Abstract
Introduction: We have previously demonstrated that sequentially extracted methanolic fractions of Boerhaavia diffusa (Linn.) showed marked antioxidant, antidiabetic and oxidative-DNA damage protective properties in vitro. The present study was undertaken to evaluate the beneficial effects of B.diffusa (Linn.) methanolic root extract and its partially purified bioactive fraction on streptozotocin (STZ)-induced hyperglycemia and hyperlipidemia in rats.
Methods: The diabetic rats were treated for fourteen weeks either with methanolic extract of B. diffusa root (D-MT1, D-MT2, and D-MT3 : doses of 50, 150, and 300 mg/rat/day, respectively), partially isolated bioactive fraction (DBT: 0.5 mg/rat/day), or glibenclamide (D-GT: 0.5 mg/rat/day).
Results: The level of fasting blood glucose (FBG) and glycated hemoglobin (HbA1c) were significantly alleviated in D-MT- and D-BT treated groups after fourteen weeks of administration. Moreover, plasma lipid profile, free fatty acids (FFAs), phospholipids (PLs), HMG-CoA reductase (HMG-R) activity, conjugated diene (CD), lipid hydroperoxide (LOOH), and malondialdehyde (MDA) were also markedly ameliorate d in all treatment groups. In addition, the activity of antioxidant enzymes, e.g., superoxide dismutase (SOD), catalase (CAT), glutathione peroxidase (GPx), glutathione reductase (Gred), and glutathione-S-transferase (GST), were also significantly restored by D-MT — and D-BT — treated groups. Furthermore, histologically, all the unseemly features of nephropathy were extensively regressed and normalized by the administration of B. diffusa and its bioactive fraction.
Conclusions: Our results demonstrate a strong antidiabetic and hypolipidemic impact of B. diffusa extract an ideal alternative therapeutic agent in the prevention and treatment of diabetes as well as diabetes-linked hyperlipidemia.
Introduction
Diabetes is a chronic disorder of carbohydrate, fat and protein metabolism, which is characterized by increased fasting and postprandial blood sugar levels1. The World Health Organization (WHO) has predicted that significant health burden and mortality will occur in developing countries. In India, diabetes has become one of the major causes of mortality and is anticipated to affect around 79.4 million people in India by 2004,. Experimental evidence have suggested the involvement of free radicals in the pathogenesis of diabetes and, more importantly, in the development of diabetic complications4. Free radicals are capable of damaging cellular molecules, DNA, proteins and lipids, leading to altered cellular functions. For the development of diabetic complications, the abnormalities produced in lipids and proteins are the major etiologic factors4. In diabetic patients, extracellular and long-lived proteins, such as elastin, laminin and collagen, are the major targets of free radicals. These proteins are modified to form glycoproteins due to hyperglycemia5. The modification of these proteins present in tissues, such as lens, vascular wall, and basement membranes, are associated with the development of complications of diabetes, such as cataracts, microangiopathy, atherosclerosis and nephropathy6. Circulating risk factors like hyperglycemia, increased free fatty acids (FFAs), and hypertension, all of which are common in diabetes, can damage endothelial cells (ECs), leading to their dysfunction7.
Currently available antidiabetic drug classes like insulin sensitizers, dipeptidyl peptidase-4 (DPP-IV) inhibitors, sodium-glucose cotransporter (SGLT-2) inhibitors, insulin, and GLP-1 agonists are extensively prescribed. However, they possess various adverse effects which include pancreatitis, ketoacidosis, increased low-density lipoprotein (LDL) cholesterol (LDL-C), bone fractures, lipoatrophy and lipohypertrophy, to name a few8. Therefore, drugs from the natural origin are receiving more importance because of their safety. In some investigations, attempts have been made to study the indigenous plants, which show the inhibitory effect of glucose utilization and are used as antidiabetic agents in the traditional system of medicine9.
Boerhaavia diffusa, also known as Punarnava, is a representative of the family Nyctaginaceae and has been found to be of great medicinal value,. In continuation to our previous in vitro study which demonstrated the antioxidant, α-amylase inhibitory and oxidative DNA damage protective properties of Boerhaavia diffusa (Linn.) root extracts11, the current study is an initial report demonstrating and correlating the antioxidant, antidiabetic, hypolipidemic, HMG-R inhibitory, and nephroprotective properties of B. diffusa root methanolic extract (and its bioactive fraction) in streptozotocin (STZ)-mediated experimental diabetes.
Materials and methods
Chemicals, extraction, and isolation of bioactive fraction of B. diffusa (Linn.) root extract
All chemicals and reagents used in this study were of analytical grade. Previously, we have published the methodology of collection, processing, and extraction of B. diffusa (Linn.) roots11. Briefly, B.diffusa (Linn.) roots were collected from the Pharmacy-herbal garden (Integral University) and authenticated at the National Botanical Research Institute (NBRI) in Lucknow, Uttar Pradesh, India (specimen voucher no. 98070). Approximately 25 g of fresh shade- dried plant roots were crushed to powder form, wrapped in muslin cloth, and subjected to Soxhlet-based extraction using 0.25 L of various solvents, including methanol (CH3OH), ethanol (CH3CH2OH) and water (H2O).
The percolation process was repeated until the solvent became colorless ; the extract was filtered, allowed to cool, left for drying and stored at 4°C for future use. Following the extraction, the methanolic, ethanolic and aqueous extracts were analyzed for their free radical scavenging activity by 2, 2-diphenyl-1-picrylhydrazyl (DPPH) assay in which CH3 OH and CH2 OH extracts showed greater antioxidant potential.
Both these extracts were then analyzed for preliminary and tentative phytochemical analysis using thin layer chromatography (TLC) by subsequently spraying different reagents on the developed chromatograms. Following the phytochemical analysis, we analyzed the antioxidant content of these extracts on TLC plates by DPPH assay in order to detect the band of bioactive compounds that was confirmed by the formation of yellow color upon exposure to 0.1 % DPPH spray11. Repetitive preparatory TLC was done to obtain the bioactive fraction band. The obtained bioactive fraction from B. diffusa (Linn.) root methanolic extract was further subjected to high performance liquid chromatography (HPLC) analysis using reverse phase C-18 column and UV detector (280 nm). W e observed a single peak (without shouldering) with the TR of 2.53 min (% area: 75.23 mV), which showed that the bioactive fraction was almost pure11. Based on our previou s in vitro antioxidant, α-amylase inhibitory and oxidative-DNA-damage protective properties11, we selected B. diffusa root methanolic extract and its purified fraction for the current in vivo study.
