Abstract
Triclosan (TCS) is present in toothpaste and other cosmetic products as an antibacterial and anti-fungal agent. This manuscript highlights that TCS is a potential oxidative stress-causing agent, an estrogenic, mutagenic, cancer-causing agent, and genotoxic agent present in cosmetic products. This study also summarizes the therapeutic approach to overcome all of the harmful effects. It is a popular current topic, and a new research study is needed to find a new alternative as an antibacterial and anti-fungal agent instead of TCS. TCS causes oxidative stress when the dynamic balance in synthesizing and removing reactive oxygen species (ROS) within typical physiological circumstances is disturbed. The antioxidant defence system includes both enzymatic and non-enzymatic antioxidants produced by the organism to tackle the harmful effects of ROS. TCSs have estrogenic, proliferative, and apoptotic properties due to research on cell fate. The mutagenic potential of TCS has been examined using in vitro and in vivo research in prokaryotic, eukaryotic systems, and mammalian cells. It also induces carcinogenic, estrogenic, and mutagenic effects. TCS's therapeutic effects, especially against inflammatory skin conditions, have been demonstrated by many materials gathered from in vitro and in vivo experiments. The new findings suggest that TCS, a commonly used cosmetic product, may cause cancer, as shown by animal and human models and clinical trials. TCS is not effectively regulated, as evidenced by its presence in various environmental media, human bodies, and animals. Its irresponsible usage and disposal may endanger humans and wildlife. TCS has been found to damage a wide variety of cells in cell-based investigations. TCS's exact function in the environmental selection of antibiotic and multidrug resistance genes is still unclear. Comprehensive evaluations of these domains, especially to derive serious human health risk inferences from TCS outcomes, may help future research and legislation to better serve the public's health.
INTRODUCTION
Toothpaste is the most widely used cosmetic product for teeth protection in modern societies. There are many chemicals and natural products that act as an antibacterial, antifungal, and protectant for teeth. However, its constituents include Triclosan (TCS), an antibacterial and antifungal agent to protect teeth from deleterious effects. TCS is also used in other cosmetic products such as soaps, detergents, toys, and surgical cleaning treatments1, 2. TCS is widely regarded as a broad-spectrum biocide that targets bacterial membranes, and cellular resistance is uncommon if TCS has a unique mechanism of action. Since the TCS inhibits Escherichia coli (E. coli) enoyl reductase (FabI), the bisphenol has also been found to inhibit the enzyme from different bacteria such as Pseudomonas aeruginosa (P. aeruginosa) and Staphylococcus aureus (S. aureus). TCS's inhibitory action on enoyl reductase was discovered, FabK in P. aeruginosa and Staphylococcus pneumoniae (S. pneumoniae), fabL in Bacillus subtilis (B. subtilis), InhA in Mycobacterium smegmatis (M. smegmatis), and Mycobacterium tuberculosis (M. tuberculosis) have all been identified3, 4. FabK and FabL are TCS resistant, whereas InhA is not. Similar inhibition of TCS fatty acid production was seen in higher lifeforms, including Plasmodium falciparum P. falciparum), which causes malaria, and Toxoplasma gondii (T. gondii)3, 5. TCS is easily photodegraded in the environment, despite its high chemical volatility and resistance to high and low pH. In the laboratory, they found eight photochemical by-products. Under various irradiation wavelengths, Latch et al.,6 reported TCS photoconversion to 2,8-dichlorodibenzo-p-dioxin (2,8-DCDD) with up to 12% output at pH > 8. The yield of 2,8-DCDD under laboratory settings (pure water) and river water treated with TCS was compared7. According to findings comparable to lab and field settings, TCS can be converted to 2,8-DCDD in sunlight irradiated water sources. According to Son et al.,8, radicals that enhance transitional dioxin degradation control TCS breakdown by titanium dioxide photocatalysis. Oxidative damage to lipid membrane, protein, and nucleic acid may result from insufficient reactive oxygen species (ROS) scavenging9. Reduced glutathione (GSH), catalase (CAT), glutathione peroxidase (GPx), superoxide dismutase (SOD), and glutathione reductase (GR) are examples of enzymatic and non-enzymatic antioxidants that defend against the harmful consequences of ROS. The peroxidation of cell membrane lipids may occur when organisms are exposed to ROS-producing contaminants. Malondialdehyde (MDA) is a biomarker for assessing cell membrane destruction that is produced as a result of membrane lipid peroxidation (LPO)10. After subchronic TCS treatment, the antioxidant mechanism of the liver may be unable to remove ROS produced by TCS, which might explain the elevated MDA levels. Antioxidant compounds are free radical scavengers because they prevent or delay-free the radical oxidation of substrates, resulting in significant LPO protection in biological systems11. Phenolic and polyphenolic chemicals are the most common natural antioxidants found in plants, foods, and beverages. The total antioxidant capacity (TAC) was calculated by reducing Mo (VI) to Mo (V) in the extract and forming a green phosphate/Mo(V) complex at an acid pH12. It evaluates overall antioxidant capacity, including both water and fat-soluble antioxidants. It has been suggested that electron donation is related to antioxidant activity, which indicates a decrease in bioactive chemical potency. Antioxidants may operate as reductants, and the deactivation of oxidants by reductants can be thought of as redox reactions in which one reaction species is reduced while the other is oxidized. Antioxidants have been found in the root of Anchomanes difformis (A. difformis), which may play a role in the plant's medicinal action9, 13. On the other hand, TCS interacts with Agrobacterium tumefaciens (A. tumefaciens) AcrR, causing structural changes and inhibiting adhesion to the AcrA promoter. TCS membrane association in human erythrocytes was also studied to see the underlying mechanisms of electrolytes channels. TCS induced K+ outflow and haemolysis, implying membrane breakdown while preventing hypotonic lysis, which might be mediated by membrane enlargement. TCS significantly decreased the enzymatic efficiency of membrane-bound K+, Na+, Mg2+-ATPase14. TCS affects erythrocyte osmoregulation, promotes membrane instability, and inhibits monovalent ion movement. According to studies on its impact on cell fate, TCS possesses estrogenic, proliferative, and apoptotic characteristics14. The cell cycle and death genes and proteins are especially susceptible to TCS regulation. TCS is cytotoxic to epithelial cells and gingival fibroblasts, suggesting that this may be a new apoptosis inducer in these cells. A research team used BG-1 ovarian cancer cells in various in vivo and in vitro studies to see how TCS affected the growth and proliferation of these cells. TCS promotes cell proliferation and raises the protein levels and expression of the cyclin D1 gene while decreasing the expression and protein levels of the p21 and Bax genes. After exposure to TCS, inflammation started via Toll-like receptor-4 (TLR-4)-mediated signalling mechanism through gut microbiota. Mustafa et al.,14 discovered TCS targets in human gingival fibroblasts, including interleukin-1β (IL-1β), interferon-γ (IFN-γ), prostaglandin E synthase-1 (PGES-1), and major histocompatibility complex class II (MHC-II). Investigations into the subcellular localization of TCS have shown that nucleus accumulation takes priority over cytosolic accumulation14. Due to the greater initial absorption of cytoplasmic TCS, a substantial portion of cytosolic TCS was removed after each wash, whereas nuclear TCS was retained. This may explain why TCS has different inflammatory signals. However, it has been known to be a potential endocrine disruptor due to binding to androgen and oestrogen receptors15, 16, 17. In terms of TCS's androgenic properties, it was found that TCS inhibits testosterone-dependent transcription while increasing androgen-dependent transcription. TCS stimulates or inhibits a variety of signaling pathways, according to evidence on xenobiotic responses to TCS18. Although considerable progress has been made in TCS signaling, much remains unknown regarding TCS's modulatory effects on cellular physiology, especially in human-based systems. In the administration to the whole blood leukocytes, TCS inhibited TLR signaling. It leads to the downregulation of several signaling mediators, most notably NF-B inducing kinase (Nik) and C-jun. It explains the cells' cumulative lowered inflammatory response lipopolysaccharides (LPS). TCS's endocrine-disrupting properties, particularly its estrogenicity, has grabbed researchers' attention. TCS promoted proliferation in BG-1 ovarian cancer cells through the oestrogen-receptor (ER), as shown by Kim J-Y et al.19. TCS's mutagenic potential has been studied in prokaryotic and eukaryotic systems using in vitro and in vivo investigations. It looks for frame shift mutations, point mutations, clastogenic events, and recombination events20.
TCS did not induce gene mutations within these systems, as shown by the negative findings of these reverse mutation studies in vitro and the host. In vitro studies of gene mutations of mammalian cells in mouse lymphoma, L5178Y cells with and without metabolic stimulation show that the potential for TCS to induce mutations in the thymidine kinase (TK) domain was examined21. Studies have proven that TCS exhibits anti-androgenic and anti-estrogenic properties, depending on species, tissues, and cell types22. A study in China had shown that prenatal TCS exposure in pregnant women led to higher cord testosterone levels in infants23. Another study revealed that TCS was higher in urine samples than 75% in all tested samples24. Here, we summarized findings from recent studies, which suggest tumorigenic effects of TCS. It has been reported in many studies that extensive usage of TCS can have cancerogenic potential. Recently, TCS has been demonstrated to cause colon-associated inflammation, which ultimately leads to colitis-mediated colon tumours. TCS is also associated with higher colitis symptoms, ultimately leading to colitis-mediated colon cancer25.
TCS's effects on breast cancer cells in vitro may be influenced by the concentration and other factors such as oestradiol (natural oestrogen). A smaller head circumference at birth, early breast development, antibiotic resistance, and hypersensitivity are all possible health consequences. TCS's presence in milk suggests that it has travelled through the human breast, raising concerns about its involvement in breast cancer development. A commonly used antimicrobial preservative in personal care products, TCS is an endocrine disruptor in hormone-dependent tissues. TCS increases vascular endothelial growth factor (VEGF) production, a chemical that promotes tumour growth via human prostate cancer stromal cells26. TCS's first specific action method in prokaryotic cells was identified just 20 years ago when it was revealed that TCS suppressed fatty acid synthesis (FAS) in E. coli 27. By replicating its native substrate in vivo, TCS permanently blocked the FAS enzyme enoyl-acyl carrier protein (Enoyl-ACP) reductase28. TCS resistance was also shown by a mutant or overexpressed ACP expressed by fabI in bacteria.
As a consequence of these investigations, ACP was identified as an intracellular TCS target. Multiple studies supporting fatty acid formulation suppression as a novel strategy for chemotherapy have been inspired by the effectiveness of cerulenin, a mycotoxin that inhibits FAS in vivo. FAS appears and acts differently in normal and malignant tissues, with the latter having a higher therapeutic index28. Because of its long history of human usage and broad prevalence in consumer products, as well as promising in vivo results, TCS is a suitable choice for chemotherapy. TCS may enhance the proliferation of BG-1 ovarian cancer cells by modulating the expression of cell cycle and cell death genes via ER-based mechanisms, according to in vitro research29. TCS, like E2, was shown to have estrogenic properties via altering the appearance of protein kinase B (PKB), mitogen-activated protein kinase (MAPK), phosphorylated insulin receptor substrate-1 (pIRS-1), and extracellular signal-regulated kinase (ERK) proteins30. It also inhibited the protein synthesis of pIRS-1, PKB, MAPK, and ERK, which were all increased by E2 or TCS, and therefore had an antiestrogenic effect. As shown through its prevalence in various environmental media, human bodies, and animals, TCS is not effectively regulated. Its careless use and disposal may put people and the environment at risk. In cell-based studies, TCS is harmful to many cells14. The precise role of TCS in selecting antibiotic resistance genes and multidrug resistance genes in the environment is unknown. It is also necessary to establish the TCS level required for tolerance choice in environmental communities. Future research should concentrate on finding signaling molecules controlled by TCS in different ways and determining their involvement in harmful or protective effects in various cell types14.
TCS has been shown to reduce the viability and growth of Michigan Cancer Foundation-7 (MCF-7) and SKBr-3 cells in culture at a range of 2.5-20 µg/mL. It also lessened the binding affinity of inhibitors in human and goose type-1 fatty acids as well as the enoyl-reductase level31, 32, 33. All present new reports indicate that widely used TCS cosmetic agents can trigger cancer, as shown in animal and human models21, 34, 35, 36 as well as in clinical studies. Therefore, government policies should reassess TCS usage in cosmetic products to prevent its harmful effects on human health. This manuscript's main aim is to highlight that TCS causes oxidative stress, an estrogenic, mutagenic, cancer-causing agent, and genotoxic agent present in cosmetic products. A further aim is to attenuate the TCS toxicity via natural products.
BIOSYNTHESIS OF TCS
TCS is a broad-spectrum antibiotic that inhibits bacterial fatty acid biosynthesis at the (Enoyl-ACP) reductase37. TCS is generally considered a broad biocide that targets bacterial membranes, and cellular resistance is rare if TCS does not have a distinct mode of action. TCS has been found to inhibit enoyl reductase FabI in various bacteria, including P. aeruginosa and S. aureus, after discovering that it blocks the enzyme in E. coli38. The enoyl-ACP reductase FabI family produces noncovalent, high-affinity ternary compounds with TCS and NAD(P)+ that effectively limit the enzyme's participation in biosynthesis39. TCS inhibits enoyl reductase by attaching to a location close to the nucleoside cofactor's nicotinamide ring. The TCS phenol ring interacts with the nicotinamide ring directly and enables substantial activities40.
More enoyl reductase genes have been identified after discovering TCS's inhibitory effect on enoyl reductase, including FabK in S. pneumoniae and P. aeruginosa, FabL in B. subtilis InhA in M. tuberculosis, and M. smegmatis 41. Both FabK and FabL are TCS resistant, whereas InhA is sensitive. Similar inhibition of TCS fatty acid biosynthesis was also observed in higher life forms, such as P. falciparum and T. gondii, which cause malaria42. Both of these species have a type II fatty acid synthase since they are apicomplexans. TCS was recently demonstrated to block a type I fatty acid synthase (a versatile human enzyme) in breast cancer cells, even though these enzymes are commonly thought to be antibiotic-resistant43.
DEGRADATION OF TCS
Antimicrobial compounds have shown a proclivity for bioaccumulation in underwater organisms, and they have been found to survive in aquatic environments for longer periods. The presence of TCS in the environment necessitates the monitoring of surface water. TCS was discovered in silt from Greifensee Lake in Switzerland deposited 30 years ago44. The survivability of TCS in sediment was confirmed in this investigation, and a breakdown of the TCS using various strategies. The TCS level in sediment has progressively enlarged since the early 1960s, when it was initially introduced, to the mid-1970s, indicating that its usage trends were becoming more widespread. This trend reversed from the mid-1970s to the early 1980s, with most wastewater treatment facilities introducing a different conventional treatment stage. TCS levels have risen since the early 1980s, owing to its rising popularity and use44.
Nonetheless, the comparatively large level of TCS found in a 30-year-old sedimentary layer from 1970 to 1971 indicated that TCS breakdown was highly sluggish in the sediment45. A parallel timeline pattern for TCS in estuary sediments in the United States was also documented. Antimicrobial chemicals can subdivide into sediments and withstand breakdown mechanisms under anaerobic circumstances, as evidenced by TCS's environmental survival in sediments. Furthermore, sediments are the last sink for the aqueous ecosystem. TCS persistence in this medium would be risky since bioturbation generated by animals or human excavation might push it back into the aqueous ecosystem46.
Despite its strong chemical volatility and resilience to both high and low pH, TCS has been discovered to be easily destroyed in the atmosphere due to photodegradation. Scientists found eight photochemical pathway subproducts in laboratory testing47. Researchers found TCS photoconversion to 2,8-DCDD with an output of up to 12% at pH > 8, using varied illumination intensities. Under the laboratory conditions (purified water), the production of 2,8-DCDD yield was compared to river water spiked with TCS. According to similar results across laboratory and real-world scenarios, TCS could transform into 2,8-DCDD in sunshine irradiated water sources48. TCS that persists in the secondary discharge after sediment processing may be chemically transformed after disposal. In the United States, sodium hypochlorite, a sterilizing oxidant and a producer of free chlorine is extensively used for various applications and may interact with TCS. In certain instances, the TCS phenol carbons may be chlorinated in either ortho- or para-positions, yielding three chlorinated TCS derivative (CTD) transitional compounds49. Direct photolysis is ineffective at degrading dioxin derivatives of TCS, so they are therefore not a public health problem6.
Similarly, chloramination of TCS results in CTDs comparable to those formed by the free method50. Chlorinated TCS derivatives like 4-Cl-TCS, 6-Cl-TCS, and 4,6-Cl-TCS have been found in sewage discharge51. CTDs have been discovered at the apex of marine biological chains and as biomethylated equivalents in fresh water, specimens taken downstream of a sewage discharge, and in carps that live in it due to the spread TCS-containing effluents in streams52. According to these findings, CTDs are either generated via TCS during water purification using free chlorine or are synthesized without passing through the conventional treatment processes. As a result, CTDs are regarded as a significant environmental concern since they have the potential to preserve or perhaps enhance the antibacterial and endocrine-disrupting properties of TCS. Furthermore, under spontaneous photochemical circumstances, CTDs such as 6-Cl-TCS, 4,6-Cl-TCS, and 4-Cl-TCS have been shown to release dioxins in water53.
Buth JM et al.,54 investigated the history of TCS dioxin photoproducts and their chlorinated counterparts in Mississippi River sedimentary basins. Sunlight irradiation of CTDs, which produces chlorinated dioxins, is another conceivable cause of TCS-derived pollutants. The photochemical breakdown of TCS occurs until by-products are subjected to ultraviolet rays after interaction with chlorinated water, 2,4-dichlorophenol (2,4-DCP), and 2,8-DCDD are formed. The chlorination of 2,4-DCP yields 2,4,6-trichlorophenol55. The transitional chlorophenols are then converted to chloroform and trihalomethanes56. The ways by which CTDs are converted to chlorophenols, chloroform, and trihalomethanes. TCS can be chlorinated by frequent exposure to chlorine at water treatment plants. A wastewater treatment plant discharges chlorinated TCS, which can be converted into more harmful dioxins by sunlight57. 2,4-DCP is a contaminant of concern according to the United States environmental protection agency and is hazardous to fish and other aquatic life58. 2,4-DCP is a chemical that is used to make insecticides, disinfectants, and antiseptics.
Furthermore, upon exposure to the sun's rays, the 2,4-DCP decomposes even more, perhaps resulting in more strongly chlorinated dioxins59. Due to low levels of ROS in natural rivers and the ineffectiveness of straight photolysis of TCS, research by Latch et al.,6 found that dioxin chemicals produced from TCS are not a public health risk. Microorganisms like Burkholderia, Pseudomonas, and Sphingomonas may degrade chlorine and CO2 under natural circumstances60.
According to Son et al. (2009)61, radicals that stimulate the destruction of transitional dioxins control TCS degradation by titanium dioxide photocatalysis. Furthermore, hydrogen peroxide enhances the oxidative process. TCS is stable at 50°C when it is kept separate from biotic contact and kept at a pH of 4–9. TCS degrades more rapidly in an aqueous medium at 25°C and pH 7, reaching 50 % in around 41 minutes. Within 4 hours after therapies, primarily 2,4-DCP is generated. TCS is easily degraded in aquatic environments by photolysis, with a half-life ranging from 1 hour in abiotic settings to roughly 10 days in freshwater sources62. Furthermore, depending on the reactivity of TCS with photochemically generated hydroxyl radicals, its aerial half-life has been predicted to be 8 hours63. Even while the current amounts of TCS and its by-products in the atmosphere are not dangerous, continued deposition of TCS into the atmosphere could approach a threshold value, affecting all categories of animals in the food chain64.
OXIDATIVE STRESS MECHANISM
If not adequately scavenged, these “two-edged sword” molecules, ROS, may disrupt the cellular redox equilibrium, resulting in oxidative harm to protein, lipids membranes, and nucleic acid65. When the dynamic balance in the synthesis and removal of ROS in typical circumstances is disturbed, "oxidative stress" is used66. The antioxidant defence mechanism, including CAT, SOD, GPx, GR, GSH, Glutathione disulphide (GSSG), and glutathione S-transferase (GST), works to counter the potentially harmful effects of ROS. As a result, ecologists may measure antioxidant levels to monitor the amount of oxidative damage induced in organisms treated with particular substances67.
Catalase (CAT)
Adult zebrafish livers treated to different dosages of TCS, SOD, CAT, and GPx enzyme activity were assessed. When the antioxidant defence fails to resist this ROS, the overwhelming generation of ROS is a potential source of enzyme inactivation. ROS elimination is caused by the change of radicals without oxygen into hydrogen peroxide molecules by SOD, further reduced into H2O by CAT and GPx68. As a result, if these critical first-line defences become less active, H2O2 and its degradable compounds may accumulate69. SOD activities were inhibited in all treatment groups relative to the control group, according to the research. At the lowest dose of 50 g/L, though, the caused suppression of SOD was significant (p < 0.05) when compared to the control70. The lower concentrations of SOD activity in the treatment groups' liver tissue may be related to the ROS generated by TCS treatment. The CAT and the GPx enzymes must maintain intracellular redox equilibrium and H2O2 degradation68.
The activity of CAT followed a parallel path to that of SOD in the liver. There was a substantial decrease in CAT activity between the treatment and control groups (p < 0.05). The reduction of CAT activity in the liver varies considerably between treatment groups at p < 0.05, with the effect of suppression being most noticeable at the lowest dose (50 g/L)71. The apparent decrease in CAT behaviour implies that the produced H2O2 may not be quickly destroyed by CAT, indicating a redox imbalance in the cell. The pattern toward reduced CAT activity was lower in the liver72. Poor regulation of antioxidant enzyme activity may result in an increased amount of ROS, thus reducing the antioxidant system's efficiency. This theory is supported by the idea that increasing ROS produced the reduction in SOD, resulting in a loss in enzyme efficiency and function73.
CAT plays a vital role in the progression of ROS due to TCS toxicity (Figure 1). After subchronic treatment with TCS, the enzyme activity of SOD and CAT was assessed in the brain of adult zebrafish74. CAT activity was reduced to control across treatment groups. There was a statistically significant difference in CAT activity between the control and increased TCS exposure (100 g/L and 150 g/L). TCS may alter the zebrafish brain's antioxidant system due to the reduction of CAT and SOD activities. Despite this, mean SOD activity in the brain was significantly greater than in the liver for both TCS treatments, whereas mean CAT function in the brain was significantly lower. The various physiological roles of the organs may describe the discrepancy in numerical ranges of antioxidant enzymatic activity74.
Reduced glutathione (GSH)
GSH and GSSG (the glutathione system) are non-enzymatical antioxidants, and also GSH enzymes are regarded as the second-line defensive mechanism for oxidative damage69. The action of GR is critical for GSH regeneration as a defence mechanism against oxidative stress. Because GSH is converted to an oxidized form, GSSG, during metabolic activity, GR recycling of GSH from GSSG is critical for sustaining the cellular antioxidant protective mechanism75. Additionally, it’s important to note that GPx is involved in detoxifying ROS and H2O2 through the oxidation of GSH to GSSG, implying that suppression of GPx activity may impact glutathione and its conjugate levels9.
Scientists found a significant decrease in GSH concentration in adult zebrafish livers exposed to TCS compared to a control group (p0.05). GSSH concentrations were also considerably less in TCS-exposed groups than in controls (p < 0.05). Furthermore, except for the 100 g/L TCS exposure group, the treatment groups did not consider the decrease in GSSG concentrations76. Following TCS exposure, the GSH/GSSG concentrations were decreased, indicating GSH levels (Figure 1). GR activity was observed to be less than control in treatment groups. Besides the 50 g/L and 100 g/L groups compared to the control group, the reduced GR activity was not substantial within groups (p < 0.05)77. The decreased GSH/GSSG found in the current research may explain that GSH could not be revived to regain its normal concentration in the liver after treatment to TCS concentrations due to lower GPx and GR activities. The results demonstrated that adult zebrafish livers lost antioxidant mechanisms following subchronic exposure to TCS.
In contrast to the roles of GPx and GR, the study found that treatment groups had higher GST activity in their livers than the control group. GST is a biotransformation enzyme that plays an important role in coupling glutathione with various contaminants68. GST protects cells from oxidative damage in phase II detoxification by catalysing the tripeptide GSH with electrophilic substrates. Except for the difference between the 150 g/L groups and the control, which was notable at p < 0.05, there were no substantial variations in increased GST activity among groups78.
The research results are under the enhanced GST activity reported in zebrafish larvae challenged following TCS exposure (250 – 350 μg/L)79. De novo synthesis may account for the decrease in rGSH content in the cells, explaining its stable level following TCS treatment. The homocysteine molecule is linked to the production of rGSH, and the cysteine amino acid in GSH is made from the same pool of homocysteine utilized to make S-adenosylmethionine (SAM). For methyltransferase enzymes, SAM serves as a methyl donor. As a result, the GSH system is intimately linked to DNA methylation, critical throughout embryonic development74.
Malondialdehyde ( MDA )
The peroxidation of cell membrane lipids is a potential result of organisms being exposed to ROS-generating pollutants. MDA is a result of membrane LPO and is often employed as a biomarker to indicate the degree of cell membrane damage80. The inability of the antioxidant system of the liver to remove ROS caused by TCS after subchronic exposure might explain the enhanced MDA concentrations in the liver (Figure 1). A recent study on Daphnia magna (D. magna) showed that MDA levels were significantly greater than controls after 6 hours of TCS exposure. Still, they were significantly lower after 24 and 48 hours, indicating lower MDA levels. The increase in MDA content in Eisenia fetida, according to Lin D et al.,81, showed oxidative stress caused by TCS. The current research found an opposite connection between MDA concentration and SOD action, rising first and then decreasing. Consequently, the decrease in MDA was likely due to the defensive activity of SOD in TCS-exposed D. magna against oxidative damage82.
In D. magna, TCS is responsible for the production of MDA, aminopyrine N-demethylase (APND), ethoxyresorufin-O-deethylase (EROD), and erythromycin N-demethylase (ERND)83. Additionally, increased amino acid levels in daphnids, like glutamate, proline, and glutamine, have been related to an overall state of oxidative stress84. TCS has been found to change the expression of stress-related proteins, including heat shock protein 70 (hsp-70) and glyceraldehyde 3-phosphate dehydrogenase (GAPDH) in D. polymorpha, in addition to LPO85. TCS exposure produced CAT, EROD, ERND, and APND in yellow catfish Pelteobagrus fulvidraco86. Up- and downregulation of Cyp1a, Cyp3a, and Gst expression was seen in response to TCS level and duration of exposure, a trend similar to MDA production87. In goldfish Carassius auratus, TCS-induced oxidative damage was also found to have elevated MDA. After TCS exposure, the goldfish's liver showed various antioxidant enzyme responses and changes in MDA levels over a pH value ranging from 6 to 99, 82.
Total Antioxidant Capacity (TAC)
Antioxidant molecules, particularly those derived from plants, have grabbed scientists' attention in recent years. On the one hand, there is expanding proof of the preventive effect of vegetables and plant foods on cancer and other neurological disorders. On the other hand, there is emerging fear about the health consequences of synthetic antioxidants currently practiced as food additives88. Antioxidant substances are free radical scavengers since they limit or postpone substrate oxidation by free radicals, leading to substantial protection of LPO in biological systems. The primary natural antioxidants found in plants, foods, and drinks are phenolic and polyphenolic compounds89. These compounds, which involve flavonols, quercetin, catechins, and anthocyanins, have common structural composition. They optimize the oxidative stability of foods and human systems through their redox characteristics which can help neutralize free radicals, quench singlet oxygen, and decompose hydroperoxides, among other things90.
The TAC was determined using the extract's reduction of Mo (VI) to Mo (V) and the synthesis of a green phosphate/Mo(V) complex at an acid pH91. It assesses TAC that is both water-soluble and fat-soluble. The findings show that the methanol and acetone extracts have greater TAC (ascorbic acid equivalent) at low quantities. However, the variations are not statistically significant (p = 0.05) compared to the n-butanol extract. Furthermore, the n-butanol extract was shown to have substantial overall antioxidant activity at greater concentrations, equal to 90 mg/g ascorbic acid11. As the antioxidant capacity of ascorbic acid has been utilized as a benchmark against which plant extracts with potential antioxidants have been tested, this indicates that the n-butanol extract may have similar antioxidant components92.
It has been proposed that the donation of electrons is linked with antioxidant activity, which reflects the reduction in potency of bioactive substances. Antioxidants may function as reductants, and the deactivation of oxidants by reductants can be regarded as redox processes in which one of the reaction species is reduced at the cost of the other being oxidized90. The Fe3+/ferricyanide complex is reduced to the ferrous form when reductants, such as antioxidant compounds, are present in the samples. The extracts' reducing power increased as concentration increased, indicating that the extracts' capacity to donate electrons is sensitive at lesser concentrations. The considerably higher absorbance values of n-butanol extract than gallic acid indicates that the n-butanol extract has strong redox capability and may function as a reducing agent, hydrogen donor, and singlet oxygen quencher93. However, the extracts included the identical classes of phytochemicals, therefore the quantitative variation in antioxidant activity may be due to differences in phytochemical concentrations94. The findings indicate that the n-butanol extract has a higher concentration of antioxidants than the methanol and acetone extract95. It was discovered that the root of A. difformis has antioxidants that may be important in the therapeutic activity of this plant portion. These results call for more research in isolating and characterizing the bioactive molecules accountable for the antioxidant action12.
Cell membrane Damage
Vischer and Regös may have been the first to describe TCS' antibacterial activity, which they demonstrated via topical treatment96. TCS's various action modes and cellular targets have been the subject of further research, which continues today. TCS was originally believed to react with the prokaryotic cell membrane in a nonspecific manner97. The TCS resistance of gram-negative bacteria has supported this hypothesis due to the membrane of their cells. The genetic response to TCS for M. tuberculosis has been investigated by Betts et al. (2003)98. Changes have been found in various cell wall genes transportation, detoxification, and other functions such as DNA replication and transcription. In E. coli and Rhodospirillum rubrum (R. rubrum) S1H, many genes implicated in the membrane tension reaction pathway were investigated99. Substantial variations in phenotypes of genetic code linked to the cell wall, flagella, cell envelope, multidrug efflux, and membrane structure were discovered during the electro-Fenton conversion of TCS. These results add to a previous study describing increased TCS resistance from an overexpressed acrAB multidrug efflux pump99. TCS is thought to interact with the Agrobacterium tumefaciens transcriptional repressor AcrR, causing structural changes and blocking it from adhering to the promoter of the efflux pump AcrA100.
In human erythrocytes, the association of TCS with the cellular membrane was also investigated. TCS caused K+ outflow and visible haemolysis, implying membrane destruction while counteracting hypotonic breakdown caused by membrane enlargement101. TCS also decreased the activity of Na+, Mg2+-ATPase, and K+, which is membrane-bound. According to these findings, TCS induces membrane instability, disrupts monovalent ion movement, and alters the overall osmotic balance of red blood cells102. Several investigations have shown proof of membrane disruption in the form of decreased integrity and permeability. Guillen J et al.,103 used nuclear magnetic resonance (NMR) spectroscopic to establish how TCS interfaces with the plasma membrane. They discovered that TCS introduction into aquaphobic regions within the lipid membrane, perpendicular to phospholipid molecules.
Cellular Longevity
TCS and final cell destiny drew attention because of its application in oral hygiene items, as shown by two pivotal research on human gingival cells85. TCS leads to having a negative impact on cellular longevity (Figure 1). A new apoptotic trigger in epithelial cells may be TCS, cytotoxic to gingival epithelial cells and gingival fibroblasts. Till now, research in both human and animal model systems has taken a more concise approach to link TCS-induced cell death to other cellular rivals. Several dosages and scheduled responses were seen if TCS was applied to placental human choriocarcinoma cells104. