introduction
Cancer is one of the leading causes of death worldwide. According to the International Agency for Research on Cancer (IARC), approximately 14.1 million of new cases of cancer occurred in 184 countries worldwide during 2012, of which 8.2 million cases were non-survivors and 32.6 million cases were those living with cancer (diagnosed within five years) Organization, 2012. Specifically, breast cancer accounted for about 25% of all cancer types worldwide and was found to be the most prevalent cancer in women worldwide International, 2012.
During the past few decades, many novel therapies for breast cancer have been developed, including radiation therapy, chemotherapy, hormonal therapy, and monoclonal antibody targeted therapy. However, few have garnered positive impact. Therefore, there have been many studies directed at investigating new, efficient and affordable cancer therapies, especially in the area of anti-cancer drug development Coseri, 2009. Screening extracts and compounds derived from herbal plants is widely considered to be a rational approach for discovering novel anti-cancer drugs. To date, most in vitro screening studies, based on cultured cancer cells, have utilized a two-dimensional (2D) culture model, which entails an adherent monolayer culture system. However, this model has its limitations, which have led to the high rate of clinical trial failures for new molecules derived from these screenings Edwards et al., 2015Sams-Dodd, 2005. Indeed, cancer cells grown in a 2D culture model lack certain characteristics of in vivo cancer cells, such as cell-cell and cell-matrix interactions Baker and Chen, 2012Kimlin et al., 2013, a hypoxic microenvironment and capacity for drug resistance Wartenberg et al., 2003. In recent years, in vitro 3D culture systems have been developed to fill the gap between conventional 2D in vitro testing models and in vivo animal models Yamada and Cukierman, 2007Zanoni et al., 2016.
Another reason for the clinical trial failures of new molecules has been the lack of understanding of the appropriate cancer targets. In fact, most drug screening studies to identify new or effective anti-cancer agents have been based on immortalized cancer cell lines. Although these cell lines have been widely used for a long time, they have major limitations, namely cross-contamination Wilding and Bodmer, 2014 and loss of tissue specific characteristics Jaeger et al., 2015. Targeting cancer stem cells (CSCs) is considered a strategy which may effectively decrease the failure of clinical trials of anti-cancer drug candidates Wilding and Bodmer, 2014.
One model of CSCs is breast cancer stem cells (BCSCs), which were first discovered by Al-Hajj et al., 2003. These cells exhibit the phenotypic surface profile (positive for CD44, negative for CD24), and play an essential role in breast tumor initiation, progression, chemotherapy resistance, and metastasis Clarke et al.,2006Croker and Allan, 2008Monteiro and Fodde, 2010Perou, 2010Sampieri and Fodde, 2012. Therefore, targeting BCSCs is a promising strategy to treat breast cancer. To date, targeting BCSCs can be carried out by several means, including gene therapy, immune therapy, monoclonal antibody directed therapy, and/or use of phytochemicals/compounds from herbal/natural plants.
In recent years, herbal extracts/natural plant extracts have garnered special interest for CSC targeting studies. Increasing evidence from studies have shown that herbal extracts can induce apoptosis and arrest cell cycle in various cell types, such as BCSCS, without affecting healthy cells. Some recent studies have shown that extracts from dandelion Taraxacum officinale, also known as Dandelion Anticancer, could inhibit the proliferation of human melanoma cells Chatterjee et al., 2011, breast cancer cells, prostate cancer cells Sigstedt et al., 2008, and cervical cancer cells (Ketut Edy Sudiarta, 20165). Studies showed that dandelion extracts could induce cell death via the tumor necrosis factor (TNF)-related apoptosis-inducing ligand (TRAIL) pathway. TRAIL is a member of the TNF super family that can initiate apoptosis by activation of death receptor 4 (DR4/ TRAILR-1) and death receptor 5 (DR5/TRAILR-2). Since TRAIL can induce apoptosis in cancer cells, including breast cancer cells, without causing toxicity to normal cells Chinnaiyan et al., 2000Keane et al., 1999Walczak et al.,1999, strategies which target TRAIL represent safe alternatives for cancer therapy development Abdelhamed et al., 2013.
In our study, we aimed to evaluate the effect of Dandelion extraction on BCSC proliferation, and determine its mechanisms of action. As a new strategy, we used a 3Din vitro model for drug screening. BCSCs were used in place of breast cancer cells.
Materials-methods
Dandelion extracts preparation
A whole Dandelion plant was dried and grinded to a fine powder. The powder was immersed in 96% ethanol and 96% methanol, for 72h, to collect the ethanol extract and methanol extract, respectively. The supernatants were collected by filtrates, then evaporated with a rotary evaporator at 40-50°C under low pressure to collect extracts. All extracts were stored at 4°C. The extract stocks were re-suspended in Dulbecco’s Modified Eagle’s Medium/Ham F12 (DMEM/F12), supplemented with 10% fetal bovine serum (FBS) and 1% antibiotic-antimycotic (all bought from Sigma-Aldrich, St Louis, MO, USA). The volume added for suspension was equivalent to a final concentration of 200mg/ml.
Breast cancer stem cell isolation and proliferation
The human breast cancer stem cell line was isolated via a previous protocol Van Phuc et al., 2010. The cells were transfected with green fluorescent protein (GFP) using a lentiviral vector, as per the previous protocol Van Pham et al., 2012. The GFP-BCSCs were grown in cell culture medium (DMEMF12/10% FBS/1% antibiotic-antimycotic) and incubated at 37°C in a humidified atmosphere with 5% CO2. For the standard 2D culture, cells were cultured in T25 culture flasks and 96-well culture plates (Corning, New York).
3D model - spheroid culture
For generation of tumor spheroids in 3D, 200 μl/well of cell suspension in culture medium was seeded at five different densities (100, 500, 1000, 2500, 5000, or 10000 cells/well). Cells were dispensed into Nunclon Sphera 96 wells plate (Thermo Fisher Scientific, Waltham, MA). The Nunclon Sphera surface is designed to cause minimal cell attachment with minimal extracellular matrix protein binding to the plate surfaces. Plates were incubated at 37°C and 5% CO2. In the 3D culture, propidium iodide (Sigma- Aldrich, St Louis, MO, USA) staining was used to verify the population and location of dead cells in the spheroids.
Cell treatment of extracts
BCSCs were cultured in 2D for 24h, and in 3D for 48h, before use in experiments. Then, cells treated with extracts. Both ethanol and methanol extracts were used as treatments in both 2D and 3D BCSC cultures, at 5 various concentrations: 10μg/ml, 100μg/ml, 500μg/ml, 1000μg/ml, and 2500 μg/ml. Doxorubicin and Tirapazamine treatment served as positive controls. Doxorubicin was used at 0.01 μg/mL, 0.1 μg/mL, 1 μg/mL, 2.5 μg/mL, and 5 μg/mL; Tirapazamine was used at 0.1 μg/mL, 1 μg/mL, 10 μg/mL, 25 μg/mL, and 50 μg/mL.
Cell Viability assay
Cell viability was assessed by AlamarBlue assay (Thermo Fisher Scientific, Waltham, MA), based on the manufacturer’s instructions. Viability of treated cells/spheroids were evaluated at 24h, 48h, and 72h after treatment. At the evaluation points, cells/spheroids were replaced with fresh cell medium without extracts. Then, they were incubated with AlamarBlue working solution for 4 h. Finally, plates were measured for fluorescence intensity at 535 nm excitation and 595 nm emission by a micro-plate reader DTX 880 (Beckman Coulter, Brea, CA). After the IC50 values of the Dandelion extracts on BCSCs were determined, BCSCs were treated with the extract at these IC50 values to evaluate induction of cell death. Cell viability percentage was determined by counting the total cell number stained with trypan blue using a hemocytometer.
Tumor spheroid-based extracellular cell matrix (ECM) gel invasion assay
A total of 100μL media was removed from wells containing 4-d spheroids and 100μL of 4% ECM gel was gently added to the wells. This provides a semisolid gel-like matrix condition. Cells extended their invadopodia into the matrix Stylli et al., 2008. The invasion of BCSC spheroids was identified by fluorescence microscopy (Cart Zeiss, Oberkochen, Germany).
Gene expression assay
Total RNA was extracted using the Easy blue reagent INTRON, according to the manufacturer’s instructions, and stored at -20°C. The first-strand complementary DNA (cDNA) synthesis reaction was performed using a one-step reverse transcriptase polymerase chain reaction (RT-PCR) premix (Intron Biotechnology, Korea) with a MasterCycle PCR apparatus (Eppendorf, Hamburg, Germany). Realtime PCR reactions were performed using qPCRBIO SyGreen one-Step Detect Lo-ROX (PCR BIOSYSTEMS, England) with a Thermal Cycler Real-Time PCR apparatus (Eppendorf, Hamburg, Germany). Quantitative data, including normalized versus GADPH gene, were analyzed using an average of triplicates Ct (cycle threshold), according to the 2–ΔΔct method. The data shown were generated from three independent experiments and the values were expressed relative to mRNA levels in the 2D cells (control), as the mean ± SEM.
For amplification, the following primers were used: GADPH: F 5’-CACCACCATGGAGAAGGCTGG-3’; R 5’-CCAAAGTTGTCATGGATGACC-3’; E-Cadherin: F: 5’-GAAGGTGACAGAGCCTCTGGAT-3’; R: 5’-GATCGGTTACCGTGATCAAAATC-3’; N-Cadherin: F 3’-CCATCACTTAATGGT-5’; R 3’-ACCCACAATCCACAT-5’; Slug: F: 5’-ATTGCCTTGTGTCTGCAAGATCT-3’; R: 5’-TCTGTCTGCAAAAGCCCTATTG-3’; TRAIL: F 5’-CTTCACAGTGCTCCTGCAGT-3’; R 5’-TTAGCCAACTAAAAAGGCCCC-3’; DR-4: F 5’-CTGAGCAACGCAGACTCGCTGTCCAC-3’; R 5’-TCCAAGGACACGGCAGAGCCTGTGCCAT-3’ and DR-5: F 5’-GCCTCATGGACAATGAGATAAAGGTGGCT-3’; R 5’-CCAAATCTCAAAGTACGCACA AACGG-3’.