Animals, induction of diabetes, and interventional strategy
Male albino rats, weighing about 175 g to 220 g were purchased from Central Drug Research Institute (CDRI), Lucknow, India, and acclimatized to the animal house environment prior to the experimental study. The interventional study design was approved by the Institutional Animal Ethics Committee (IAEC) (Approval No.: IU/Biotech Project/CPCSEA/14/17). The rats were given pelleted rat chow and water ad libitum. In order to induce experimental diabetes, STZ (freshly dissolved in 10 mM citrate buffer, pH 4.5, 60 mg/kg b.w t.) was injected intraperitoneally (i.p.) to 30 overnight -fasted rats12. Male albino wistar rats in the normal control (NC) group were injected with buffer only. After 12 days, fasting plasma glucose (FBG) level was determined to designate the rats as diabetic or non-diabetic. Both the B. diffusa methanolic root extract and its bioactive fraction were administered through gastric intubations in different doses. The doses were adjusted according to the previous study where the lethal dose of B. diffusa root ex tract was greater than 500 mg/kg b.wt.13. The diabetic rats were treated for 14 weeks either with methanolic extract of B. diffusa root (D-MT1, D-MT2, and D-MT3: doses of 50, 150, and 300 mg/rat/day, respectively), partially isolated bioactive fraction (D-BT: 0.5 mg/rat/day), or glibenclamide (D-GT: 0.5 mg/rat/day). The treatment scheme is shown in Table 1.
Determination of plasma glucose and glycosylated hemoglobin
An enzymatic “Autospan kit” was used for the quantitative determination of FBG in accordance with the manufacturer’s instructions. The quantification was based on the Glucose Oxidase-Peroxidase (GOD-POD) method. Glycosylated hemoglobin (HbA1c) was estimated by using Accucare Glycosylated Hemoglobin-A1c kit supplied by Lab Care Diagnostics Pvt. Ltd. (Valsad, India).
Determination of cholesterol content in plasma
Plasma low-density lipoprotein (LDL) and high-density lipoprotein (HDL) were isolated according to the standard methods,. Plasma total cholesterol (TC), LDL cholesterol (LDL-C), and HDL cholesterol (HDL-C) levels were assayed using a cholesterol enzymatic kit (Merck, India), based on cholesterol oxidase phenol aminophenazone (CHODPAP) method, whereas triglyceride (TG) level was determined using an enzymatic kit (Merck, India) based on glycerol-3-phosphate oxidase peroxide (GPO-POD) method. The very low-density lipoprotein cholesterol (VLDL-C) level was calculated by dividing plasma TG values (mg/dl) by a factor of 5.
Determination of plasma free fatty acid (FFA) and phospholipid (PL) content
The procedure of Folch et al. was used for extracting free fatty acids (FFAs) from plasma lipids16. FFA was estimated as described by Khawaja et al., using palmitic acid as a standard17. The estimation of phospholipids (PLs) was performed according to the method of Zou et al. 18.
Assay of 3-hydroxy-3-methyl-glutaryl-CoA (HMG-CoA) reductase activity in liver homogenate
The hepatic 3-hydroxy-3-methyl-glutaryl-coenzyme A reductase (HMG-R) activity in liver homogenate was estimated indirectly, as described by Alvi et al.19.
Estimation of lipid peroxidation products in plasma, liver and kidney homogenates
In order to estimate lipid peroxidation by-products of plasma, liver and kidney, the lipids were initially extracted following the procedure of Folch et al. 6. The method of Beuje and Aust 20 was applied to determine the level of conjugated dienes (CD), whereas the quantification of lipid hydroperoxide (LOOH) was done according to the method of Naurooz-Zadeh et al. 21. The level of malondialdehyde (MDA) was determined using thiobarbituric acid (TBA) reaction22, which measures the color produced by the reaction of TBA with MDA at 532 nm.
Activities of antioxidant enzymes
The enzymatic activities of catalase (CAT) and superoxide dismutase (SOD) in liver post-mitochondrial supernatant (PMS) were assayed using the procedure of Sinha 23 and Kakkar et al. 24, respectively. Glutathione peroxidase (GPx) activity was determined using the method of Hafeman et al. 25. Glutathione reductase (GRed) activity was evaluated using the procedure of Carlberg and Mannervik26. Furthermore, glutathione-S-transferase (GST) activity of liver PMS fraction was assessed by the procedure of Habig et al. 27.
Histopathological studies
For histopathological studies related to nephropathy, sections of kidney were used. For microscopic preparation s of the above tissues, formalin-fixed samples from each tissue were embedded in paraffin and sectioned after block preparation. The sections were double-stained with Ehrlich’s hematoxylin and eosin (H&E) followed by microscopic visualization and photography.
Statistical analysis
The samples were taken in triplicate for all the biochemical measurements, and data were represented as mean ± SD. The statistical significance was evaluated using one-way analysis of variance (ANOVA), followed by Post Hoc Tukey-Kramer multiple comparisons test, using GraphPad Prism version 4.02 for Windows (GraphPad Software, San Diego, CA, USA)9.
Results
Boerhaavia diffusa root extract and its bioactive fraction ameliorate plasma glucose and HbA1C levels
STZ is known to cause diabetes and raise blood glucose. In our in vivo study, the level of plasma glucose was found to be significantly elevated from 87.38 to 333.32 mg/d l (+281.46%) after the induction of diabetes through STZ in DC rats (diabetic control). This elevated plasma glucose was significantly reduced in all diabetic rats treated with the methanolic extract of B. diffusa root (D-MT) with a maximum restoration of -69.22% (101.31 mg/dl) in D-MT-3 rats when compared to corresponding DC rats. Similarly, the very low dose (0.5 mg/kg b.wt.) of the bioactive fraction (D-BT) significantly restored plasma glucose level with a decline of -70.78% (95.29 mg/dl), when compared to DC rats. Furthermore, treatment with the standard drug (D-GT) also decreased the plasma glucose level in STZ-induced rats by -71.25% (92.12 mg/dl), when compared to DC rats (Figure 1 a).
Group designation | No. of animals (n) | Diet and doses |
NC | 5 | Normal rat chow + water ad libitum throughout the experiment + Citrate buffer as STZ vehicle (single intraperitonial injection) |
DC | 5 | STZ (60mg/kg B.Wt/Rat) dissolved in citrate buffer |
D-MT1 | 5 | STZ + B. diffusa methanolic root extract at 50 mg/kg B.Wt/Rat for fourteen weeks |
D-MT2 | 5 | STZ + B. diffusa methanolic root extract at 150 mg/kg B.Wt/Rat for fourteen weeks |
D-MT3 | 5 | STZ + B. diffusa methanolic root extract at 300 mg/kg B.Wt/Rat for fourteen weeks |
D-BT | 5 | STZ + Bioactive fraction at 0.5 mg/kg B.Wt/Rat for fourteen weeks |
D-GT | 5 | STZ + Glibenclamide at 0.5 mg/kg B.Wt/Rat for fourteen weeks |
Moreover, persistent hyperglycemia also leads to the formation of glycated adducts of plasma hemoglobin, called glycosylated hemoglobin (HbA1c). Similarly, the level of HbA1c, at the end of the experiment, w as found to be raised from 4.8 % to 9.87 % in untreated DC rats after the induction of diabetes. Treatment with D-MTs significantly decreased the HbA1c level with the maximum restoration in D-MT-3 treated rats, in which the level of HbA1c was found to be decreased by 39.53% when compared to DC rats. Similarly, D-BT treatment also showed a significant restoration in HbA1c level by -43% while the D-GT rats showed a restoration of -50% in HbA1c level when compared to DC rats (Figure 1 b).
Boerhaavia diffusa root extract and bioactive fraction normalize plasma lipid and lipoprotein levels
In STZ-induced diabetic rats, the increase in blood glucose and Hba1C levels was accompanied by an altered lipoprotein secretion. The level of TC was significantly increased from 146.27 to 230.45 mg/dl (+57.55%), whereas the level of TG was significantly increased from 81.12 to 226.20 mg/dl (+178.84 %) in STZ-induced DC rats when compared to corresponding NC rats. These elevated plasma TC and TG levels were markedly reduced in all the B. diffusa extract treated diabetic rats (D-MTs) with a maximum restoration of -32.64% (155.23 mg/dl) and -60.17 % (90.10 mg/dl), respectively, in D-MT-3 rats, when compared to corresponding DC rats. D-BT treatment also showed a marked reduction in TC and TG levels by 30.26 % (160.71 mg/dl) and 44.42 % (125.72 mg/dl), respectively, whereas D-GT treatment showed comparatively meager reduction of 13.21 % (200 mg/dl) and 12.10 % (198.83 mg/dl) in the levels of TC and TG, respectively (Figure 2 a).
N-C | D-C | D-MT 1 | D-MT 2 | D-MT 3 | D-BT | D-GT | |
GPx (U/gr.prot.) | 1.27±0.15 | 0.73±0.6 a (-42.52%) | 0.83±0.15 a,f (+13.70%) | 0.97±0.15 b,e (+32.88%) | 1.12±0.11 c,d (+53.42%) | 0.92±0.12 b,e (+26.03%) | 0.78±0.12 a,f (+6.85%) |
SOD (µgr/gr.prot.) | 23.69±1.2 | 16.01±1.15 a (-32.42%) | 17.21±1.1 a,f (+7.49%) | 19.46±1.18 a,e (+21.55%) | 21.79±1.2 a,d (+36.10%) | 19.17±1.1 a,e (+19.74%) | 17.19±1.2 a,f (+7.37%) |
CAT (kU/gr.prot.) | 487.1±6.5 | 322.6±5.9 a (-33.77%) | 331.3±5.1 a,f (+2.67%) | 343.3±5.92 a,f (+6.42%) | 399.6±6.7 b,d (+23.87%) | 378.1±5.3 b,e (+17.20%) | 341.5±7.3 a,f (+5.86%) |
GST (U/mg.prot.) | 9.91±0.31 | 4.96±0.12 a (-49.95%) | 6.06±0.13 a,e (+22.18%) | 7.08±0.17 a,d (+42.74%) | 8.14±0.26 b,d (+64.11%) | 7.01±0.17 a,d (+41.33%) | 4.99±0.14 a,f (+0.60.0%) |
GRed (µmolNADP min -1 .g -1 wet tissue wt) | 3.21±0.16 | 2.92±0.1 b (-9.35%) | 3.01±0.11 c,f (+3.08%) | 3.11±0.13 c,e (+6.51%) | 3.18±0.1 c,d (+8.90%) | 3.13±0.12 c,e (+7.19%) | 3.03±0.18 c,f (+3.77%) |
Additionally, we also reported that the PL and FFA levels were significantly elevated in DC rats by 104.38 % and 128.37 %, respectively, after the induction of diabetes through STZ, when compared to NC rats. These elevated plasma PL and FFA levels were markedly reduced in all the B. diffusa extract treated diabetic rats (D-MTs) with a maximum restoration of -42.11 % (98.5 mg/dl) and -46.90 % (80.42 mg/dl), respectively, in D-MT-3 rats, when compared to corresponding DC rats. Treatment with the bioactive fraction (D-BT) also showed a marked reduction in PL and FFA levels by 37.32% (106.61 mg/dl) and 30.75% (104.86 mg/dl), respectively, whereas D-GT treatment showed comparatively very low reduction of 11.70% (150.23 mg/dl) and 17.87% (124.36 mg/dl) in the levels of PL and FFA, respectively, when compared to the PL and FFA levels of corresponding DC rats (Figure 2 a).
On the other hand, the level of LDL-C was significantly increased by 83.73 %, whereas the level of HDL-C showed a decline of 37.67 % in STZ-induced DC rats when compared to NC rats. These levels were restored in all the D-MT treated diabetic rats with a maximum restoration of -39.49% (93.87 mg/dl) and +49.12% (37.40 mg/dl) in the level of LDL-C and HDL-C, respectively, in D-MT3 rats, whereas D-BT treatment showed a restoration of -40.48% and +40.15 % in the level of LDL-C and HDL-C, respectively, when compared to corresponding DC rats. In contrast, D-GT treatment did not show any significant amelioration in LDL-C and HDL-C levels when compared to DC rats. In addition to the TG levels, the level of VLDL-C was also significantly raised - by 178.84 % in DC rats- when compared to NC rats. D-MT1, D-MT2 and D-MT3 treatment markedly restored the level of VLDL-C by 32.56%, 54.80%, and 60.16 %, respectively, whereas D-BT showed a significant reduction of 44.52% in VLDL-C level when compared to corresponding DC rats (Figure 2 b).
