Abstract
Lead poisoning causes numerous clinical implications in almost all organs, with the brain, liver, and kidneys serving as the primary targets due to the abundant presence of mitochondria. Curcumin is one of the most potent constituents of Curcuma longa, which is lipophilic, phenolic and water insoluble. Curcumin is a strong antioxidant and anti-inflammatory agent in the treatments of neurodegenerative disease, cardiovascular, renal, and liver diseases, with a potential anticancer mechanism in a few clinical and experimental trials. This review will focus on the health impact of lead-induced toxicity in different organ-systems, which occurs as result of increased oxidative stress through the generation of reactive oxygen species (ROS) and reactive nitrogen species (RNS) and will discuss the therapeutic potential of curcumin against lead-induced toxicity in both human and animals.
Introduction
Lead toxicity is a common public health threat in developing countries due to human activities such as mining and farming 1. Lead is a multi-organ toxicant involved in various cancers, neuronal and renal damages and reproductive impairments in both human and animals, which can eventually causes death in young children 1,2. Although several occupational and public health safety measures have been carried out to reduce the cases of lead exposure to the minimal level, yet, several cases of lead poisoning are still recorded.
The application of standard drugs such as chelators in the treatment and management of heavy metal poisoning have been documented to show numerous side effects ranging from mild to severe levels, which may include fever, headache, nausea and vomiting, seizures, brain damage, anemia, permanent kidney and liver diseases, low blood pressure, and severe allergic reactions such as anaphylactic shock. Only a few local herbs extracts have been shown to provide positive effects against lead mediated injury both in in vitro and in vivo studies 1.
Curcumin has anti-inflammatory and antioxidant properties with a wide range of therapeutic potentials both in vitro and in vivo 3, which makes it suitable in reversing the biomarkers and alterations induced by lead toxicity in several organs due to induction of oxidative stress (OS) through generation of reactive oxygen species (ROS) and stimulation of inflammatory response 4,5. This review will focus on the therapeutic potential of curcumin against lead-induced toxicity in both human and animals.
Epidemiology of Lead Toxicity
According to World Health Organization (WHO) survey about 0.6% of global heath threatening diseases and 600,000 sources of intellectual impairment among children are attributed by lead poisoning 6,7. WHO and United State Center for Disease Control and Prevention (CDC) has indicated that a critical level of 10-15µg/dL of lead in the blood can be considered as an elevated blood lead level 8,9.
Blood lead levels in the pediatric population have decline dramatically since 1970s as a result of public health policies such as removal of lead in paints, gasoline, and other consumer products 10. However, based on National Health and Nutrition Examination (NHANES) survey from 2007 to 2010, children between the age of 1 to 5 years with blood lead levels (BLLs) exceeding 5µg/dL accounted for approximately 2.6% with 535000 children 11. In addition, socioeconomic status plays an important role in mean blood lead levels (BLLs) in younger children, however, the greater risk is attributed to non-Hispanic black population 10,11,12.
Occupational exposure to lead is associated with numerous health implications such as, cancer and mortality. Even at a low concentration in adults, lead can results in different adverse health challenges, which may include cognitive impairments, reproductive effects and hypertension 13,14.
According to the United State Occupational Safety and Health Administration (OSHA) report, an estimated population of 804,000 within the general industry workers and 838,000 among construction industry workers are prone to lead exposure due to the nature of their occupations. Data from the Adults Blood Lead Epidemiology and Surveillance (ABLES) program revealed a significant reduction in elevated blood lead levels (BLLs) prevalence among adults, thus occupational exposure remains a public health concern with approximately 94% of industrial workers exposed to lead 2.
Lead (Pb) is a naturally occurring soft, moldable, and blue-gray heavy metal with relatively low melting point which is found in combination with other elements in our environment. It is considered as the major pollutant of the environment due to its popular use in product manufacture (Figure 1) such as paints, gasoline, batteries, cosmetic products, water pipes, tank linking, poetry glazing and toys 7,15. In addition, exposure to lead in the environment can caused damage to several biological systems, which can occur from several sources such as air, water, food and other consumer products 10. Ingestion and inhalation of lead particles are the primary modes of exposure in the environment, while skin and prenatal exposure has been reported in few cases 10,16.
Nevertheless, lead exists in two (2) different forms, organic and inorganic lead 16. Organic lead (tetraethyl and tetra methyl lead) is used as a fuel additive to increase octane rating in the past. However, organic lead exposure is considered as an occupational exposure and is tremendously hazardous because it can penetrates the skin before being absorbed by the body, which results in extreme toxicity to the central nervous system when compared to inorganic lead 7. In contrast, inorganic lead is found in consumer products such as paint and toys, and other environmental content such as soil, and dust 7,17.
Lead and other heavy metals are found in combination with other element in a relatively low pollutant concentrations in all parts of the environment, hence, the presence of lead in the environment has no role to play in the physiological system and this can lead to an irreversible health effects with a lot of morbidity such as hepatic, nervous and renal system disorder 18,19. Moreover, due to human activities such as farming and mining concentration of these heavy metals have increased drastically in the environment, particularly in areas where the metals are mined and processed for industrial use 16,20,21.
Pathophysiology of Lead Toxicity
Absorption of Lead
Gastrointestinal and inhalational absorption of lead is the most common route of absorption of lead particles. However, dermal absorption is a form of absorption route for organic lead although it is considered insignificant, and only account for less than 1% of lead absorbed in the body system 22.
Papa Nikolaou et al. 23 reported that gastrointestinal absorption rate of lead is correlated to socio-demography of the exposed individual. In addition, children absorbed almost 50% of lead compared with 15% in adults due to their pica behavior (Figure 2). However, when lead is absorbed it accumulates in blood, soft tissues, and bones 1,24,25.
Nevertheless, approximately 100% of fine particles of lead inhaled as fume or vapor may be absorbed by the lungs directly or transported by the mucociliary tree to the esophagus where it may be swallowed and absorbed through the gastrointestinal tract. The absorption of lead depends on the particular nature of the toxicant, volume of the respiratory tract and mucociliary clearance of the toxicant 22.
Distribution of Lead
Lead concentration in the plasma is the most important means of distribution of lead to target organs such as the brain, kidney, liver, spleen, bones, aorta and teeth, which accounts for only 1% of the total lead concentration in the blood, leaving 99% concentration in the erythrocytes 1,10,23,26. Furthermore, lead distribution to the entire body depends on the systemic blood flow and soluble phosphate, which harbors more than 95% of lead deposited into the skeletal bones (Figure 2) 7,12,27. Nevertheless, bone contaminated with lead in adult accounts for about 80-95% of the total health burden, which is higher than in children with about 70-73% 12.
In addition, an estimated half-life of lead in adults blood is 30-40 days and is longer in children and pregnant women 10,12,25. Moreover, lead in the liver interfere with the cytochrome P450 enzymes, which affects hormone synthesis, and cholesterol synthesis 10. In adults and children about 94%-70% of lead is accumulated in the bones where it is tightly bounded and is less harmful 25.
Excretion
Lead absorbed in the body are not easily metabolized, therefore the excretion is low and the excretion route is mostly through the urinary tract. In addition, chelating agent can facilitate the excretion of lead from the body via urine 12,23. Little amounts of lead are excreted through the gastrointestinal tract, sweat and nails, thus these routes are considered as insignificant 12.
General Symptoms of Lead Toxicity
Clinical symptoms of lead toxicity are asymptomatic or nonspecific and are dependents on the duration and levels of exposure. However, the symptoms can be classified into two categories, which could be either acute or chronic clinical symptoms 1,7,9,28. The symptoms of acute toxicity includes muscle pain, fatigue, abdominal pain, headache, vomiting, seizures and coma. Clinical symptoms of chronic toxicity includes persistent vomiting, encephalopathy, lethargy, delirium, convulsions and coma 1,28,29.