Luminol-based Chemiluminescence assay
ROS signals were made chemiluminescent by Luminol probe (5mM). For typical measurements of chemiluminescence (CL), 5×104 cells were incubated in 0.2 mL of assay buffer (PBS including 5 mM glucose, 1 mM Mg2+, 0.5 mM Ca2+ and 0.05% BSA) for 3 min before addition of 200 ng/ml Phorbol 12-myristate 13-acetate (PMA). Luminescence signal was monitored for 30 min using the microplate reader DTX 880 (Beckman Coulter, Brea, CA).
Statistical analysis
All data are expressed as mean ± SEM and normalized against the untreated control. Statistical comparison was conducted by one-way ANOVA. All statistical procedures were carried out using GraphPad Prism 6.0 (GraphPad Software Inc., San Diego, CA, USA). *P<0.05, **P<0.01, and ***P<0.001 were considered as significant differences. IC50 value was calculated by the GraphPad Prism 6.0 based on formulation Fifty=(Top+Baseline)/2 and Y= Bottom + (Top-Bottom)/(1+10^((LogIC50-X)*HillSlope + log((Top-Bottom)/(Fifty-Bottom)-1))).
Results
Establishing the 3D model of BCSCs
Under non-adhesive conditions, GFP-BCSC cells automatically and rapidly formed spheroids after 48 h of incubation ( Figure 1 ). The morphology of spheroids were different among the various densities of BCSCs.
Indeed, in the low densities (100 and 500 BCSCs per well), spheroids formed a ball-like shape, while in higher densities BCSCs spheroids formed a grape cluster-like shape ( Figure 1 ).
To choose the optimal density of BCSCs to generate spheroids, the spheroid growth was determined based on diameter measurement ( Figure 2 ) an AlamarBlue assay ( Figure 3 ). The results showed that the diameter of spheroids in the 100, 500, 1000, 2500 and 5000 cells/well groups gradually increased from day 1 to day 10. In the group of 10000 cells/well, the diameter of spheroid rapidly reduced from day 2 onward ( Figure 2 ). The AlamarBlue assay also showed that the fluorescent intensity of BCSCs per well gradually increased from day 1 to day 7, in the 100, 500, 1000, 2500 and 5000 cells/wells groups, while the fluorescent intensity in the 10,000 BCSCs/well group did not increase but rather reduced after day 3. In this assay, we also compared BCSC proliferation in the 3D model vs 2D model. The results showed that at the same cell density (5000 BCSCs/well) the BCSCs in 2D model grew more robustly and rapidly than those in the 3D model. Therefore, as a result, BCSCs cultured in 2D aged sooner than those in 3D ( Figure 3 ).
BCSC spheroids express markers of the EMT process
Based on these results, we chose the cell density of 5000 BCSCs/well for further experiments. In the next experiments, all spheroids from this group were evaluated for their structures. PI staining assay showed that spheroids in vitro were like microtumors that contained two groups of cells one in the center and one in the outer layer of spheroids. Similar to in vivo tumors, the spheroid centers contained dead cells ( Figure 4 ).
Tumor marker expression on the spheroids was evaluated; these included E-cadherin, N-cadherin and Slug. BCSCs in spheroids reduced 0.215±0.146 fold of the expression of E-cadherin compared to BCSCs in 2D model. BCSCs increased the expression of N-cadherin and Slug (a regulatory factor in the epithelial-to-mesenchymal (EMT) process) more in the 3D model than in the 2D model (8.15±0.24 fold and 3.32±0.06 fold, respectively, for N-cadherin and Slug) ( Figure 5 ). BCSCs spheroids also demonstrated invasion of ECM in vitro. After 3 days of incubation of BCSC spheroids in the ECM gel, invadopodia development and invasion into the matrix were both observed ( Figure 6 ).
Effects of doxorubicin and Tirapazamine on spheroid proliferation
Before applying the 3D spheroid models of BCSCs in future experiments, the spheroids were used to evaluate the effects of the anti-tumor drugs, including Doxorubicin and Tirapazamine. The results showed that the IC50 value of Tirapazamine in the 2D model was significantly higher than that for the 3D model (14.45±1.365 μg/ml vs 1.709±0.4655 μg/ml, respectively, for 2D and 3D; p=0.0004). However, the IC50 values for doxorubicin in both culture conditions was not significantly different ( Figure 7 )
Dandelion extracts showed a strong ability to inhibit BCSC proliferation
We applied the 2D and 3D models, established in previous experiments, to evaluate the effects of dandelion extracts. The results showed that ethanoltreated and methanol-treated Dandelion extracts inhibited BCSC proliferation in a manner dependent on extract doses for both 2D and 3D models ( Figure 8 ).
In the 2D model, ethanol-treated and methanoltreated Dandelion extracts exhibited an antiproliferative effect on BCSCs; the IC50 values at 48 h were 59.22±0.5μg/ml and 14.88±0.03μg/ml, respectively. Moreover, the IC50 values of at 72 h were 92.30±2.83 μg/ml and 69.40±0.5 μg/ml, respectively. In the 3D model, both methanol- and ethanol-treated dandelion extracts yielded IC50 values that were much higher compared to those for the 2D model. In the 3D model, the IC50 value of the ethanol-treated extract at 48 h and 72 h were 1021±49.85 and 412±85.4 μg/ml, respectively; the IC50 values of the methanol-treated extract at 48 h and 72 h were 1012±5 and 142.2±17.45 μg/ml, respectively ( Figure 9 ). These results demonstrate that the IC50 value for BCSCs in 3D culture were much higher than in conventional 2D culture. The results also indicate that methanol-treated dandelion extract is more active than ethanol-treated extract, in both 2D and 3D culture systems.