N-C | D-C | D-MT 1 | D-MT 2 | D-MT 3 | D-BT | D-GT | |
GPx (U/gr.prot.) | 1.49±0.30 | 1.04±0.13 a (-30.20%) | 1.16±0.13 a,g (+11.54%) | 1.27±0.11 a,e (+22.11%) | 1.39±0.12 c,e (+33.65%) | 1.21±0.3 a,f (+16.35%) | 1.19±0.14 a,f (+14.42%) |
SOD (µg/gr.prot.) | 21.95±1.04 | 15.90±1.02 a (-27.56%) | 17.12±1.08 a,f (+7.67%) | 18.86±1.15 b,f (+18.62%) | 20.23±1.09 c,e (+27.23%) | 18.13±1.02 b,f (+14.02%) | 18.16±1.10 b,f (+14.21%) |
CAT (kU/gr.prot.) | 475.26±7.19 | 255.3±4.35 a (-46.28%) | 267.1±4.24 a,h (+4.62%) | 293.6±5.16 b,g (+15.0%) | 389.3±5.73 b,f (+52.49%) | 359.1±5.62 b,f (+40.66%) | 297.7±6.01 b,g (+16.61%) |
GST (U/mg.prot.) | 26.13±1.51 | 9.62±0.47 a (-63.18%) | 13.35±1.12 b,f (+38.77%) | 17.0±1.15 b,f (+76.71%) | 22.34±1.25 c,e (+132.22%) | 17.34±1.20 b,e (+80.25%) | 16.26±1.12 b,f (+69.03%) |
GRed (µmol.g 1 tissue) | 5.20±0.20 b | 4.63±0.15 b (-10.96%) | 4.87±0.18 c,h (+5.18%) | 5.03±0.24 d,f (+8.64%) | 5.18±0.22 d,e (+11.88%) | 5.17±0.28 d,e (+11.66%) | 5.14±0.20 d,e (+11.01%) |
Boerhaavia diffusa root extract and bioactive fraction restore hepatic HMG-R activity
The hypolipidemic potential of D-MTs and D-BT was assessed by evaluating the activity of hepatic HMG-R. We found that the activity of hepatic HMG-R was significantly upregulated (+2.13- fold) in STZ-induced diabetic rats when compared to corresponding NC rats. Subsequent treatment with D-MTs resulted in a marked amelioration of hepatic HMG-R activity with a maximum restoration of -65.62% in D-MT3 rats, when compared to corresponding DC rats. In contrast, the HMG-R activity in rats from D-MT3 group was almost comparable to the HMG-R activity of the NC rats. Surprisingly, we also reported a significant inhibition of HMG-R activity (-55.18%) in the D-BT treated rats, when compared to corresponding DC rats, whereas the reference standard drug treatment (D-GT) showed an inhibition of HMG-R activity only by 42.5%, when compared to DC rats (Figure 3 ).
Boerhaavia diffusa root extract and bioactive fraction reduce systemic lipid peroxidation in STZ-induced rats
Effects on plasma lipid peroxidation
Streptozotocin and alloxan are well-known to increase the rate of peroxidation to generate various lipid peroxidation products. As shown in Figure 4 a, w e report that the level s of plasma CD, LOOH, and MDA were increase d significantly by 112.55%, 65.93%, and 98.04%, respectively, in STZ-induced DC rats. Subsequent treatment with D-MTs markedly reduced the levels of CD, LOOH, and MDA, with a maximum restoration of 34.48%, 38.41%, and 47.93%, respectively, in D-MT3 group, when compared to corresponding DC rats. D-BT treated rats also showed a significant decline of 31.26%, 26.49%, and 43.80% in plasma CD, LOOH and MDA levels, respectively, whereas D-GT rats showed comparatively less amelioration of these markers.
Effects on hepatic lipid peroxidation
Similar to the observation in plasma, we found that the levels of the hepatic CD, LOOH, and MDA were also markedly increased — by 121.18%, 71.95%, and 97.06 %, respectively, in STZ-induced DC rats. Treatment with all the concentrations of D-MT resulted in amelioration of these markers of hepatic lipid peroxidation with a maximum restoration of 52.11%, 39.00%, and 46.27 % in CD, LOOH and MDA levels, respectively, in D-MT3 treated rats, when compared to corresponding DC rats. Treatment with D-BT also showed a reduction of 46.48%, 34.04%, and 42.62% in hepatic CD, LOOH and MDA, respectively, whereas D-GT showed slight amelioration in the levels of hepatic CD, LOOH and MDA- by only 9.85 %, 13.47%, and 22.38 %, respectively (Figure 2 b).
Effects on renal lipid peroxidation
Prolonged oxidative stress and elevated glucose level also lead to peroxidative and glycoxidative modifications of renal lipids. As shown in Figure 4 c, we also found that the levels of CD, LOOH, and MDA in the kidney homogenate w ere markedly increased by 123.33%, 76.74%, and 77.80 %, respectively, in STZ-induced DC rats. Treatment with D-MTs resulted in amelioration of these markers of renal lipid peroxidation with a maximum restoration of 44.78%, 39.47 %, and 49.50% in CD, LOOH and MDA levels, respectively, in D-MT3 treated rats, when compared to corresponding DC rats. The treatment of STZ-induced diabetic rats with the bioactive fraction (D-BT) of B. diffusa root methanolic extract also showed a reduction of 38.81%, 25.66%, and 38.31% in hepatic CD, LOOH and MDA, respectively. In contrast, D-GT treatment showed slight amelioration in the level of hepatic CD, LOOH and MDA by only 20.89 %, 13.16 %, and 18.22 %, respectively.
Boerhaavia diffusa root extract and bioactive fraction maintain redox status in STZ-induced rats
Effects on hepatic antioxidant enzymes
Persistent diabetes has been linked to enhanced oxidative stress due to the diminished activities of antioxidant enzymes. Similarly, the activities of hepatic antioxidant enzymes G-Px, SOD, CAT, GST, and GRed w ere markedly decreased in STZ-induced DC rats by 42.52 %, 32.42%, 33.78%, 49.95% and 9.03 %, respectively, when compared to the corresponding values of NC rats. The activities of G-Px, SOD, CAT, GST and GRed were restored in all the D-MT- treated rats with a maximum setback of 53.42%, 36.10 %, 23.87%, 64.11% and 8.90%, respectively, in D-MT3 treated rats, whereas D-BT treatment also showed a considerable increase of 26.03%, 19.74%, 17.20%, 41.33 % and 7.19 % in the hepatic G-Px, SOD, CAT, GST and G Red activities, respectively, when compared to DC rats. In contrast, the effect of D-GT treatment on the activities of hepatic antioxidant enzymes was not significantly different, when compared to corresponding untreated DC rats (Table 2).
