Abstract
This article was retracted by author on 29 Feb 2022
Introduction: The increased presence of radiation and toxins in the atmosphere has given rise to fuel cells and nanoparticle technology with the ability to catalyze reactions at favorable energy levels. The purpose of this experiment was to determine the lasting effects of a synthesized catalyst on a model organism, Caenorhabditis (C.) elegans.
Methods: Copper sulfate was tested alongside copper(I) oxide (i.e. Cu2O) to dissociate the copper composition effects from those of the nanoparticles themselves. The prospect of testing both defecation rate and size differences allowed for C. elegans to be utilized due to their low maintenance costs, mapped neuronal pathways, and short-generation times.
Results: The results indicated significant toxicity effects in wild-type worms as witnessed by the decreases in nematode defecation rate and length by copper sulfate, with similar results in SMF-1 and PCS-1 mutants by Cu2O catalysts in cubic synthesized form.
Conclusion: These outcomes reinforce the known effects of metal oxides on pollutants and highlight the need for further testing with additional variables such as varying pH and temperature.
Introduction
The predicament created by the rise in manufacturing, attributed to industrial growth after the 1850s, has slowly eroded the once “pure” atmosphere that was present before the age of human emissions. Although billions of dollars of research ensue every year in search of catalytic converters, little attention is given regarding the effective application and installation of these converters to solve unfavorable energy processes.
A potential promising solution to this issue includes the use of complete oxidative catalyst agents, capable of absorbing organic pollutants while leaving a limited resulting environmental trace1. This particular study employs cubic-shaped particles for testing nematodes under 48-h time intervals with copper(I) oxide (i.e. Cu2O) nanoparticles and copper sulfate, and for quantifying each facet’s potential bodily effects if implemented in toxin remediation2. Wild-type, as well as SMF-1 and phytochelatin synthase (PCS-1) gene-lacking mutant Caenorhabditis (C.) elegans, were used to quantify the toxicity effects on bodily size and defecation rate. It was predicted that all three genotypes would have insignificant differences in both assays when treated with water. It was also expected that SMF-1 and PCS-1 mutants would have decreased body size and defecation rate due to the respective transport and metal tolerant roles assigned to each missing gene.
Materials — Methods
Synthesis
All reagents were used as received. In a typical procedure, all steps were carried out as referenced in Sui et al.3.
Characterization
The plugs were inserted into an FEI XL-30 Field Emission ESEM/SEM (scanning electron microscope) (Field Electron and Ion Company, Hillsboro, OR) with energy-dispersive X-ray spectroscopy (EDS) capability, as described by Murphy et al.4. The field emission gun tip then initiated a narrow electron beam, allowing for image analysis (as documented in Figure 1), as well as atomic mass percentage breakdown5.
C. elegans worm culture and treatment with nanoparticles
All C. elegans strains were cultured on a growth medium with Escherichia (E.) coli strain OP50 as the food source. SMF-1 and PCS-1 mutants were included in each sampling preparation, as described by Mashock6. Formulation of dried nanoparticles into viable solution involved transferring 20 mg into a 1.5 mL microcentrifuge tube and dissolving in 95% ethanol at 200 mg/ml. This was followed by bath sonication for 5 min to disperse particles with subsequent vortexing for 5 s. Next, 10 μL of particles was then immediately transferred into a microcentrifuge tube, followed by addition of 990 μL of sterile deionized (DI) water. This mixture was then vortexed for 30 s. The copper sulfate solution was prepared using a stock 0.1 M vial with a 1:4 stock to DI water ratio. Thus, 1 mL in total was maintained across all organized sample tubes. The water control consisted of 990 μL of DI water mixed with 10 μL of 95% ethanol. Finally, 200 μL of the nanoparticle, copper sulfate, and water solutions were pipetted on top of the OP50 bacteria growth medium in each condition’s respective plates. These were then allowed to incubate for 48 hours at 20°C.
Defecation and size analysis
Defecation was assayed using a Nikon Light Microscope with an Amscope Cold-Light Source Lamp (Amscope, Irvine, CA). The worms were examined for 5-min time intervals using the Apple stopwatch app to calculate the time between defecations. Only adult C. elegans were chosen for defecation analysis to ensure the proper biological effect7. Three worms were observed for each condition (wild type, PCS-1, and SMF-1) during each experimental period. This study was performed over 6 observation periods. Conditions that were unable to be surveyed due to widespread nematode death were noted for statistical reference. Size analysis was carried out after the equivalent incubation period via a Leica M165FC stereomicroscope (Leica Microsystems, Wetzlar, Germany) using a PLAN APO 1.0x/0.5 NA objective, as performed by Munro et al.8. Similarly, FIJI software (National Institutes of Health, USA) was utilized to measure worm lengths from image progressions. Approximately 18 individuals per treatment condition (wild-type, PCS-1, and SMF-1) were measured for defecation and size analysis. Worms were considered dead if no movement was observed after a period of 60 seconds or 15 seconds after applying mechanical stimulation.
Statistical Analysis
Data analysis from the image analysis and defecation rate observations was conducted using JASP statistical software (The JASP Team, Amsterdam, The Netherlands). A p-value of ≤ 0.05 was considered significant. Conditions with significant p-values are indicated in their respective figure legends. All statistical tests performed were corrected to account for multiple comparisons.
Results
Defecation Results
Statistical data taken from the four defecation analysis trial periods yielded multiple significant p-value comparisons across both treatments and C. elegans conditions. SMF-1 mutants showed decreased defecation rates compared to wild-type worms when treated with nanoparticles (Figure 2). SMF-1 mutants treated with both copper sulfate and nanoparticles showed significantly more time between defecations in comparison to those treated with water (Figure 3). Figure 4 indicates that wild-type nematodes treated with copper sulfate exhibited decreased defecation rates compared to those treated with water.
Length Results
Wild type nematodes treated with copper sulfate were found to be smaller than their water-treated counterparts with notably precise distribution points (Figure 5). PCS-1 mutants treated with both copper sulfate and nanoparticles were significantly shorter in length than those treated with water (Figure 6). Of those treated with nanoparticles, SMF-1 mutants were significantly larger than their PCS-1 and wild-type counterparts. Concurrently, PCS-1 mutants were significantly shorter compared to wild-type nematodes, averaging around 0.3 mm in length less than wild type C. elegans. The same relationships were observed in the copper sulfate-induced group; PCS-1 mutants were remarkably smaller than both the wild-type and SMF-1 mutants, with SMF-1 mutants being the largest of the three conditions treated with copper sulfate (Figure 8). In contrast, the SMF-1 mutant nematodes had no significant size differences in comparison to the wild-type treatments.
Discussion
For the defecation analysis trial periods, insufficient data was generated due to the broad worm death observed in worms exposed to copper sulfate for the 48-h period. Perhaps the most significant finding of the defecation section is that wild-type worms treated with nanoparticles showed no significant difference in defecation rate when compared to those treated with water. While wild-type nematodes treated with copper sulfate demonstrated lower defecation rates compared to those treated with water, this may be explained by the wide distribution of data points seen in Figure 4. The sample size disparity shown between the two conditions was due, in part, to worm death in wild type C. elegans treated with copper sulfate on multiple trial sessions.
Length assays were carried out on fewer occasions than defecation analyses due to the premature cell death mentioned above. However, this allowed for equivalent sample sizes across each condition for statistical representation. A very dispersed sample distribution can be seen in the wild type nematodes in Figure 7 treated with nanoparticles, possibly explained by the relatively large size differences. It can be concluded from Figure 6 that the PCS-1 mutants were hypersensitive to nanoparticles and even more sensitive to copper sulfate in terms of length. These results can be interpreted reasonably due to the equivalent control group sample sizes for each worm type.
Conclusions
The potential effects of implementing copper oxide nanoparticles in toxin remediation were examined through defecation rate and size analysis of a soil-inhabiting model organism, C. elegans. A very insightful finding of this study was the decreased defecation rate and length of wild-type nematodes when treated with copper sulfate, indicative of copper ion-induced toxicity. Similarly, the hypersensitive shrinking observed in PCS-1 mutants exposed to copper sulfate and nanoparticles reflects the metal-tolerant functional role of PCS-1 gene expression9. The steady length of SMF-1 mutants in the presence of both copper sulfate and nanoparticles relative to the wild-type levels can be explained by the metal transport dysfunction observed when the SMF gene is not expressed. This causes reduced metal transport to neuron pathways and interior organs, lessening the degenerative effect of metal ions on size for short time intervals. Perhaps most central to this study was the lack of significant nanoparticle-induced effects on defecation rate and size of wild-type worms. While these are positive result in the pursuit of environmental pollutant catalysts, these findings also highlight the need for additional testing under longer incubation time intervals, other metal types, and ulterior application-affected organisms10.