After determining the IC50 values of the Dandelion extracts on BCSCs, cells were treated with the extract at the IC50 to evaluate induction of cell death. As shows in Figure 10 , the effect on cell death induction by Dandelion extracts differed between 2D and 3D models, as well as between the methanol and ethanol extracts. In monolayer cell culture (2D), ethanolinduced cell dead was greater than that for methanol; however, in 3D, the effect of methanol extract on cell death was greater.
Dandelion extracts induced the apoptosis via TRAIL
RT-PCR analysis of TRAIL, DR4, DR5 expression in BCSCs after treatment with dandelion extracts is shown in Figure 11 . The results showed that there were increased expression of TRAIL, DR4, and DR5 in treated BCSCs, for both ethanol and methanol extracts. Particularly, the extracts induced expression of DR4, which was not expressed in untreated cells. The relative quantity of the strength of the electrophoresis band, imaged by ImageJ software, also supported this observation. Indeed, compared to the control group, after treatment with ethanol and methanol dandelion extracts the expression of TRAIL increased 1.79 fold and 1.68 fold, respectively; expression of DR-4 increased 29.66 fold and 29.71 fold, respectively; lastly, expression of DR-5 increased 1.54 fold and 2.28 fold, respectively.
Dandelion extracts produced an increase of reactive oxygen species in BCSCs
To evaluate the effect of the dandelion extracts on ROS produced by BCSCs in 3D culture, a luminolbased chemiluminescence assay was performed 72h after treatment with IC50 doses of Dandelion extracts. As shown in Figure 12 , the luminescence value of the sample was markedly increased by 1.205 fold, compared with the untreated sample, following addition of the methanol-treated dandelion extract on BCSCs in 3D culture (p=0.0003). Addition of the ethanol-treated Dandelion extract showed no significant difference. The results suggest that methanol Dandelion extract treatment increases production of ROS in BSCSs.
Discussion
For screening anti-cancer drugs, many types of 3D cell culture models have been developed. For high throughput screening, 3D models need to focus mainly on methods that avoid adhesion of cells to the surface of culture equipment and promotion of cell-cell attachment Moore et al., 2001Robinson et al., 2004Schurr, 2006. Most systems are based on cellular aggregation on low-adherent plates, agarose-coated flatbottomed plates Schurr, 2006, or hanging drops Bayley and Devilee, 2012Vinci et al., 2012.
In this present study, a 3D model of BCSCs was established with low-attachment 96-well plates, called Nunclon Sphera plates, which provide a cell freescaffold form on which minimal cell attachment occurs with minimal extracellular matrix protein binding to the plate surfaces. Our results demonstrated that by using Nunclon Sphera, we were successful in establishing BCSCsin 3D culture. The cells which were cultured in this condition possessed the standard characteristics of microtumors in vivo.
The first tumor characteristic was related to the formation of a tumor spheroid core with a hypoxia environment and presence of dead cells. Many recent studies have shown that in most solid tumors, the center has a hypoxic environment, and that cancer cells at the center are mostly dead. Propidium iodide staining of GFP-BCSCs showed that spheroids also had a core of dead cells. These results demonstrate that there were the junctions between cells in the spheroids that caused oxygen and nutrients to enter the center. The hypoxia in the spheroids also confirms the antiproliferative nature of Tirapazamine, an anti-cancer chemical activated to a toxic radical only at very low levels of oxygen (hypoxia) Denny, 2004. Therefore, Tirapazamine should have biological effects on cancer cells in the hypoxic core. Indeed, the results showed that the IC50 of Tirapazamine in 3D was extremely lower than that for the 2D model of BCSCs. Potentially, the spheroids created a hypoxic environment to enable the Tirapazamine be active. Doxorubicin is a chemical not affected by hypoxia. In the doxorubicin assay, the IC50 value of doxorubicin was nearly the same in the 2D and 3D models. In some previous studies, hypoxia in spheroids was considered to be an important property of 3D cancer cell screening Imamura et al., 2015.
The second tumor characteristic relates to expression of markers related to the EMT process of tumors. The expression of E-cadherin was significantly reduced in spheroids (3D) compared to 2D. Loss or downregulation of E-cadherin in breast cancer is considered to be a marker for invasive lobular carcinoma Singhai et al., 2011, promoting cancer invasion and metastasis Onder et al., 2008. Moreover, N-cadherin and Slug were expressed much stronger in spheroids compared to 2D culture. Decrease of E-cadherin expression, and increase of N-cadherin and Slug (also called Snail) expression are clearly the phenotype of invasive ductal breast carcinoma tumor ElMoneim and Zaghloul, 2011. The expression of N-cadherin demonstrates that these tumors can strongly invade the ECM Jeschke et al., 2007Nieman et al., 1999. Additionally, the in vitro invasion assay confirms it; after 3 days of incubation in the ECM gel, the cells in the spheroids could form invadopodia and could migrated into the ECM gel.