Effects on renal antioxidant enzymes
In addition to hepatic antioxidant activities, STZ-induced diabetes can alter the functionality of renal antioxidant enzymes. We also reported that the activities of renal antioxidant enzymes GPx, SOD, CAT, GST and GRed were also markedly decreased in STZ-induced DC rats- by 30.20 %, 27.56 %, 46.28 %, 63.18 % and 10.96%, respectively, when compared to the corresponding values of NC rats. The activities of GPx, SOD, CAT, GST and GRed were restored in all the D-MT- treated rats with a maximum setback of 33.65 %, 27.23 %, 52.49 %, 132.22 % and 11.88%, respectively, in D-MT3 treated rats, whereas D-BT treatment led to a considerable increase of 16.35 %, 14.02 %, 40.65 %, 80.25 % and 11.66 % in the activities of renal GPx, SOD, CAT, GST and GRed, respectively, when compared to DC rats. Moreover, D-GT treatment led to an increase of 14.42%, 14.21%, 16.60%, 69.02% and 11.02% in the activities of GPx, SOD, CAT, GST and GRed, respectively, when compared to DC rats (Table 3).
B. diffusa root extract and its bioactive fraction normalize nephropathic architecture
The observations from our histopathological investigations of the kidney also indicated that the induction of experimental diabetes via STZ for 14 weeks resulted in the progression of diffused nodular glomerulosclerosis, along with thickening of the basement membrane. Furthermore, an increased number of mesangial cells and enlarged Bowman space were also seen in the kidney section s from DC rats. After 14 weeks of B. diffusa methanolic extract (D-MT3) and bioactive fraction (D-BT) treatment, the kidney sections from chronic diabetic rats showed significant regression in glomerulosclerosis and normalization of the basement membrane. In addition, histologically, the overall appearance was similar to that of normal kidney. Similarly, the intervention of glibenclamide to diabetic rats (D-GT) was associated with a substantial reduction in basement membrane thickening and glomerulosclerosis. However, unlike D-MT3, D-GT treatment induced interstitial inflammation of lymphocytes (Figure 5 ).
Discussion
Streptozotocin (STZ), a well-known diabetogenic agent, participates in DNA damage in pancreatic β-cells via xanthine oxidase (XOD) induced hydrogen peroxide, hydroxyl radicals, and toxic amounts of nitric oxide generation to cause diabetes and raise blood glucose28. In the same vein, in this study, we demonstrate a significant rise in the plasma glucose level in STZ-induced DC rats, which might be the consequence of STZ-triggered damage/disintegration to β-cells of the Islets of Langerhans. Moreover, the elevated plasma glucose level in diabetic rats was significantly reverted back by the treatment of B. diffusa extract (D- MTs) and a bioactive fraction (D-BT), up to the normal levels. These beneficial effects might be the result of its protective impact against STZ-induced pancreatic DNA damage, which further corroborates our previous study demonstrating the oxidative DNA damage preventive ability of D- MTs and D-BT11. Furthermore, treatment with the standard drug glibenclamide (D-GT) also decreased the plasma glucose level in STZ-induced rats by -71.25% (92.12 mg/dl), when compared to DC rats.
Moreover, we also reported significantly (a p<0.001) elevated levels of HbA1c (%) in DC rats (STZ-induced diabetic rats) at the end of our interventional study, possibly resulting from persistent hyperglycemia, leading to the reaction between excess blood glucose and hemoglobin via a non-enzymatic process to form glycosylated hemoglobin (HbA1c) ; the rate of the reaction is likely directly proportional to the blood glucose concentration29. These elevated HbA1c levels were significantly restored back up to normal level after treatment with different doses of D- MT and D-BT in diabetic rats. This significant restoration in plasma FBG and HbA1c levels was possibly achieved via the inhibition of α-amylase activity by the D-MTs and D-BT since they have been demonstrated to inhibit in vitro α-amylase activity11.
On the other hand, diabetes has been associated with altered lipoprotein levels (such as increased plasma TC, TG, LDL and VLDL, and diminished HDL) which make the lipoproteins more atherogenic and worsen the diabetic complications30. Similarly, the increase in blood glucose and HbA1c levels in the present in vivo study was also accompanied by altered lipid and lipoprotein levels in the plasma. These changes in the lipoprotein and TG levels were markedly ameliorated in D-MT- and D-BT - treated diabetic rats. These athero-protective effects of B. diffusa against STZ-induced diabetes may be attributed to the increased lipolysis of chylomicrons via lipoprotein lipase (LPL), as the activity of LPL is thought to be diminished by hyperglycemia31. In contrast, the activity of LPL is greatly reflected by the apolipoprotein-CIII (Apo-CIII), an indigenous inhibitor of LPL32. Therefore, it can be inferred from our study that the secondary metabolites from B. diffusa might decrease the level or activity of Apo-CIII, which in turn stimulate the activity of LPL and subsequent chylomicron lipolysis to counter balance the STZ-induced diabetic-atherosclerosis.
In addition to the lipoproteins, we also reported a marked increase in the level of FFAs and PLs in STZ-induced DC rats which may be correlated to the activity of hormone-sensitive lipase (HSL), an enzyme which regulates the release of FFA and glycerol from adipocyte lipid stores. Elevation of plasma FFA has been linked with insulin resistance, diabetes, and hyperlipidemia as the excess FFAs are converted into PLs and cholesterol in the liver33. These two substances, along with excess triglycerides formed in the liver, may be discharged into the blood in the form of lipoproteins33. The ameliorative effect of D-MTs and D-BT against elevated FFAs and PLs in diabetic rats in our study could be attributed to their ability to revert the potency of pancreatic β – cells to secrete enough insulin to inhibit the HSL-mediated lipolysis. The above-discussed findings are in accordance with recently published reports demonstrating the in vivo lipoprotein modulatory potential of various natural pharmacological agents,,.
Further more, to assess the molecular mechanisms underlying potent hypolipidemic potential of D-MTs and D-BT, we evaluated the activity of hepatic HMG-R, the rate-limiting enzyme of the cholesterol biosynthetic pathway and which controls hepatic cholesterol synthesis,. In this study, the in vivo HMG-R activity was found to be 2.13-fold higher than that of the NC rats. The plasma level of cholesterol in DC rats was significantly increased, whereas subsequent treatment with D-MTs and D-BT showed a marked inhibition of hepatic HMG-R activity in diabetic rats. This HMG-R inhibitory effect of D-MTs and D-BT is in accordance with our plasma levels of lipids and lipoproteins, as well as with previously published reports,.
Apart from the effects on blood sugar, plasma lipids and lipoprotein levels, STZ and alloxan are well-known to increase the level of thiobarbituric acid reactive substances (TBARS), and there is indirect evidence of intensified free radical production36. In addition, plasma from diabetic subjects also contain increased levels of CD, LOOH, TBARS, and/or MDA37. Persistent oxidative stress and elevated glucose levels in various tissues e.g., liver and kidney, also lead to the peroxidative and glycoxidative modification of lipids which ultimately leads to the formation of TBARS and various advanced glycation end products (AGEs),. We also evident a significant increase in the plasma, as well as hepatic and renal lipoperoxidative events, in DC rats that could be attributed to the enhanced rate of free radical generation under hyperglycemia and subsequent generation of CD, LOOH and MDA37. The damaging responses of diabetes-induced oxidative stress, in terms of lipid peroxidation, were markedly alleviated after the treatment of diabetic rats with D-MTs and D-BT. Thus, D-MTs and D-BT can mediate a decrease in the lipid peroxidation events which might occur due to their potent antioxidant activity11.