Abbreviations
APO: Apochromatic
ESEM: Environmental scanning electron microscope
NA: numerical aperture
Acknowledgments
The author would like to thank the Howard Hughes Medical Institute for providing laboratory space and materials for this research. The author acknowledges the Knecht Group and Collins Lab for resources used throughout the experiment. This research also used laboratories provided by the University of Miami Department of Biology and utilized SEM analysis from the University of Miami Center for Advanced Microscopy (UMCAM).
Author’s contributions
Author read and approved the final manuscript.
Funding
None.
Availability of data and materials
Data and materials used and/or analyzed during the current study are available from the corresponding author on reasonable request.
Ethics approval and consent to participate
Not applicable.
Consent for publication
Not applicable.
Competing interests
The author declares that they have no competing interests.
References
-
Ben-Moshe
T.,
Ishai
D.,
Brian
B.,
Oxidation of organic pollutants in aqueous solutions by nanosized copper oxide catalysts. Applied Catalysis. B, Environmental.
2009;
85
(3-4)
:
207-211
.
View Article Google Scholar -
Martinez-Finley
E.J.,
Michael
A.,
Revelations from the Nematode Caenorhabditis elegans on the Complex Interplay of Metal Toxicological Mechanisms. Journal of Toxicology.
2011;
2011
:
895236
.
View Article PubMed Google Scholar -
Sui
Y.,
Low Temperature Synthesis of Cu2O Crystals: Shape Evolution and Growth Mechanism. Crystal Growth & Design.
2010;
10
(1)
:
99-108
.
View Article Google Scholar -
Murphy
C.J.,
Gold Nanoparticles in Biology: Beyond Toxicity to Cellular Imaging. Accounts of Chemical Research.
2008;
41
(12)
:
1721-1730
.
View Article PubMed Google Scholar -
Nguyen
M.A.,
Direct Synthetic Control over the Size, Composition, and Photocatalytic Activity of Octahedral Copper Oxide Materials: Correlation Between Surface Structure and Catalytic Functionality. ACS Applied Materials & Interfaces.
2015;
7
(24)
:
13238-13250
.
View Article PubMed Google Scholar -
Mashock
M.J.,
Copper Oxide Nanoparticles Impact Several Toxicological Endpoints and Cause Neurodegeneration in Caenorhabditis elegans. PloS One.
2016;
11
(12)
:
E0167613
.
View Article PubMed Google Scholar -
Zhang
Y.,
Selection of Reliable Reference Genes in Caenorhabditis elegans for Analysis of Nanotoxicity. PloS One.
2012;
7
(3)
:
E31849
.
View Article PubMed Google Scholar -
Munro
C.J.,
Identification of toxicity effects of Cu2O materials on C. elegans as a function of environmental ionic composition. Environmental Science: Nano.
2020;
7
(2)
:
645-655
.
View Article PubMed Google Scholar -
Kühnlenz
T.,
Expression of Caenorhabditis elegans PCS in the AtPCS1‐deficient Arabidopsis thaliana cad1‐3 mutant separates the metal tolerance and non‐host resistance functions of phytochelatin synthases. Plant, Cell & Environment.
2015;
38
(11)
:
2239-2247
.
View Article PubMed Google Scholar -
Settivari
R.,
LeVora
J.,
Nass
R.,
The Divalent Metal Transporter Homologues SMF-1/2 Mediate Dopamine Neuron Sensitivity in Caenorhabditis elegans Models of Manganism and Parkinson Disease. Journal of Biological Chemistry.
2009;
284
(51)
:
35758-35768
.
View Article PubMed Google Scholar
Comments
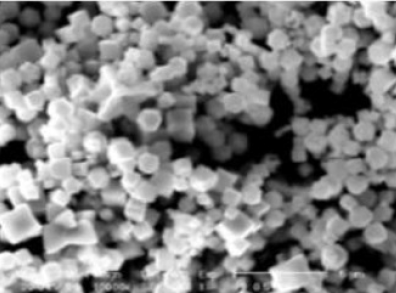
Downloads
Article Details
Volume & Issue : Vol 7 No 10 (2020)
Page No.: 4045-4051
Published on: 2020-10-31
Citations
Copyrights & License

This work is licensed under a Creative Commons Attribution 4.0 International License.
Search Panel
- HTML viewed - 4984 times
- Download PDF downloaded - 1393 times
- View Article downloaded - 0 times