Mechanism and Toxicological Profile of Lead-induce Toxicity
Pathogenesis of lead is due to its ability to bind to proteins group sulfhydryl, resulting to toxicity of multiple enzyme systems. Calcium-activated proteins binds to lead in a greater affinity than to calcium, hence hindering cellular physiology 10,25,30. Upturn in metabolic rate results in over-production of free radicals, which in turn leads to oxidative stress damage such as lipid peroxidation, damage to proteins and nucleic acids and distortion of normal physiology in tissues 31. Oxidative stress is a major biomarker in the development of several disorders in disease manifestation such as diabetes, chronic kidney disease, hepatic inflammation, chronic cardiac disease, neurodegenerative diseases and others 32.
However, at the molecular level it is difficult to limit lead toxicity on cellular damage 33. The enzymatic functions of proteins and other cellular activities are affected by the presence of lead in the system 30. In addition, the induction of oxidative stress and damage of nucleic acids are other mechanisms of lead toxicity and exposure, which can result in several clinical symptoms of toxicity. Hence, the magnitude of exposure and cell types depends on the mechanism of toxicity and the levels of lead exposure (Figure 3) 33,34,35.
Oxidative Stress
Increased levels of reactive oxygen species (ROS) are the major toxic effects of lead 1. Reactive oxygen species are by-products of biochemical processes in aerobic organisms and ROS concentration is regulated by the activity of antioxidant enzymes such as glutathione (GSH), super oxide dismutase (SOD), glutathione peroxidase (GPx), and catalase (CAT) under normal conditions 12. The imbalance between the production and scavenging of ROS results to oxidative stress, which could lead to ROS detoxification system impairment and increased production of ROS 1,33,34. However, during oxidative stress, overproduction of free radicals results in negative effects on cells, tissues, inflammatory responses, and apoptosis 1,12,33. Neurodegenerative diseases such as Alzheimer’s and Parkinson’s disease are the primary results of increased production of ROS in the body system 36.
Glutathione (GSH) is a tripeptide with a sulfhydryl group and is the most important antioxidant found in mammalian cells in millimolar concentrations. GSH is vital in the scavenging of free radicals and can exist in either reduced (GSH) or oxidized (GSSG) forms. However, 90% of glutathione under normal circumstances are in a reduced form (GSH). In abnormal condition of oxidative stress, glutathione concentration in oxidized form (GSSG) is in abundant compared to GSH 1.
Super oxide dismutase (SOD) and catalase (CAT) are notable antioxidant enzymes that are essential in rendering lead inactivation in the system. However, the impairment in superoxide radicals (O2-) scavenging is a direct consequence of reduced CAT concentration in the serum decrease SOD concentration, which results in reduced disposal of superoxide radicals 1. In addition, lead has the ability to replace zinc ions which are an important factors in deactivating the antioxidant defense mechanism beside targeting sulfhydryl groups 1,37.
Another important oxidative stress biomarker associated with lead toxicity is lipid peroxidation, which accounts for increased reactive oxygen species (ROS) on lipid membrane, thus damaging the cells 1.
Oxidation of hemoglobin is attributed to lead toxicity, leading to red blood cell (RBC) destruction through the inhibition of Delta-aminolevulinic acid dehydrates (ALAD) and increased substrate alpha-Linolenic acid (ALA) concentrations in urine and blood. Furthermore, increased in ALA levels produce superoxide and peroxide radical that interfere with oxyhemoglobin consequently leading to hydroxyl radicals generation, which in turn put the cells at risk of oxidative stress and apoptosis 38.
Ionic Mechanism
The ability of lead as a divalent cation to substitute bivalent (Ca2+, Mg2+, Fe2+) and monovalent (Na+) cations results in the impairment of several important biological processes in the system. This ability to substitute both bivalent and monovalent cations, especially the bivalent cations is termed the ionic mechanism of lead toxicity 1,7,39.
Neurological deficits are associated with the ionic mechanism of lead toxicity due to the ability of lead to replace calcium ions 12. Therefore, lead molecules become competent and subsequently cross the blood brain barrier (BBB) at a significant rate, where they accumulate in the astroglial cells. Moreover, developing nervous system contain numerous immature astroglial cells, which become more prone to toxic effects of lead due to the lack of lead binding proteins in the immature astroglial cells 12,40.
The concentration of lead even in a pico molar, has the ability to replace calcium, and affect vital neurotransmitters such as protein kinase C, which regulates memory storage and neural excitation. In addition, sodium ions concentration is also affected by lead toxicity resulting in several neuronal signaling impairments due to misbalancing of neurotransmitters 1.
Other deleterious effects of ionic mechanism of lead-induced toxicity on different fundamental biological cellular processes include, cell adhesion, protein folding and maturation, intra and inter cellular signaling, neurotransmitters release, enzyme regulation, ionic transportation, and apoptosis 1,40.
Effects of Lead Toxicity
Nervous System
Lead-induced neurotoxicity mechanisms are composite and include membrane bio-physics alteration, deregulation of cell signaling, oxidative stress and impairment of neurotransmitter transmission. Furthermore, lead absorption rate through the gastrointestinal tract relies on the physicochemical and individual characteristics of the materials ingested 12.
Lead toxicity interferes with glial cells interaction, trimming and pruning of synapses, and neuronal migration during brain development, which lead to failure in creating a proper link between different brain structures and subsequently resulting to permanent alteration of brain physiology 15,41.
Lead toxicity is multi-systemic with high affinity and severity on the central nervous system (CNS) by blocking N-methyl-D aspartate receptors that aid in the maturation of brain, flexibility, and memory storage. This result to the interruption in long-term storage of memory and other cognitive behaviors. In addition, elevated blood lead levels is pivotal in disrupting the integrity of the blood-brain barrier 30.
Exposure to high levels of lead concentration in the blood-brain barrier results in the movement of plasma into the brain interstitial space, subsequently leading to encephalopathy of the brain and edema of the cerebellum 30. At the blood lead levels of (BLLs) 10 µg/dL, extreme increase in pressure is caused by edema in the brain, which results in irreversible brain destruction and alteration including impaired visual-motor skill, reduced attention, decreased social behavior and decline in cognitive ability 30,41.
Renal System
Kidney remains a vital organ of target upon cumulative exposure to lead both environmentally or occupationally, which may cause acute or chronic nephropathy with several health implications such as deficit in tubular transport mechanism (tubular absorption and reabsorption), degenerative changes in tubular epithelium, renal dysfunction, renal failure, hypertension, and hyperuricemia 42. In comparison, acute Pb nephropathy effects are reversible in children manifesting glycosuria and aminoaciduria by using chelation therapy, while chronic Pb nephropathy is irreversible that develops over a long period of time due to prolonged exposure 43,44.
The results obtained by Mao et al., 45, suggested that lead selenide nanoparticles induction of oxidative damage in Sprague-Dawley rats showed histopathological changes in kidney such as, foci of ischemia and clogged blood vessels, interstitial fibrosis, raise in number of fibroblasts and severe renal parenchymal destruction.
Bones
Bones remain the primary site for storage of lead in the human body after exposure 46. Once lead is absorbed, it moves into the bloodstream where it is primarily circulated to the soft tissues through plasma (kidney, liver, and brain), blood and mineralized tissues such as the bones. Then it exerts more toxic effects by binding to the cell membrane, damaging protein architectural structure, and meddling with gene interpretation in the body 18,47.
Lead deposited in the bone have an estimated half-life of 20-30 years. However, bone metabolism and activities results in the discharge of lead back into the bloodstream, thus, blood lead level (BLLs) is increased, which in turn have detrimental effects in pregnancy, lactation, and menopause 41.