Chronic diabetes has been linked to enhanced oxidative stress due to the diminished activities of antioxidant enzymes (e.g., G-Px, SOD, CAT, GST, and GRed) in various tissues39. In our study, too, we found the activity of the above mentioned antioxidant enzymes in liver homogenates to be markedly reduced in DC rats at the end of the experiment. Their activities were potentially regained after treatment with methanolic extract (various concentrations) as well as the purified fraction of B. diffusa. These findings are well justified by a previous report demonstrating the protective role of Alhagi maurorum against experimental diabetes induced oxidative stress via restoring antioxidant activities of G-Px, SOD, and CAT40. In addition to the hepatic antioxidant activities, STZ-induced diabetes also alters the activities of renal antioxidant enzymes41. W e also evident diminished activities of G-Px, SOD, CAT, GST, and GRed in renal homogenates from DC rats. However, administration of D-MTs and D-BT was capable of markedly restoring the functionality of these antioxidant enzymes in diabetic rats. The restoration of these antioxidant enzyme activities is similar to that observed in other studies,.
Chronic hyperglycemia, dyslipidemia and lipid peroxidation are the major determinants of the development of secondary complications of diabetes. Patients with diabetic nephropathy display marked degenerative changes in these tissues. On the other hand, the most important factor influencing the occurrence of diabetic complications is the duration of disease42. Our histopathological investigations of kidney showed that persistent hyperglycemia in DC rats lead to marked abnormalities in the histoarchitecture of the kidney, e.g., progression of diffused nodular glomerulosclerosis, thickening of basement membrane, increased number of mesangial cells, and enlarged Bowman space. However, administration of D-MTs and D-BT markedly normalized the renal histoarchitecture in diabetic rats. Our results are consistent with previously published reports which have demonstrated that improved glycemic control is strongly associated with decreased severity of diabetic complications in both type-1 and type-2 diabetes43. To sum up the whole histological investigations, all the untoward features of nephropathy were noticeably regressed and normalized by D-MT3 and D-BT administration. These results are in agreement with a previous report which demonstrated that a combined therapy consisting of a hypoglycemic agent, Ocimum sanctum Linn, and α-tocopherol for 16 weeks completely reversed the hist o pathological changes in chronic diabetic rats44.
Conclusions
In conclusion, this report demonstrates the strong antidiabetic -cum hypolipidemic potential of the methanolic extract of B. diffusa root (D-MTs) together with its partially purified fraction (D-BT) via targeting FBG, HbA1c, plasma lipid and lipoprotein content, HMG-R activity, and systemic lipoperoxidative events. Our results also revealed strong antioxidant and antilipoperoxidative activities which may contribute to the protection against diabetic nephropathy (Figure 6 ). Although a detailed investigation is needed to elucidate the possible mechanisms involved, it is interesting to postulate that B. diffusa (Linn.), being a potent antioxidant, α-amylase inhibitor, and DNA damage preventive agent, may effectively protect beta-cells from total damage in order to amend STZ-induced diabetes and its associated complications.
ABBREVIATIONS
ASCVD: Atherosclerotic cardiovascular disease
CAT: Catalase
CD: Conjugated dienes
DC: Diabetic control
DPP-IV: Dipeptidyl peptidase-4
FBG: Fasting blood glucose
FFA: Free fatty acid
GPx: Glutathione peroxidase
Gred: Glutathione reductase
GST: Glutathione-S-transferase
HbA1c: Glycated hemoglobin
HDL: High density lipoprotein
HMG-R: HMG-CoA reductase
LDL: Low density lipoprotein
LDL-R: Low density lipoprotein-receptor
LOOH: Lipidhydroperoxide
MDA: Malondialdehyde
PLs: Phospholipids
SGLT-2: Sodium-glucose cotransporter
SOD: Superoxide dismutase
STZ: Streptozotocin
TC: Total cholesterol
TG: Triglycerides
VLDL: Very low density lipoprotein
XOD: Xanthine oxidase
Competing interests
The authors declare that they have no competing interests.
Acknowledgment
The authors like to acknowledge Council of Science and Technology, UP (UPCST) for financial assistance to this work. The author would also like to thank Deanship of Scientific Research at Majmaah University for their support and contribution to this study.
AUTHOR’S CONTRIBUTION
All authors equally contributed to this manuscript. Interventional hypothesis: M.S.K.; Animal handling, biochemical and histopathological analysis: F.A., S.S.A. & P.A.; Data analysis and statistical validations: F.A. & S.S.A.; Manuscript writing and figure preparation: M.S.K, F.A., S.S.A., P.A., D.A & B.M.A ; Answers of queries and Revision: D.A & B.M.A; English grammar editing and thorough revision & approval of the final manuscript: M.S.K. All authors approved to publish this manuscript.
References
-
Nabi
R.,
Alvi
S.S.,
Saeed
M.,
Ahmad
S.,
Khan
M.S.,
Glycation and HMG-CoA Reductase Inhibitors: Implication in Diabetes and Associated Complications. Curr Diabetes Rev.
2019;
15
(3)
:
213-23
.
View Article PubMed Google Scholar -
Ogurtsova
K.,
da Rocha Fernandes
J.D.,
Huang
Y.,
Linnenkamp
U.,
Guariguata
L.,
Cho
N.H.,
IDF Diabetes Atlas: global estimates for the prevalence of diabetes for 2015 and 2040. Diabetes Res Clin Pract.
2017;
128
:
40-50
.
View Article PubMed Google Scholar -
Kumar
A.,
Goel
M.K.,
Jain
R.B.,
Khanna
P.,
Chaudhary
V.,
India towards diabetes control: key issues. Australas Med J.
2013;
6
(10)
:
524-31
.
View Article PubMed Google Scholar -
Asmat
U.,
Abad
K.,
Ismail
K.,
Diabetes mellitus and oxidative stress-A concise review. Saudi Pharm J.
2016;
24
(5)
:
547-53
.
View Article PubMed Google Scholar -
Li
C.,
Miao
X.,
Li
F.,
Wang
S.,
Liu
Q.,
Wang
Y.,
Oxidative Stress-Related Mechanisms and Antioxidant Therapy in Diabetic Retinopathy. Oxid Med Cell Longev.