The effects of the dandelion extracts were studied in both the 2D and 3D models. The experiments demonstrate that dandelion extracts could strongly inhibit the proliferation as well as induce apoptosis of BCSCs in both 2D and 3D cell culture models. However, the anti-proliferative effect of these extracts in 2D culture is stronger than in 3D. It is also observed that the BCSCs showed greater drug resistance capability in 3D. This result was similar to some previous publications about the chemosensitiyity of 3D and 2D cancer cell models Howes et al., 2014Lee et al., 2013. Lee et al., 2013 showed in many various cell lines that the transition from a 2D to 3D microenvironment likely induced changes in expression of several biomarkers relevant to cancer, proliferated more slowly, and were more chemoresistant (in 3D compared with 2D culture) Lee et al., 2013. In another model, (Howes et al., 2014) compared the IC50 of some anti-cancer chemicals in both 2D and 3D models of BT-474 breast cancer cells. They showed that for most chemicals, the IC50 values in the 3D model were higher than for the 2D model. In a 3D model of lung cancer, Godugu et al. (2013) showed that the IC50 of docetaxel on H460, A549 and H1650 were much higher than in the 2D models (1.41±0.29 vs 76.27±8.52 in H460 cancer cells, 1.94±0.35 vs 118.11±12.42 for A549 cancer cells, 2.70±0.66 vs 81.95±6.34 for H1650 Parental, and 14.53±1.24 vs 151.04±15.73 H1650 Stem; for 2D vs 3D, respectively).
Importantly, results in the 2D model revealed a new finding-that the IC50 values at 72h were higher than that for 48h. Commonly, in almost studies, the IC50 gradually decrease after treatment with anti-cancer chemicals for 24h, 48h, 72h, etc. However, in this study, the IC50 value was higher after 72 h compared to 48h in the 2D culture of BCSCs. That means that the drug resistance of BCSCs increased at 72 h, compared to 48h. This is a special property of cancer stem cells as well as BCSCs; after treatment with chemicals, initially almost all the cells would have died; however, the surviving cells become strongly resistance against the chemicals and thus grow rapidly.
Several previous studies have demonstrated that dandelion extracts can induce apoptosis in some kinds of cancers, including breast cancer, prostate cancer Sigstedt et al., 2008, hepatocarcinoma Yoon et al., 2016, and cervical cancer. In this study, dandelion extracts suppressed the growth of BCSCs. In the 2D model, BCSCs can be sensitive to this extract; however, in the 3D model, BCSCs can exhibit strong resistance to the extract. We also discovered that the antiproliferation effects of Dandelion extracts relates to expression of TRAIL and increased of ROS in cells.
Indeed, the potential of using TRAIL to destroy putative cancer stem cells has been recognized by others Chandrasekaran et al., 2014Yang et al., 2015. Increasing TRAIL expression has previously been shown to promote cancer cell death and to decrease tumor and metastasis development in vivo. Therefore, these results indicate that TRAIL-related apoptosis via death receptors is a mechanism of the dandelion extracts on BCSCs. Furthermore, the methanol-treated dandelion extract significantly increased ROS in BCSCs. Therefore, the induction of ROS in cancer stem cells has been approved to reduce the stability of cancer stem cells and reduce their drug resistance capability Diehn et al., 2009Kobayashi and Suda, 2012Trachootham et al., 2009.
Conclusion
In conclusion, in this study, we successfully developed a 3D culture of BCSCs for screening of natural products. BCSC-derived spheroids exhibited particular properties of microtumors, such as a hypoxic microenviroment at the center of spheroids, increased expression of mesenchymal markers (N-cadherin, Slug), and decreased expression of E-cadherin. Dandelion ethanol and methanol extracts effectively inhibited the growth of BCSCs in 2D. However, in the 3D culture, BCSCs were strongly resistant to these extracts. The inhibition of BCSC proliferation by the Dandelion extracts occurred through activation of TRAIL signaling and increased ROS production in BCSCs. Our findings suggest that dandelion plant extract is a suitable anti-cancer drug candidate, particularly for breast cancer.
References
-
S.
Abdelhamed,
S.
Yokoyama,
L.
Hafiyani,
S.K.
Kalauni,
Y.
Hayakawa,
S.
Awale,
I.
Saiki.
Identification of plant extracts sensitizing breast cancer cells to TRAIL. ncol Rep.
2013;
29
:
1991-1998
.
-
M.
Al-Hajj,
M.S.
Wicha,
A.
Benito-Hernandez,
S.J.
Morrison,
M.F.
Clarke.
Prospective identification of tumorigenic breast cancer cells. Proc Natl Acad Sci U S.
2003;
A100
:
3983-3988
.
-
B.M.
Baker,
C.S.
Chen.
Deconstructing the third dimension: how 3D culture microenvironments alter cellular cues. J Cell Sci.
2012;
125
:
3015-3024
.
-
J.P.
Bayley,
P.
Devilee.
The Warburg effect in 2012. Curr Opin Oncol.
2012;
24
:
62-67
.