Moreover, methodology such as stable lead isotope revealed that an estimated 40-70% of lead is released from the blood to bones in adults, hence, adults bones store 85-95% of lead but children only store 70% of lead in the bones and greater absorption of lead is in the soft tissues 1. The rate of exposure, age, pregnancy, race and gestation are factors that affect storage and mobilization of lead in bones 1.
Reproductive System
Exposure to lead have numerous effects on the reproductive system both in human and animals which includes reduced libido, abnormal spermatogensis, infertility, changes in serum testosterone, abnormal prostatic function, miscarriage, pre-eclampsia, premature delivery, and premature membrane rupture in both males and females 1.
Previous studies indicated that lead could cause peritubular testicular fibrosis, reduced sperm number, disrupted regulation of luteinizing hormone, lower testosterone synthesis, distraction of preantral follicles and increased atresia in ovaries, and reduced number of primordial follicles in female pups in rodents 48,49.
Cardiovascular System
Lead exposure associated with cardiovascular effects is not restricted to amplified blood pressure and hypertension but is rather associated with other deleterious cardiovascular clinical burdens such as stroke, peripheral arterial diseases (arteriosclerosis and atherosclerosis), and coronary heart diseases which have underlying cardiovascular malfunctioning and abnormalities such as alterations in cardiac rhythmicity and left ventricular hypertrophy 49.
Hematological Parameters
The total body burden of blood lead represents merely 1-5%, however, 95-99% concentration of blood lead at lower concentrations binds to red blood cells (RBCs) with an estimated 1% in the plasma in an ionized form. Lead binding sites in the red blood cells (RBCs) can become saturated at higher concentrations, resulting in more lead presence in the plasma 47.
Destruction of Red Blood Cells (RBCs), and alteration in heme synthesis is associated with lead toxicity, however, hemoglobin production decreases when heme biosynthesis is altered due to lead toxicity at the blood lead levels (BLLs) of 40 1g/dL 10,50. Moreover, lead toxicity inhibits heme synthesis by impeding the actions of δ-aminolevulinic acid dehydratase (ALAD), ferrochelatase, aminolevulinic acid synthatase (ALAS), aminolevulinic acid (ALA) and other mitochondria enzymes, which reduce the life span of erythrocytes in circulation due to persistent instability of the cell membrane, and lead to anemia 1,51.
Moreover, hemolytic (acute exposure) and Frank (chronic exposure) anemia are the direct consequence of lead poisoning 1.
Lead toxicity causes anemia in young and iron deficient children, which is correlated with blood lead concentration (BLLs). In addition, lead toxicity results in clinical alteration of heme-biosynthesis and increased red blood cell destruction 7,51.
Alwaleedi 51 induced lead toxicity in white wool albino mice using different concentrations, of 0.4, 0.8, and 1.2mg/kg orally within 12 weeks which resulted in hematological and tissue morphological alteration. Importantly, lead toxicity has several effects on the biological system and it is considered as the major cause of physiological, biochemical, hematological, and morphological alterations. Even at low concentrations, treatments with lead acetate have an adverse effect on health in animals. Therefore, it is important to prevent exposure to lead in the environment 52.
Spleen
The work of Ekanem et al. 18 showed an evidence of lymphoid follicles hyperplasia within the white pulp with congested blood vessels and alteration in hematological profiles within the spleen which occurred upon lead-induced toxicity in Albino rats treated with different concentrations of lead acetate.
The works of Türkay et al. 51 revealed ultra-structural alterations on spleen with macrophage and lymphocyte infiltration, vacuolation of cytoplasm, erythrocyte stasis, swollen mitochondria with cristae loss in lymphoid cells, and degeneration of spleen cells.
Curcumin and its curative potential mechanism
Curcumin (1,7-bis[4-hydroxy-3-methoxyphenyl]-1,6-heptadiene-3,5-dione) is a yellow color compound that is lipophilic, phenolic, water insoluble and is a derivative of the rhizomes of turmeric (curcuma longa). Curcumin is a member of the ginger family (Zingiberaceae) that is widely considered as a traditional medicine and food additive in Asia (Figure 4) 53,54,55. Curcumin is the most biologically active constituent of Curcuma longa and it is readily available commercially as a mixture of 3 curcuminoids (95% curcumin). However, this mixture of the 3 curcuminoids classically contains 77% curcumin, 17% demethoxycurcumin, and bisdemethoxycurcumin 6% responsible for the yellow color characteristics of turmeric 54,56.
Structurally, curcumin can exists in either β-diketone (CurK) or β-keto-enol (CurE) tautomers, with the higher antioxidant activity and stability in β-keto-enol tautomers (CurE) when compared to the β-diketone (CurK) tautomers. In addition, β-keto-enol tautomer has triple chelation sites for metals, which includes a double phenol group that serves as a possible reactive centers, and the keto-enolic moieties, which can possibly form metal chelates and scavengers for reactive radicals generated by metal ions 57. Curcumin has a molecular weight of 368.37g/moL and a melting point of 183oC. Available literatures have shown that curcumin is more stable in cell culture medium or human blood and is unstable at basic pH medium 58.
In respect to curcumin side effects, approved bodies such as European Food Safety Authority (EFSA), US Food and Drug Administration (FDA), United Nation and World Health Organization Expert Committee on Food Additives reported, that curcumin is Generally Recognized As Safe (GRAS), with the tolerable and safety doses between 4000 and 8000 mg/day 59.
In concurrence with several literatures, curcumin has been proven to have a wide range of therapeutic effect which include, antioxidant, antibacterial, antifungal, antiviral, antitumor, cancer chemo-preventive, antimalarial, anti-inflammatory and hepatoprotective abilities, and the ability to improve cardiovascular and neurodegenerative disorders 57,60,61,62. In addition, curcumin has the ability to easily cross the blood-brain barrier where it binds to plaques in the brain to inhibit amyloid-β peptide aggregation in patient with Alzheimer’s disease 60,63,64.
Despite the therapeutic benefits of curcumin, the major challenge is attributed to its poor bioavailability due to poor absorption, fast metabolism, and rapid elimination from the body by the gastrointestinal tract 65. Curcumin bioavailability can be enhanced through several agents such as piperine which is an active component of black pepper and its mechanism of action which includes the blocking of the metabolic pathway in order to delay the metabolism of curcumin 5. In addition, about 2000% increase in bioavailability of curcumin is associated with the primary active component of black pepper, piperine 5,66.
Antioxidant Mechanism
Curcumin alleviates systematic markers of oxidative stress by increasing the activities of serum anti-oxidants enzymes such as superoxide dismutase (SOD), glutathione peroxidase (GSH) and lipid peroxides. Moreover, curcumin antioxidant mechanism is executed in different patterns, which may include free radicals scavenging of reactive nitrogen species (RNS) and reactive oxygen species (ROS) as well as increasing the activity of serum enzymes such as GSH, catalase, and SOD that could result in the activation of inflammatory responses 5 (Figure 5).
Moreover, curcumin phenolic antioxidants activity is mainly dependent on electron donation, and although curcumin is hydrophobic, but can be liquefied in ethanol, acetone, and dimethylsulphoxide 67.
Noteworthy, curcumin, as a lipophilic compound, acts similarly vitamin E to efficiently scavenge peroxyl radicals and is referred to as a chain breaking antioxidant. Furthermore, curcumin inhibits the activity of ROS-generating enzymes such as xanthine hydrogenase/oxidase and lipoxygenase/cyclooxygenase, which is essential in the down regulation of oxidative stress 5,68.
Anti-inflammatory Mechanism
Previous research has shown that curcumin as a polyphenolic compound, interact with several targets that are involved is inflammation. Curcumin moderates inflammatory response by suppressing the activity of cyclooxygenase-2 (COX-2), inducible nitric oxide synthase (iNOS) and lipoxygenase enzymes as well as inhibiting the production of interleukin 1,2,6,8 and 12, inflammatory cytokines tumor necrosis factor-alpha (TNF-α), monocytes chemoattractant protein (MCP) and the down regulation of Janus and mitogen-activated kinase 3,69,70.