2017;
2017
:
9702820
.
View Article PubMed Google Scholar -
Singh
V.P.,
Bali
A.,
Singh
N.,
Jaggi
A.S.,
Advanced glycation end products and diabetic complications. Korean J Physiol Pharmacol.
2014;
18
(1)
:
1-14
.
View Article PubMed Google Scholar -
Carrizzo
A.,
Izzo
C.,
Oliveti
M.,
Alfano
A.,
Virtuoso
N.,
Capunzo
M.,
The Main Determinants of Diabetes Mellitus Vascular Complications: Endothelial Dysfunction and Platelet Hyperaggregation. Int J Mol Sci.
2018;
19
(10)
:
2968
.
View Article PubMed Google Scholar -
Chaudhury
A.,
Duvoor
C.,
Reddy Dendi
V.S.,
Kraleti
S.,
Chada
A.,
Ravilla
R.,
Clinical Review of Antidiabetic Drugs: Implications for Type 2 Diabetes Mellitus Management. Front Endocrinol (Lausanne).
2017;
8
(6)
:
6
.
View Article PubMed Google Scholar -
Patel
D.K.,
Prasad
S.K.,
Kumar
R.,
Hemalatha
S.,
An overview on antidiabetic medicinal plants having insulin mimetic property. Asian Pac J Trop Biomed.
2012;
2
(4)
:
320-30
.
View Article PubMed Google Scholar -
Mishra
S.,
Aeri
V.,
Gaur
P.K.,
Jachak
S.M.,
Phytochemical, therapeutic, and ethnopharmacological overview for a traditionally important herb: boerhavia diffusa Linn. BioMed Res Int.
2014;
2014
:
808302
.
View Article PubMed Google Scholar -
Akhter
F.,
Hashim
A.,
Khan
M.S.,
Ahmad
S.,
Iqbal
D.,
Srivastava
A.K.,
Antioxidant, α-amylase inhibitory and oxidative DNA damage protective property of Boerhaavia diffusa (Linn.) root. S Afr J Bot.
2013;
88
:
265-72
.
View Article Google Scholar -
Deeds
M.C.,
Anderson
J.M.,
Armstrong
A.S.,
Gastineau
D.A.,
Hiddinga
H.J.,
Jahangir
A.,
Single dose streptozotocin-induced diabetes: considerations for study design in islet transplantation models. Lab Anim.
2011;
45
(3)
:
131-40
.
View Article PubMed Google Scholar -
Adefokun
D.I.,
Iwalewa
E.O.,
Omisore
N.O.,
Obuotor
E.,
Idowu
I.J.,
The Antimalarial Effect and Mechanism of Action of Methanolic Root Extract of Boerhaavia diffusa in Mice. Br J Pharm Res.
2015;
8
(2)
:
1-14
.
View Article Google Scholar -
Wieland
H.,
Seidel
D.,
A simple specific method for precipitation of low density lipoproteins. J Lipid Res.
1983;
24
(7)
:
904-9
.
PubMed Google Scholar -
Patsch
W.,
Brown
S.A.,
Morrisett
J.D.,
Gotto
A.M.,
Patsch
J.R.,
A dual-precipitation method evaluated for measurement of cholesterol in high-density lipoprotein subfractions HDL2 and HDL3 in human plasma. Clin Chem.
1989;
35
(2)
:
265-70
.
PubMed Google Scholar -
Folch
J.,
Lees
M.,
Sloane Stanley
G.H.,
A simple method for the isolation and purification of total lipides from animal tissues. J Biol Chem.
1957;
226
(1)
:
497-509
.
PubMed Google Scholar -
Khawaja
O.,
Maziarz
M.,
Biggs
M.L.,
Longstreth
W.T.,
Ix
J.H.,
Kizer
J.R.,
Plasma free fatty acids and risk of stroke in the Cardiovascular Health Study. Int J Stroke.
2014;
9
(7)
:
917-20
.
View Article PubMed Google Scholar -
Zou
W.,
Total Phospholipids and Phospholipid Subclasses DeterminationProto Excha 2011.
View Article Google Scholar -
Sultan Alvi
S.,
Ansari
I.A.,
Khan
I.,
Iqbal
J.,
Khan
M.S.,
Potential role of lycopene in targeting proprotein convertase subtilisin/kexin type-9 to combat hypercholesterolemia. Free Radic Biol Med.
2017;
108
:
394-403
.
View Article PubMed Google Scholar -
Beuje
J.A.,
Aust
S.D.,
Methods in enzymologyAcad Press New York 1978.
Google Scholar -
Nourooz-Zadeh
J.,
Tajaddini-Sarmadi
J.,
Ling
K.L.,
Wolff
S.P.,
Low-density lipoprotein is the major carrier of lipid hydroperoxides in plasma. Relevance to determination of total plasma lipid hydroperoxide concentrations. Biochem J.
1996;
313
(Pt 3)
:
781-6
.
View Article PubMed Google Scholar -
Ohkawa
H.,
Ohishi
N.,
Yagi
K.,
Assay for lipid peroxides in animal tissues by thiobarbituric acid reaction. Anal Biochem.
1979;
95
(2)
:
351-8
.
View Article PubMed Google Scholar -
Sinha
A.K.,
Colorimetric assay of catalase. Anal Biochem.
1972;
47
(2)
:
389-94
.
View Article PubMed Google Scholar -
Kakkar
P.,
Das
B.,
Viswanathan
P.N.,
A modified spectrophotometric assay of superoxide dismutase. Indian J Biochem Biophys.
1984;
21
(2)
:
130-2
.
PubMed Google Scholar -
Hafeman
D.G.,
Sunde
R.A.,
Hoekstra
W.G.,
Effect of dietary selenium on erythrocyte and liver glutathione peroxidase in the rat. J Nutr.
1974;
104
(5)
:
580-7
.
View Article PubMed Google Scholar -
Carlberg
I.,
Mannervik
E.B.,
Glutathione level in rat brain. J Biol Chem.
1975;
250
:
4475-80
.
-
Habig
W.H.,
Pabst
M.J.,
Jakoby
W.B.,
Glutathione-S-transferases in mercapturic acid formation. J Biol Chem.
1974;
249
:
7130-9
.
PubMed Google Scholar -
Szkudelski
T.,
The mechanism of alloxan and streptozotocin action in B cells of the rat pancreas. Physiol Res.
2001;
50
(6)
:
537-46
.
PubMed Google Scholar -
Beltran Del Rio
M.,
Tiwari
M.,
Amodu
L.I.,
Cagliani
J.,
Rodriguez Rilo
H.L.,
Glycated Hemoglobin, Plasma Glucose, and Erythrocyte Aging. J Diabetes Sci Technol.