-
S.
Chandrasekaran,
J.R.
Marshall,
J.A.
Messing,
J.W.
Hsu,
M.R.
King.
TRAIL-mediated apoptosis in breast cancer cells cultured as 3D spheroids. PLoS One.
2014;
9
:
e111487
.
-
S.J.
Chatterjee,
P.
Ovadje,
M.
Mousa,
C.
Hamm,
S.
Pandey.
The efficacy of dandelion root extract in inducing apoptosis in drug-resistant human melanoma cells. vid Based Complement Alternat Med.
2011;
2011
:
129045
.
-
A.M.
Chinnaiyan,
U.
Prasad,
S.
Shankar,
D.A.
Hamstra,
M.
Shanaiah,
T.L.
Chenevert,
B.D.
Ross,
A.
Rehemtulla.
Combined effect of tumor necrosis factor-related apoptosis-inducing ligand and ionizing radiation in breast cancer therapy. Proc Natl Acad Sci U S A.
2000;
97
:
1754-1759
.
-
M.F.
Clarke,
J.E.
Dick,
P.B.
Dirks,
C.J.
Eaves,
C.H.
Jamieson,
D.L.
Jones,
J.
Visvader,
I.L.
Weissman,
G.M.
Wahl.
Cancer stem cells perspectives on current status and future directions: AACR Workshop on cancer stem cells. Cancer Res.
2006;
66
:
9339-9344
.
-
S.
Coseri.
Natural products and their analogues as efficient anticancer drugs. Mini Rev Med Chem.
2009;
9
:
560-571
.
-
A.K.
Croker,
A.L.
Allan.
Cancer stem cells: implications for the progression and treatment of metastatic disease. J Cell Mol Med.
2008;
12
:
374-390
.
-
W.A.
Denny.
Prospects for hypoxia-activated anticancer drugs. Curr Med Chem Anticancer Agents.
2004;
4
:
395-399
.
-
M.
Diehn,
R.W.
Cho,
N.A.
Lobo,
T.
Kalisky,
M.J.
Dorie,
A.N.
Kulp,
D.
Qian,
J.S.
Lam,
L.E.
Ailles,
M.
Wong.
Association of reactive oxygen species levels and radioresistance in cancer stem cells. Nature.
2009;
458
:
780-783
.
-
A.M.
Edwards,
C.H.
Arrowsmith,
C.
Bountra,
M.E.
Bunnage,
M.
Feldmann,
J.C.
Knight,
D.D.
Patel,
P.
Prinos,
M.D.
Taylor,
M.
Sundstrom.
Preclinical target validation using patient-derived cells. Nat Rev Drug Discov.
2015;
14
:
149-150
.
-
H.M.A.
ElMoneim,
N.M.
Zaghloul.
Expression of ecadherin, n-cadherin and snail and their correlation with clinicopathological variants: an immunohistochemical study of 132 invasive ductal breast carcinomas in Egypt. Clinics.
2011;
66
:
1765-1771
.
-
A.L.
Howes,
R.D.
Richardson,
D.
Finlay,
K.
Vuori.
3-Dimensional Culture Systems for Anti-Cancer Compound Profiling and High-Throughput Screening Reveal Increases in EGFR Inhibitor-Mediated Cytotoxicity Compared to Monolayer Culture Systems. PLoS ONE.
2014;
9
:
e108283
.
-
Y.
Imamura,
T.
Mukohara,
Y.
Shimono,
Y.
Funakoshi,
N.
Chayahara,
M.
Toyoda,
N.
Kiyota,
S.
Takao,
S.
Kono,
T.
Nakatsura.
Comparison of 2D- and 3D-culture models as drug-testing platforms in breast cancer. Oncol Rep.
2015;
33
:
1837-1843
.
-
W.
International.
Breast cancer statistics. 2012
.
-
S.
Jaeger,
M.
Duran-Frigola,
P.
Aloy.
Drug sensitivity in cancer cell lines is not tissue-specific. Molecular Cancer.
2015;
14
:
40
.
-
U.
Jeschke,
I.
Mylonas,
C.
Kuhn,
N.
Shabani,
C.
Kunert-Keil,
C.
Schindlbeck,
B.
Gerber,
K.
Friese.
Expression of E-cadherin in human ductal breast cancer carcinoma in situ, invasive carcinomas, their lymph node metastases, their distant metastases, carcinomas with recurrence and in recurrence. Anticancer Res.
2007;
27
:
1969-1974
.
-
M.M.
Keane,
S.A.
Ettenberg,
M.M.
Nau,
E.K.
Russell,
S.
Lipkowitz.
Chemotherapy augments TRAIL-induced apoptosis in breast cell lines. Cancer Res.
1999;
59
:
734-741
.
-
S.
Ketut Edy Sudiarta,
Riawan
Wibi,
Gede Muliartha
Ketut,
Mintaroem
Karyono,
Aulanni’am
Aulanni’am,
Ali
Mulyohadi.
The Efficacy of Taraxacum officinale Leaves Extract in Regulate Apoptosis, RARß2 gene and Sox2 expression on Primary Culture Human Cervical Cancer Stem Cells. International Journal of PharmTech Research.
20165;
8
.
-
L.C.
Kimlin,
G.
Casagrande,
V.M.
Virador.
In vitro three-dimensional (3D) models in cancer research: an update. Mol Carcinog.
2013;
52
:
167-182
.
-
C.I.
Kobayashi,
T.
Suda.
Regulation of reactive oxygen species in stem cells and cancer stem cells. J Cell Physiol.
2012;
227
:
421-430
.
-
J.M.
Lee,
P.
Mhawech-Fauceglia,
N.
Lee,
L.C.
Parsanian,
Y.G.
Lin,
S.A.
Gayther,
K.
Lawrenson.
A threedimensional microenvironment alters protein expression and chemosensitivity of epithelial ovarian cancer cells in vitro. Lab Invest.
2013;
93
:
528-542
.
-
J.
Monteiro,
R.
Fodde.
Cancer stemness and metastasis: therapeutic consequences and perspectives. Eur J Cancer.
2010;
46
:
1198-1203
.
-
P.S.
Moore,
B.
Sipos,
S.
Orlandini,
C.
Sorio,
F.X.
Real,
N.R.
Lemoine,
T.
Gress,
C.
Bassi,
G.
Kloppel,
H.
Kalthoff.
Genetic profile of 22 pancreatic carcinoma cell lines. Analysis of K-ras, p53, p16 and DPC4/Smad4. Virchows Arch.
2001;
439
:
798-802
.
-
M.T.
Nieman,
R.S.
Prudoff,
K.R.
Johnson,
M.J.
Wheelock.
N-Cadherin Promotes Motility in Human Breast Cancer Cells Regardless of Their E-Cadherin Expression. The Journal of Cell Biology.
1999;
147
:
631-644
.
-
T.T.
Onder,
P.B.
Gupta,
S.A.
Mani,
J.
Yang,
E.S.
Lander,
R.A.
Weinberg.
Loss of E-cadherin promotes metastasis via multiple downstream transcriptional pathways. Cancer Res.
2008;
68
:
3645-3654
.
-
W.H.
Organization.
All Cancers (excluding nonmelanoma skin cancer) Estimated Incidence, Mortality and Prevalence Worldwide in 2012. 2012
.
-
C.M.
Perou.
Molecular stratification of triple-negative breast cancers. Oncologist15 Suppl.
2010;
5
:
39-48
.
-
E.E.
Robinson,
R.A.
Foty,
S.A.
Corbett.
Fibronectin matrix assembly regulates alpha5beta1-mediated cell cohesion. Mol Biol Cell.
2004;
15
:
973-981
.
-
K.
Sampieri,
R.
Fodde.
Cancer stem cells and metastasis. Seminars in Cancer Biology.
2012;
22
:
187-193
.
-
F.
Sams-Dodd.
Target-based drug discovery: is something wrong?. Drug Discov Today.
2005;
10
:
139-147
.
-
A.
Schurr.
Lactate: the ultimate cerebral oxidative energy substrate?. J Cereb Blood Flow Metab.
2006;
26
:
142-152
.
-
S.C.
Sigstedt,
C.J.
Hooten,
M.C.
Callewaert,
A.R.
Jenkins,
A.E.
Romero,
M.J.
Pullin,
A.
Kornienko,
T.K.
Lowrey,
S.V.
Slambrouck,
W.F.
Steelant.
Evaluation of aqueous extracts of Taraxacum officinale on growth and invasion of breast and prostate cancer cells. International journal of oncology.
2008;
32
:
1085-1090
.
-
R.
Singhai,
V.W.
Patil,
S.R.
Jaiswal,
S.D.
Patil,
M.B.
Tayade,
A.V.
Patil.
E-Cadherin as a diagnostic biomarker in breast cancer. North American Journal of Medical Sciences.
2011;
3
:
227-233
.
-
S.S.
Stylli,
A.H.
Kaye,
P.
Lock.
Invadopodia: at the cutting edge of tumour invasion. J Clin Neurosci.
2008;
15
:
725-737
.
-
D.
Trachootham,
J.
Alexandre,
P.
Huang.
Targeting cancer cells by ROS-mediated mechanisms: a radical therapeutic approach?. Nat Rev Drug Discov.
2009;
8
:
579-591
.
-
P.
Van Pham,
N.B.
Vu,
T.T.
Duong,
T.T.
Nguyen,
N.H.
Truong,
N.
Phan,
T.G.
Vuong,
V.Q.
Pham,
H.M.
Nguyen,
K.T.
Nguyen.
Suppression of human breast tumors in NOD/SCID mice by CD44 shRNA gene therapy combined with doxorubicin treatment. Onco Targets Ther.
2012;
5
:
77-84
.
-
P.
Van Phuc,
T.T.T.
Khuong,
T.D.
Kiet,
T.T.
Giang,
P.K.
Ngoc.
Isolation and characterization of breast cancer stem cells from malignant tumours in Vietnamese women. Journal of Cell and Animal Biology.
2010;
4
:
163-169
.
-
M.
Vinci,
S.
Gowan,
F.
Boxall,
L.
Patterson,
M.
Zimmermann,
W.
Court,
C.
Lomas,
M.
Mendiola,
D.
Hardisson,
S.A.
Eccles.
Advances in establishment and analysis of three-dimensional tumor spheroid-based functional assays for target validation and drug evaluation. BMC Biology.
2012;
10
:
1-21
.
-
H.
Walczak,
R.E.
Miller,
K.
Ariail,
B.
Gliniak,
T.S.
Griffith,
M.
Kubin,
W.
Chin,
J.
Jones,
A.
Woodward,
T.
Le.
Tumoricidal activity of tumor necrosis factor-related apoptosis-inducing ligand in vivo. Nat Med.
1999;
5
:
157-163
.
-
M.
Wartenberg,
F.C.
Ling,
M.
Muschen,
F.
Klein,
H.
Acker,
M.
Gassmann,
K.
Petrat,
V.
Putz,
J.
Hescheler,
H.
Sauer.
Regulation of the multidrug resistance transporter Pglycoprotein in multicellular tumor spheroids by hypoxiainducible factor (HIF-1) and reactive oxygen species. Faseb j.
2003;
17
:
503-505
.
-
J.L.
Wilding,
W.F.
Bodmer.
Cancer cell lines for drug discovery and development. Cancer Res.
2014;
74
:
2377-2384
.
-
K.M.
Yamada,
E.
Cukierman.
Modeling tissue morphogenesis and cancer in 3D. Cell.
2007;
130
:
601-610
.
-
Y.
Yang,
H.
Xu,
W.
Huang,
M.
Ding,
J.
Xiao,
D.
Yang,
H.
Li,
X.-Y.
Liu,
L.
Chu.
Targeting lung cancer stemlike cells with TRAIL gene armed oncolytic adenovirus. ournal of Cellular and Molecular Medicine.
2015;
19
:
915-923
.
-
J.Y.
Yoon,
H.S.
Cho,
J.J.
Lee,
H.J.
Lee,
S.Y.
Jun,
J.H.
Lee,
H.H.
Song,
S.
Choi,
V.
Saloura,
C.G.
Park.
Novel TRAIL sensitizer Taraxacum officinale F.H. Wigg enhances TRAIL-induced apoptosis in Huh7 cells. Mol Carcinog.
2016;
55
:
387-396
.
-
M.
Zanoni,
F.
Piccinini,
C.
Arienti,
A.
Zamagni,
S.
Santi,
R.
Polico,
A.
Bevilacqua,
A.
Tesei.
3D tumor spheroid models for in vitro therapeutic screening: a systematic approach to enhance the biological relevance of data obtained. Sci Rep.
2016;
6
:
19103
.
Comments
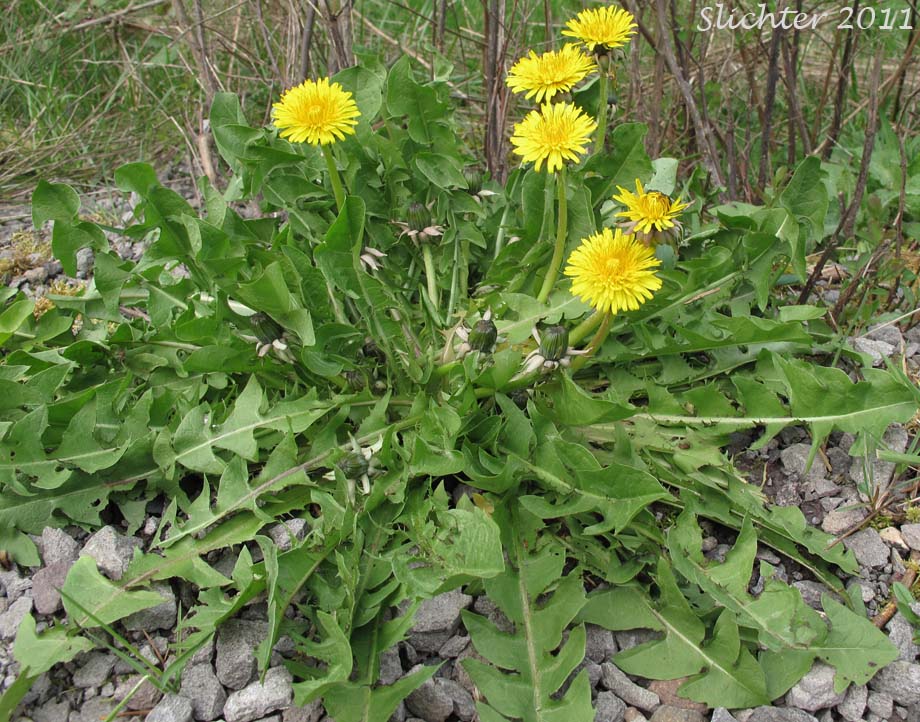
Downloads
Article Details
Volume & Issue : Vol 3 No 07 (2016)
Page No.: 733-741
Published on: 2016-07-30
Citations
Copyrights & License

This work is licensed under a Creative Commons Attribution 4.0 International License.
Search Panel
Pubmed
Google Scholar
Pubmed
Google Scholar
Pubmed
Google Scholar
Pubmed
Search for this article in:
Google Scholar
Researchgate
- HTML viewed - 16078 times
- Download PDF downloaded - 2402 times
- View Article downloaded - 10 times