In vivo studies on inflammatory models showed that curcumin inhibits the metabolism of arachidonic acid and inflammation in the epidermis through the down regulation of lipoxygenase and cyclooxygenase pathways and the reduction in PGE2 inflammatory exudate 71,72.
In vitro studies revealed that curcumin modulates the stimulation of specific transcription factors such as NF-kB and activating protein-1 (AP-1) in activated alveolar macrophages and monocytes, which subsequently hinders cytokine expression of gene 3,69.
Curcumin reduces inflammation, modulates mitogen-activated protein kinase (MAPK) pathway signaling and attenuates colitis in experiment models by reduction in p38 MAPK activation 73. In addition curcumin has been proven to attenuate neuropathic pain, nerve ischemia, demyelination, and decrease inflammation via subsequent activation of NF-kB and phosphorylation inhibition of IKK complex 74.
However, down-regulation of protein kinase C could be attributed to another anti-inflammatory mechanism of curcumin via inhibition of cytokine production and blocking of NF-kB activation by numerous inflammatory stimuli 5,69.
Anticancer Mechanism
In vitro and in vivo studies revealed that curcumin plays a vital role in anticancer mechanism via the inhibition of nuclear factor (NF)-kB and signal transducer and activator of transcription 3 (STAT3) pathway signals which are influential in cancer development and proliferation by resisting and inhibiting chemotherapy-induced apoptosis in several cancer cells 70,75,76.
Curcumin has been proposed to induce apoptosis which results in the down regulation of cMET cyclin D1, epidermal growth factor receptor (EGFR), and protein kinase B (PKB) in tumor cells 73. Furthermore, curcumin has been proved to stimulate apoptosis and inhibit cell cycle arrest and cell proliferation through the modulation of activation protein 1 (AP-1), early growth response protein 1 (EGR-1), peroxisome proliferator-activated receptor alpha (PPAR-α), Beta-catenin and other transcript factors 73,75,77.
Aoki et al. 76, reported that curcumin treatment can competently suppress malignant glioma cell growth as well as autophagy in U87 AND U373-MG cells in a dose-dependent manner. In addition, lung metastasis of breast cancer cells can be inhibited by curcumin or in mixture with paclitaxel.
Endoplasmic reticulum stress and DNA damage can be induced by curcumin resulting in mitochondrial-dependent programed cell death (apoptosis) in human cancer A-549 cells via the activation of caspase-3, and enhanced hepatocellular carcinoma apoptosis in HCC J5 cells via increase in intracellular utilization of Ca2+ and the disruption of reactive oxygen species (ROS) in the mitochondria 74,71.
Curcumin is instrumental in preventing cancer invasion and metastasis via inhibition of focal adhesion kinase (FAK) phosphorylation and improving extracellular matrix components expression. In addition, curcumin is known to increase cell adhesion via stimulation of extracellular matrix mechanisms of fibronectin, laminin and, collagen (I, III, IV, and IX) in a concentration reliant pattern, hence avoiding the migration and detachment of cancer cells 75.
Curative Evidence of Curcumin In vitro and In vivo Studies
Liver and Body Weight
In the work of Mahmoud et al. 62 reported that curcumin significantly decreases body weight in curcumin treated group by inhibiting the effect on hepatic gene expression in carbohydrate binding protein responsive elements and sterol regulatory binding protein elements, which subsequently stimulates the expression of lipogenic genes. In addition, curcumin as a dietary additive, may decrease the circulation of lipid by fatty acid synthase inhibition in adipocytes, leading to the decrease in lipid transportation to the liver, thus, hepatic lipid accumulation is inhibited 78,79,80.
The results obtained by Um et al. 79 revealed that microvesicular and macrovesicular steaosis within hepatic cells could be attenuated by dietary supplementation of curcumin in high fat diet (HFD) induced mice via improved hepatic lipid accumulation, thus, serum lipid profiles and insulin resistance are enhanced.
Moreover, curcumin action significantly reduces white adipose tissue macrophage infiltration, upswings adipose tissue adiponectin production and reduce the activity of hepatic NF-kB, hepatomegaly, and inflammation of the hepatocytes 62,81,82.
Nervous System
Neuroprotective mechanism against neurodegenerative disorders of the brain by curcumin is due to its ability to bind redox-active metal ions such as Mn2+, Cu2+, Cd2+, Pb2+, Hg2+ to produce a tight and active complex of antioxidant with its anti-inflammatory properties that are essential for the reduction in swelling among neuronal cells within the body 57.
As reported by Yuan et al. 61 post-treatment with curcumin in subarachnoid hemorrhage (SAH)-induced neurological damage in mice, improves brain functions by inhibiting microglia cells and down-regulating Matrix Metallopeptidase 9 (MMP-9), and finally reducing water content in the brain, hence, preserving the integrity of the blood brain barrier (BBB).
Curcumin possesses a robust antioxidant properties such as decreasing the generation of reactive oxygen species (ROS), moderation of antioxidant enzyme concentrations, subduing the peroxidation of lipid and reducing the level of malondialdehyde (MDA). Moreover, curcumin has been proved to decrease plaque pathogenesis by overpowering β-secretase activity, and eventually hinders oligomer and fibril production 60.
In a recent work by Shi et al. 58 it was reported that curcumin could reverse marked apoptosis and restore alteration in key Alzheimer’s disease (AD) linked proteins, such as amyloid precursor protein (APP), beta-amyloid enzyme 1 (BACE-1), and receptors for advanced glycation end products (RAGE) and decrease in A-disintegrin and metalloprotease (ADAM-10), after 24 hours acrolein induction in HTT22 Cells.
Wang et al. 81 also found that hippocampal and frontal neurons damage due to stress could be protected by curcumin through cyclic AMP response element binding protein (CREB) and BDNF/TrkB up-regulation. Moreover, neurotoxicity induced by nicotine could be protected by curcumin through revival of signal pathway of P-CREB/BDNF, thus, curcumin could reverse the inhibition of brain-derived neurotropic factor (BDNF) and tropomyosin receptor kinase B (TrkB) signaling due to acrolein induction 60,83,84.
Cardiovascular System
Cardiovascular diseases are pivotal threats to human health with atherosclerosis, vascular disease and heart disease being among contributing factors. Many researchers have proposed the application of phytochemicals such as curcumin to solve this problem based on its numerous benefits in antioxidant and anti-inflammation mechanisms 85.
Several research on curcumin showed that atherosclerosis can be alleviated through its anti-inflammatory mechanism against the oxidation of low- density lipoproteins inhibition of thrombocyte aggregation and cholesterol homeostasis modulation. In addition, curcumin supplementation is helpful in lipid peroxidation reduction, low-density lipoprotein-cholesterol (LDL) down-regulation and increasing high-density lipoprotein-cholesterol 86,87,88.
In vivo studies revealed that curcumin dietary supplementation in high fat-fed induced atherosclerotic rabbit decreases the levels of triglycerides and cholesterol, as well as inhibiting the oxidation of low density lipoproteins (LDL) 88. Moreover, atherosclerotic lesions in the aorta such as increased lipid peroxidation and increased oxidative stress in a similar model were showed to be improved by curcumin administration 86,88.
Similarly, cardiac hypertrophy, fibrosis and inflammation can be protected by curcumin via the inhibition of P300-histone acetyltransferase (HAT), downstream GATA4, NF-kB and further signaling pathways 85. Curcumin has been widely investigated to suppress excessive manifestation of lipopolysaccharide induced inflammatory mediators in rat vascular smooth muscle cells (VSMCs) by impeding TLR4-MAPK/NF-kB pathways as a result of blocking NADPH-mediated reactive oxygen species (ROS) intracellular production 89. Moreover, curcumin has been proven to trigger a programed cell death in H9c2 cells by stimulating the activity of JNKs and increasing reactive oxygen species (ROS) generation 85.