2016;
10
(6)
:
1303-7
.
View Article PubMed Google Scholar -
Vergès
B.,
Pathophysiology of diabetic dyslipidaemia: where are we?. Diabetologia.
2015;
58
(5)
:
886-99
.
View Article PubMed Google Scholar -
Cianflone
K.,
Paglialunga
S.,
Roy
C.,
Intestinally derived lipids: metabolic regulation and consequences\textemdashan overview. Atheroscler Suppl.
2008;
9
(2)
:
63-8
.
View Article PubMed Google Scholar -
Alvi
S.S.,
Ansari
I.A.,
Ahmad
M.K.,
Iqbal
J.,
Khan
M.S.,
Lycopene amends LPS induced oxidative stress and hypertriglyceridemia via modulating PCSK-9 expression and Apo-CIII mediated lipoprotein lipase activity. Biomed Pharmacother.
2017;
96
:
1082-93
.
View Article PubMed Google Scholar -
Claus
T.H.,
Lowe
D.B.,
Liang
Y.,
Salhanick
A.I.,
Lubeski
C.K.,
Yang
L.,
Specific inhibition of hormone-sensitive lipase improves lipid profile while reducing plasma glucose. J Pharmacol Exp Ther.
2005;
315
(3)
:
1396-402
.
View Article PubMed Google Scholar -
Alvi
S.S.,
Ansari
I.A.,
Khan
M.S.,
Pleiotropic role of lycopene in protecting various risk factors mediated atherosclerosis. Ann Phytomed..
2015;
4
(1)
:
54-60
.
-
Alvi
S.S.,
Iqbal
D.,
Ahmad
S.,
Khan
M.S.,
Molecular rationale delineating the role of lycopene as a potent HMG-CoA reductase inhibitor: in vitro and in silico study. Nat Prod Res.
2016;
30
(18)
:
2111-4
.
View Article PubMed Google Scholar -
Maritim
A.C.,
Sanders
R.A.,
Watkins
J.B.,
Diabetes, oxidative stress, and antioxidants: a review. J Biochem Mol Toxicol.
2003;
17
(1)
:
24-38
.
View Article PubMed Google Scholar -
Martín-Gallán
P.,
Carrascosa
A.,
Gussinyé
M.,
Domínguez
C.,
Biomarkers of diabetes-associated oxidative stress and antioxidant status in young diabetic patients with or without subclinical complications. Free Radic Biol Med.
2003;
34
(12)
:
1563-74
.
View Article PubMed Google Scholar -
Nabi
R.,
Alvi
S.S.,
Khan
R.H.,
Ahmad
S.,
Ahmad
S.,
Khan
M.S.,
Antiglycation study of HMG-R inhibitors and tocotrienol against glycated BSA and LDL: A comparative study. Int J Biol Macromol.
2018;
116
:
983-92
.
View Article PubMed Google Scholar -
Sindhu
R.K.,
Koo
J.R.,
Roberts
C.K.,
Vaziri
N.D.,
Dysregulation of hepatic superoxide dismutase, catalase and glutathione peroxidase in diabetes: response to insulin and antioxidant therapies. Clin Exp Hypertens.
2004;
26
(1)
:
43-53
.
View Article PubMed Google Scholar -
Sheweita
S.A.,
Mashaly
S.,
Newairy
A.A.,
Abdou
H.M.,
Eweda
S.M.,
Changes in Oxidative Stress and Antioxidant Enzyme Activities in Streptozotocin-Induced Diabetes Mellitus in Rats: Role of Alhagi maurorum Extracts. Oxid Med Cell Longev.
2016;
2016
:
5264064
.
View Article PubMed Google Scholar -
Sadi
G.,
Eryilmaz
N.,
Tütüncüoğlu
E.,
Cingir
\cS.,
Güray
T.,
Changes in expression profiles of antioxidant enzymes in diabetic rat kidneys. Diabetes Metab Res Rev.
2012;
28
(3)
:
228-35
.
View Article PubMed Google Scholar -
Aldebasi
Y.H.,
Mohieldein
A.H.,
Almansour
Y.S.,
Almutairi
B.L.,
Dyslipidemia and lipid peroxidation of Saudi type 2 diabetics with proliferative retinopathy. Saudi Med J.
2013;
34
(6)
:
616-22
.
PubMed Google Scholar -
Fioretto
P.,
Bruseghin
M.,
Berto
I.,
Gallina
P.,
Manzato
E.,
Mussap
M.,
Renal protection in diabetes: role of glycemic control. J Am Soc Nephrol.
2006;
17
(4)
:
86-9
.
View Article PubMed Google Scholar -
Halim
E.M.,
Mukhopadhyay
A.K.,
Effect ofOcimum sanctum (Tulsi) and vitamin E on biochemical parameters and retinopathy in streptozotocin induced diabetic rats. Indian J Clin Biochem.
2006;
21
(2)
:
181-8
.
View Article PubMed Google Scholar
Comments
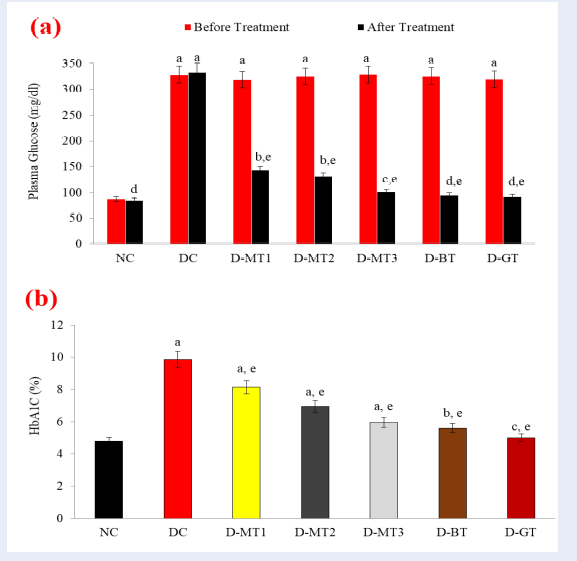
Downloads
Article Details
Volume & Issue : Vol 6 No 7 (2019)
Page No.: 3293-3306
Published on: 2019-07-13
Citations
Copyrights & License

This work is licensed under a Creative Commons Attribution 4.0 International License.
Search Panel
Pubmed
Google Scholar
Pubmed
Google Scholar
Pubmed
Google Scholar
Pubmed
Google Scholar
Pubmed
Google Scholar
Pubmed
Search for this article in:
Google Scholar
Researchgate
- HTML viewed - 9967 times
- Download PDF downloaded - 1632 times
- View Article downloaded - 0 times