Curcumin ameliorates hematological parameters, which was indicated in restoring the alterations in white blood cells (WBCs), platelets, fibrinogens, red blood cells (RBCs) count and other hematological indices close to normal in high fat diet (HFD)-fed rats 90.
Renal System
Chronic kidney disease (CKD) is associated with continuous loss of renal functions that results in decreased glomerular filtration rate (GFR), abnormalities in urine content such as blood cells and proteins as well as accumulation of uremic toxins due to decreased ability of the kidney to remove soluble waste 91.
Ali et al. 90 reported that curcumin reduces creatinine clearance, increases the activity of urinary N-acetyl-B-D-glucosaminidase and uplifts the levels of neutrophil gelatinase-associated lipocalin against adenine-induced chronic kidney disease (CKD) toxicity in a dose dependent pattern in rats.
Curcumin is also shown to attenuate histopathological markers of apoptosis, fibrosis, inflammation, and morphological renal damage, which helps to restore the concentration of plasma sclerostin and bring down the oxidative stress in kidney homogenates 92.
Glomerular hemodynamic changes and oxidative stress seen in 5/6 nephrectomized rats can be reversed by curcumin via the attenuation in proteinuria and restructuring such as mesangial expansion, tubular atrophy, fibrotic glomeruli, and interstitial fibrosis. Importantly, hemodynamic alterations such as oxidative stress, hyperfiltration and glomerular hypertension were reduced by curcumin via increased antioxidant activity and decreased MDA 93.
Curcumin can be beneficial in uremia problem and treatment of intestinal dysbiosis, and can improve renal functions and defend the kidney against renal failure by decreasing the expression of mRNA of inflammatory proteins (monocyte chemoattractant protein-1) and down-regulating matrix proteins, collagen, TGF-B, and laminin 94.
Conclusions and future perspectives
Lead-induced toxicity could lead to serious irreversible health implications such as cardiovascular, renal, nervous, and hematopoietic system impairments which causes molecular, cellular, and intracellular alterations in living organisms through apoptosis, ionic mechanism and increased generation of oxidative stress.
In consideration to numerous established findings on the therapeutic applications and effects of curcumin on different organ-systems based on its antioxidant, anticancer, and anti-inflammatory mechanism of actions, curcumin could be used both as a protective and therapeutic agent against lead-induced toxicity (Figure 6).
Finally, in correlation to curcumin poor bioavailability, its poor absorption, fast metabolism, and rapid elimination by the gastrointestinal tract, it is recommended that a method of enhancing the bioavailability of curcumin should be carried out for future research.
Abbreviations
ALAD: Aminolevulinic acid dehydrates
ALA: Alpha-linolenic acid
BACE-1: Beta-amyloid enzyme 1
CAT: Catalase
CKD: Chronic kidney disease
GSH: Glutathione
HFD: High fat diet
LDL: Low density lipoprotein
MAPK: Mitogen-activated protein kinase
MDA: Malondialdehyde
MCP: Monocytes chemoattractant protein
ROS: Reactive oxygen species
SOD: Super oxide dismutase
STAT 3: Signal transducer and activator of transcription 3
TNF-a: Tumor necrosis factor-alpha
VSMCs: Vascular smooth muscle cells
WBCs: White blood cells
Competing Interests
The authors declare no conflicts of interest.
Authors' Contributions
All authors contributed to the drafting of this manuscript. AK and MMM searched, obtained and summarized the data. EAR, AD, and MZA review and edited the first draft of the manuscript. All authors reviewed, commented and approved the final draft of the manuscript.
References
-
Flora
G.,
Gupta
D.,
Tiwari
A.,
Toxicity of lead: A review with recent updates. Interdiscip Toxicol.
2012;
5
(2)
:
47-58
.
View Article PubMed Google Scholar -
Shaffer
R.M.,
Gilbert
S.G.,
Reducing occupational lead exposures: strengthened standards for a healthy workforce. Neurotoxicology.
2017;
69
:
181-186
.
View Article PubMed Google Scholar -
Edwards
R.L.,
Luis
P.B.,
Varuzza
P.V.,
Joseph
A.I.,
Presley
S.H.,
Chaturvedi
R.,
others
The anti-inflammatory activity of curcumin is mediated by its oxidative metabolites. J Biol Chem.
2017;
292
(52)
:
21243-52
.
View Article PubMed Google Scholar -
Carolina
A.,
Lopes
B.A.,
Peixe
T.S.,
Mesas
A.E.,
Reviews of Environmental Contamination and Toxicology Volume 236Springer Nature: Switzerland AG; 2016.
Google Scholar -
Hewlings
S.J.,
Kalman
D.S.,
Curcumin: A Review of Its' Effects on Human Health. Foods.
2017;
6
(10)
:
92
.
View Article PubMed Google Scholar -
Ajumobi
Olufemi Olamide,
Tsofo
Ahmed,
Yango
Matthias,
Aworh
Mabel Kamweli,
Anagbogu
Ifeoma Nkiruka,
Mohammed
Abdulazeez,
Umar-Tsafe
Nasir,
Mohammed
Suleiman,
Abdullahi
Muhammad,
Davis
Lora,
others
High concentration of blood lead levels among young children in Bagega community, Zamfara-Nigeria and the potential risk factor. The Pan African Medical Journal.
2014;
18
(Suppl 1)
.
-
WHO
Childhood lead poisoning prevention. JAMA J Am Med.
2010;
89
(7)
:
1129-30
.
-
Mazumdar
M.,
Bellinger
D.C.,
Gregas
M.,
Abanilla
K.,
Bacic
J.,
Needleman
H.L.,
Low-level environmental lead exposure in childhood and adult intellectual function: a follow-up study. Environ Heal.
2011;
10
(1)
:
24
.
View Article Google Scholar -
Needleman
H.,
Lead poisoning. Annu Rev Med.
2004;
55
(1)
:
209-22
.
View Article PubMed Google Scholar -
Dapul
H.,
Laraque
D.,
Lead poisoning in children. Adv Pediatr.
2014;
61
(1)
:
313-33
.
View Article PubMed Google Scholar -
Gerberding
J.L.,
Popovic
M. Director Tanja,
Stephens
J.W.,
Solomon
S.L.,
Bernhardt
J.M.,
Director
M.,
Centers for Disease Control and Prevention 2008.
Google Scholar -
Sanders
T.,
Liu
Y.,
Buchner
V.,
Tchounwou
P.B.,
Neurotoxic effects and biomarkers of lead exposure: a review. Rev Environ Health.
2009;
24
(1)
:
15-45
.
View Article PubMed Google Scholar -
Kosnett
M.J.,
Wedeen
R.P.,
Rothenberg
S.J.,
Hipkins
K.L.,
Materna
B.L.,
Schwartz
B.S.,
others
Recommendations for medical management of adult lead exposure. Environ Health Perspect.
2007;
115
(3)
:
463-71
.
View Article PubMed Google Scholar -
Chowdhury
R.,
Sarnat
S.E.,
Darrow
L.,
McClellan
W.,
Steenland
K.,
Mortality among participants in a lead surveillance program. Environ Res.
2014;
132
:
100-4
.
View Article PubMed Google Scholar -
ATSDR
Decision Support Systems 2007.
Google Scholar -
ATSDR
Lead Toxicity Case Studies in Environmental Medicine. Case Stud Environ Med.
2017;
(WB2832)
:
1-182
.
-
ATSDR
Agency for Toxic Substances and Disease Registry Case Studies in Environmental Medicine (CSEM) Lead Toxicity. Environ Heal Med Educ.
2015;
(WB1105)
:
71
.
-
Ekanem
A.U.,
Kwari
H.D.,
Garba
S.H.,
Salami
H.A.,
Effect of Lead Acetate on Spleen and Blood Parameters in Albino Rats. IOSR J Dent Med Sci Ver I.
2015;
14
(3)
:
2279–-861
.
-
Murata
K.,
Iwata
T.,
Dakeishi
M.,
Karita
K.,
Lead toxicity: does the critical level of lead resulting in adverse effects differ between adults and children?. J Occup Health.
2009;
51
(1)
:
1-12
.
View Article PubMed Google Scholar -
Ahijjo
Y.M.,
Baba-Kutigi
A.N.,
Momoh
M.,
Moreh
A.U.,
A Review of Sequential Extraction Method for Lead in Samples: A Case Study of Artisanal Mines of Sokoto Basin (Zamfara State). IJSRST.
2015;
2
(1)
:
105-9
.
-
Hassan
M.M.,
Nuhu
A.A.,
Sallau
M.S.,
Majiya
H.M.,
Mohammed
A.K.,
Zamfara lead poisoning saga% UNKNOWN UNICODE CHARACTER 0202F (NARROW NO-BREAK SPACE): comparison of lead contamination level of water samples and lead poisoning in Bagega Artisanal gold mining district, Nigeria. J Chem Pharm Res.
2015;
7
(3)
:
7-12
.
-
Holstege
CP,
Huff
JS,
Rowden
AK,
O’Malley
RN,
Pathophysiology and etiology of lead toxicity. Retrieved from Medscape Web site: http://emedicine. medscape. com/article/2060369-overview.
2013
.
-
Papanikolaou
N.C.,
Hatzidaki
E.G.,
Belivanis
S.,
Tzanakakis
G.N.,
Tsatsakis
A.M.,
Lead toxicity update. A brief review. Med Sci Monit.
2005;
11
(10)
:
RA329-36
.
PubMed Google Scholar -
Rabinowitz
M.B.,
Toxicokinetics of bone lead. Environ Health Perspect.
1991;
91
(5)
:
33-7
.
View Article PubMed Google Scholar -
Barbosa
Jr, F.,
Tanus-Santos
J.E.,
Gerlach
R.F.,
Parsons
P.J.,
A critical review of biomarkers used for monitoring human exposure to lead: advantages, limitations, and future needs. Environ Health Perspect.
2005;
113
(12)
:
1669-74
.
View Article PubMed Google Scholar -
Skerfving
S.,
Nilsson
U.,
Schütz
A.,
Gerhardsson
L.,
Biological monitoring of inorganic lead. Scand J Work Environ Health.
1993;
19
(May)
:
59-64
.
PubMed Google Scholar -
Philip
A.T.,
Philip At. Clin Lab Med.
1994;
14
:
84
.
-
Shukla
Vaibhav,
Shukla
Priyanka,
Tiwari
Avanish,
Lead poisoning. Indian Journal of Medical Specialities.
2018;
9
(3)
:
146-149
.
View Article Google Scholar -
Atsdr.Cdc.Gov
Potential for Human Exposure. AtsdrCdcGov.
2008;
3
:
247-308
.
-
Brochin
R.,
Leone
S.,
Phillips
D.,
Shepard
N.,
Zisa
D.,
Angerio
A.,
The Cellular Effect of Lead Poisoning and Its Clinical Picture. Georg Undergrad J Heal Sci..
2008;
5
(2)
:
1-8
.
-
Samarghandian
S.,
Azimi-Nezhad
M.,
Farkhondeh
T.,
Samini
F.,
Anti-oxidative effects of curcumin on immobilization-induced oxidative stress in rat brain, liver and kidney. Biomed Pharmacother.
2017;
87
:
223-9
.
View Article PubMed Google Scholar -
Djordjevic
J.,
Djordjevic
A.,
Adzic
M.,
Niciforovic
A.,
Radojcic
M.B.,
. Chronic Stress Differentially Affects Antioxidant Enzymes and Modifies the Acute Stress Response in Liver of Wistar Rats..
2010;
381
:
729-36
.
-
Szymanski
M.,
Molecular mechanisms of lead toxicity. J Biotechnol Comput Biol Bionanotechnol [Internet].
2014;
95
(2)
:
137-49
.
-
Hanas
J.S.,
Rodgers
J.S.,
Bantle
J.A.,
Cheng
Y.G.,
Lead inhibition of DNA-binding mechanism of Cys(2)His(2) zinc finger proteins. Mol Pharmacol.
1999;
56
(5)
:
982-8
.
View Article PubMed Google Scholar -
García-Lestón
J.,
Méndez
J.,
Pásaro
E.,
Laffon
B.,
Genotoxic effects of lead: an updated review. Environ Int.
2010;
36
(6)
:
623-36
.
View Article PubMed Google Scholar -
Breitenbach
M.,
Ralser
M.,
Perrone
G.G.,
Iglseder
B.,
Rinnerthaler
M.,
Dawes
I.W.,
Oxidative stress and neurodegeneration: the yeast model system. Front Biosci.
2013;
18
(3)
:
1174-93
.
View Article PubMed Google Scholar -
Flora
S.J.,
Flora
G.,
Saxena
G.,
Environmental occurrence, health effects and management of lead poisoning. 2006;
:
158-228
.
View Article Google Scholar -
Patrick
L.,
Lead toxicity, a review of the literature. Part 1: Exposure, evaluation, and treatment. Altern Med Rev.
2006;
11
(1)
:
2-22
.
PubMed Google Scholar -
Lidsky
T.I.,
Schneider
J.S.,
Lead neurotoxicity in children: basic mechanisms and clinical correlates. Brain.
2003;
126
(Pt 1)
:
5-19
.
View Article PubMed Google Scholar -
Garza
A.,
Vega
R.,
Soto
E.,
Cellular mechanisms of lead neurotoxicity. Med Sci Monit.
2006;
12
(3)
:
RA57-65
.
PubMed Google Scholar -
Mason
L.H.,
Harp
J.P.,
Han
D.Y.,
Mason
L.H.,
Harp
J.P.,
Han
D.Y.,
Pb neurotoxicity: neuropsychological effects of lead toxicity. BioMed Res Int.
2014;
2014
:
840547
.
View Article PubMed Google Scholar -
Rastogi
S.K.,
Renal effects of environmental and occupational lead exposure. Indian J Occup Environ Med.
2008;
12
(3)
:
103-6
.
View Article PubMed Google Scholar -
Odigie
IP,
Ladipo
CO,
Ettarh
RR,
Izegbu
MC,
Effect of chronic exposure to low levels of lead on renal function and renal ultrastructure in SD rats. Nigerian Journal of Physiological Sciences.
2004;
19
(1)
:
27-32
.
-
Lin
J.L.,
Tan
D.T.,
Hsu
K.H.,
Yu
C.C.,
JaLiang
L,
Environmental lead exposure and progressive renal insufficiency. Arch Intern Med.
2001;
161
(2)
:
264-71
.
View Article PubMed Google Scholar -
Mao
L.,
Qian
Q.,
Li
Q.,
Wei
S.,
Cao
Y.,
Hao
Y.,
others
Lead selenide nanoparticles-induced oxidative damage of kidney in rats. Environ Toxicol Pharmacol.
2016;
45
:
63-7
.
View Article PubMed Google Scholar -
Renner
R.,
Exposure on tap: drinking water as an overlooked source of lead. Environ Health Perspect.
2010;
118
(2)
:
A68-72
.
View Article PubMed Google Scholar -
Ambrose
Theresa M,
Al-Lozi
Muhammad,
Scott
Mitchell G,
Bone lead concentrations assessed by in vivo X-ray fluorescence. Clinical Chemistry.
2000;
46
(8)
:
1171-1178
.
-
Assi
M.A.,
Hezmee
M.N.,
Haron
A.W.,
Sabri
M.Y.,
Rajion
M.A.,
The detrimental effects of lead on human and animal health. Vet World.
2016;
9
(6)
:
660-71
.
View Article PubMed Google Scholar -
Apostoli
P.,
Catalani
S.,
Metal ions affecting reproduction and development. Met Ions Life Sci.
2011;
8
:
263-303
.
PubMed Google Scholar -
Lubran
M.M.,
Lead toxicity and heme biosynthesis. Ann Clin Lab Sci.
1980;
10
(5)
:
402-13
.
PubMed Google Scholar -
Schwartz
J.,
Landrigan
P.J.,
Baker
Jr, E.L.,
Orenstein
W.A.,
von Lindern
I.H.,
Lead-induced anemia: dose-response relationships and evidence for a threshold. Am J Public Health.
1990;
80
(2)
:
165-8
.
View Article PubMed Google Scholar -
Alwaleedi
S.A.,
Hematobiochemical changes induced by lead intoxication in male and female albinomice. Natl J Physiol Pharm Pharmacol.
2016;
6
(1)
:
46-51
.
View Article Google Scholar -
TÜRKAY
M.,
H. TÜRKER,
T. GÜVEN,
Ultrastructural effects of lead acetate on the spleen of rats. Turk J Biol.
2015;
39
(3)
:
511-6
.
-
Mohiuddin
E.,
Asif
M.,
Sciences
A.,
Curcuma Longa and Curcumin : a Review Article. Plant Biol.
2010;
55
(2)
:
65-70
.
-
Schiborr
C.,
Kocher
A.,
Behnam
D.,
Jandasek
J.,
Toelstede
S.,
Frank
J.,
The oral bioavailability of curcumin from micronized powder and liquid micelles is significantly increased in healthy humans and differs between sexes. Mol Nutr Food Res.
2014;
58
(3)
:
516-27
.
View Article PubMed Google Scholar -
Madhavi
D.,
Kagan
D.,
Bioavailability of a Sustained Release Formulation of Curcumin. Integr Med (Encinitas).
2014;
13
(3)
:
24-30
.
PubMed Google Scholar -
Mary
C.P.,
Vijayakumar
S.,
Shankar
R.,
Metal chelating ability and antioxidant properties of Curcumin-metal complexes - A DFT approach. J Mol Graph Model.
2018;
79
:
1-14
.
View Article PubMed Google Scholar -
Wang
Y.J.,
Pan
M.H.,
Cheng
A.L.,
Lin
L.I.,
Ho
Y.S.,
Hsieh
C.Y.,
others
Stability of curcumin in buffer solutions and characterization of its degradation products. J Pharm Biomed Anal.
1997;
15
(12)
:
1867-76
.
View Article PubMed Google Scholar -
Basnet
P.,
Skalko-Basnet
N.,
Curcumin: an anti-inflammatory molecule from a curry spice on the path to cancer treatment. Molecules.
2011;
16
(6)
:
4567-98
.
View Article PubMed Google Scholar -
Shi
L.Y.,
Zhang
L.,
Li
H.,
Liu
T.L.,
Lai
J.C.,
Wu
Z.B.,
Protective effects of Curcumin on acrolein-induced neurotoxicity in HT22 mouse hippocampal cells. Pharmacol Reports .
2018;
70
(5)
:
1040-1046
.
View Article Google Scholar -
Alisi
I.O.,
Uzairu
A.,
Abechi
S.E.,
Idris
S.O.,
Evaluation of the antioxidant properties of curcumin derivatives by genetic function algorithm. J Adv Res.
2018;
12
:
47-54
.
View Article PubMed Google Scholar -
Mahmoud
A.A.,
Abdelrahman
A.,
Abd el Aziz
H.O.,
Protective effect of curcumin on the liver of high fat diet-fed rats. Gene Rep.
2018;
11
(January)
:
18-22
.
View Article Google Scholar -
Yuan
J.,
Liu
W.,
Zhu
H.,
Zhang
X.,
Feng
Y.,
Chen
Y.,
others
Curcumin attenuates blood-brain barrier disruption after subarachnoid hemorrhage in mice. J Surg Res.
2017;
207
(30)
:
85-91
.
View Article PubMed Google Scholar -
Baum
L.,
Ng
A.,
Curcumin interaction with copper and iron suggests one possible mechanism of action in Alzheimer's disease animal models. J Alzheimers Dis.
2004;
6
(4)
:
367-77
.
View Article PubMed Google Scholar -
Anand
P.,
Kunnumakkara
A.B.,
Newman
R.A.,
Aggarwal
B.B.,
Bioavailability of curcumin: problems and promises. Mol Pharm.
2007;
4
(6)
:
807-18
.
View Article PubMed Google Scholar -
Shoba
G.,
Joy
D.,
Joseph
T.,
Majeed
M.,
Rajendran
R.,
Srinivas
P.S.,
Influence of piperine on the pharmacokinetics of curcumin in animals and human volunteers. Planta Med.
1998;
64
(4)
:
353-6
.
View Article PubMed Google Scholar -
Jovanovic
S.V.,
Steenken
S.,
Boone
C.W.,
H-Atom transfer is a preferred antioxidant mechanism of curcumin. . J Am Chem Soc.
1999;
121
:
9677-81
.
View Article Google Scholar -
Priyadarsini
K.I.,
Maity
D.K.,
Naik
G.H.,
Kumar
M.S.,
Unnikrishnan
M.K.,
Satav
J.G.,
Role of phenolic O-H and methylene hydrogen on the free radical reactions and antioxidant activity of curcumin. Free Radic Biol Med.
2003;
35
(5)
:
475-84
.
View Article PubMed Google Scholar -
Jurenka
J.S.,
Anti-inflammatory properties of curcumin, a major constituent of Curcuma longa: a review of preclinical and clinical research. Altern Med Rev.
2009;
14
(2)
:
141-53
.
PubMed Google Scholar -
Wilken
R.,
Veena
M.S.,
Wang
M.B.,
Srivatsan
E.S.,
Curcumin: A review of anti-cancer properties and therapeutic activity in head and neck squamous cell carcinoma. Mol Cancer.
2011;
10
(1)
:
12
.
View Article PubMed Google Scholar -
Huang
M.T.,
Robertson
F.M.,
Lysz
T.,
Ferraro
T.,
Wang
Z.Y.,
Georgiadis
C.A.,
Inhibitory effects of curcumin on carcinogenesis in mouse epidermis. InACS symposium series (USA).
1992;
507
:
338-49
.
-
Kohli
K.,
Ali
J.,
Ansari
M.J.,
Raheman
Z.,
Cur cumin : A natural antiinflammatory agent Curcumin. Indian J Pharmacol.
2005;
37
(3)
:
141-7
.
View Article Google Scholar -
Shehzad
A.,
Lee
Y.S.,
Molecular mechanisms of curcumin action: signal transduction. Biofactors.
2013;
39
(1)
:
27-36
.
View Article PubMed Google Scholar -
Kazazis
C.,
Vallianou
N.G.,
Kollas
A.,
Evangelopoulos
A.,
Curcumin and diabetes: mechanisms of action and its anti-diabetic properties. Curr Top Nutraceutical Res.
2014;
12
(4)
:
135-42
.
-
Vallianou
N.G.,
Evangelopoulos
A.,
Schizas
N.,
Kazazis
C.,
Curcumin and Sp-1. Anticancer Res.
2015;
652
(35)
:
645-51
.
PubMed Google Scholar -
Panda
A.K.,
Chakraborty
D.,
Sarkar
I.,
Khan
T.,
Sa
G.,
New insights into therapeutic activity and anticancer properties of curcumin. J Exp Pharmacol.
2017;
9
:
31-45
.
View Article PubMed Google Scholar -
Davie
J.R.,
He
S.,
Li
L.,
Sekhavat
A.,
Espino
P.,
Drobic
B.,
Nuclear organization and chromatin dynamics\textemdashSp1, Sp3 and histone deacetylases. Adv Enzyme Regul.
2008;
48
(1)
:
189-208
.
View Article PubMed Google Scholar -
Aoki
H.,
Takada
Y.,
Kondo
S.,
Sawaya
R.,
Aggarwal
B.B.,
Kondo
Y.,
Evidence that curcumin suppresses the growth of malignant gliomas in vitro and in vivo through induction of autophagy: role of Akt and extracellular signal-regulated kinase signaling pathways. Mol Pharmacol.
2007;
72
(1)
:
29-39
.
View Article PubMed Google Scholar -
Ejaz
A.,
Wu
D.,
Kwan
P.,
Meydani
M.,
Curcumin inhibits adipogenesis in 3T3-L1 adipocytes and angiogenesis and obesity in C57/BL mice. J Nutr.
2009;
139
(5)
:
919-25
.
View Article PubMed Google Scholar -
Zhao
J.,
Sun
X.B.,
Ye
F.,
Tian
W.X.,
Suppression of fatty acid synthase, differentiation and lipid accumulation in adipocytes by curcumin. Mol Cell Biochem.
2011;
351
(1-2)
:
19-28
.
View Article PubMed Google Scholar -
Um
M.Y.,
Hwang
K.H.,
Ahn
J.,
Ha
T.Y.,
Curcumin attenuates diet-induced hepatic steatosis by activating AMP-activated protein kinase. Basic Clin Pharmacol Toxicol.
2013;
113
(3)
:
152-7
.
View Article PubMed Google Scholar -
Weisberg
S.P.,
Leibel
R.,
Tortoriello
D.V.,
Dietary curcumin significantly improves obesity-associated inflammation and diabetes in mouse models of diabesity. Endocrinology.
2008;
149
(7)
:
3549-58
.
View Article PubMed Google Scholar -
Wang
R.,
Li
Y.H.,
Xu
Y.,
Li
Y.B.,
Wu
H.L.,
Guo
H.,
others
Curcumin produces neuroprotective effects via activating brain-derived neurotrophic factor/TrkB-dependent MAPK and PI-3K cascades in rodent cortical neurons. Prog Neuropsychopharmacol Biol Psychiatry.
2010;
34
(1)
:
147-53
.
View Article PubMed Google Scholar -
Motaghinejad
M.,
Motevalian
M.,
Fatima
S.,
Faraji
F.,
Mozaffari
S.,
The Neuroprotective Effect of Curcumin Against Nicotine-Induced Neurotoxicity is Mediated by CREB-BDNF Signaling Pathway. Neurochem Res.
2017;
42
(10)
:
2921-32
.
View Article PubMed Google Scholar -
He
Y.,
Yue
Y.,
Zheng
X.,
Zhang
K.,
Chen
S.,
Du
Z.,
Curcumin, inflammation, and chronic diseases: how are they linked?. Molecules.
2015;
20
(5)
:
9183-213
.
View Article PubMed Google Scholar -
Kapakos
G.,
Youreva
V.,
Srivastava
A.K.,
Cardiovascular protection by curcumin: molecular aspects. Indian J Biochem Biophys.
2012;
49
(5)
:
306-15
.
PubMed Google Scholar -
Li
Xiaolong,
Lu
Yan,
Sun
Yi,
Zhang
Qi,
Effect of curcumin on permeability of coronary artery and expression of related proteins in rat coronary atherosclerosis heart disease model. International journal of clinical and experimental pathology.
2015;
8
(6)
:
7247
.
-
Ramírez-Tortosa
M.C.,
Mesa
M.D.,
Aguilera
M.C.,
Quiles
J.L.,
Baró
L.,
Ramirez-Tortosa
C.L.,
Oral administration of a turmeric extract inhibits LDL oxidation and has hypocholesterolemic effects in rabbits with experimental atherosclerosis. Atherosclerosis.
1999;
147
(2)
:
371-8
.
View Article PubMed Google Scholar -
Meng
Z.,
Yan
C.,
Deng
Q.,
Gao
D.F.,
Niu
X.L.,
Curcumin inhibits LPS-induced inflammation in rat vascular smooth muscle cells in vitro via ROS-relative TLR4-MAPK/NF-κB pathways. Acta Pharmacol Sin.
2013;
34
(7)
:
901-11
.
View Article PubMed Google Scholar -
El-Habibi
EM,
El-Wakf
AM,
Mogall
A,
Efficacy of curcumin in reducing risk of cardiovascular disease in high fat diet-fed rats. Journal of Bioanalysis and Biomedicine.
2013;
5
:
66-70
.
-
Ghosh
S.S.,
Gehr
T.W.,
Ghosh
S.,
Curcumin and chronic kidney disease (CKD): major mode of action through stimulating endogenous intestinal alkaline phosphatase. Molecules.
2014;
19
(12)
:
20139-56
.
View Article PubMed Google Scholar -
Ali
B.H.,
Al-Salam
S.,
Al Suleimani
Y.,
Al Kalbani
J.,
Al Bahlani
S.,
Ashique
M.,
others
Curcumin Ameliorates Kidney Function and Oxidative Stress in Experimental Chronic Kidney Disease. Basic Clin Pharmacol Toxicol.
2018;
122
(1)
:
65-73
.
View Article PubMed Google Scholar -
Tapia
Edilia,
Soto
Virgilia,
Ortiz-Vega
Karla Mariana,
Zarco-Márquez
Guillermo,
Molina-Jijón
Eduardo,
Cristóbal-García
Magdalena,
Santamaría
José,
García-Niño
Wylly Ramsés,
Correa
Francisco,
Zazueta
Cecilia,
others
Curcumin induces Nrf2 nuclear translocation and prevents glomerular hypertension, hyperfiltration, oxidant stress, and the decrease in antioxidant enzymes in 5/6 nephrectomized rats. Oxidative medicine and cellular longevity.
2012;
2012
.
-
de Almeida Alvarenga
L.,
Curcumin - A promising nutritional strategy for chronic kidney disease patients. J Funct Foods.
2017;
2018
(40)
:
715-21
.
View Article Google Scholar
Comments
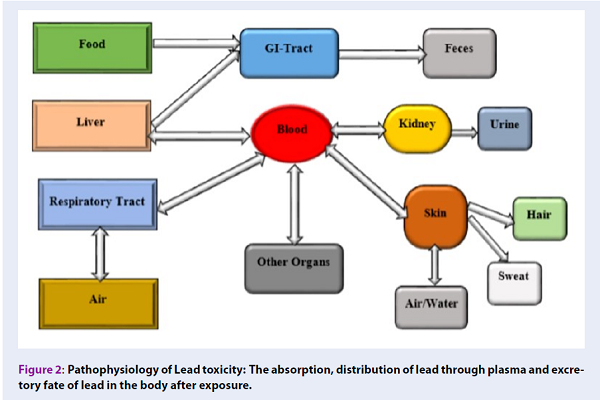
Downloads
Article Details
Volume & Issue : Vol 6 No 3 (2019)
Page No.: 3053-3066
Published on: 2019-03-31
Citations
Copyrights & License

This work is licensed under a Creative Commons Attribution 4.0 International License.
Search Panel
Pubmed
Google Scholar
Pubmed
Google Scholar
Pubmed
Google Scholar
Pubmed
Google Scholar
Pubmed
Search for this article in:
Google Scholar
Researchgate
- HTML viewed - 12576 times
- View Article downloaded - 0 times
- Download PDF downloaded - 3007 times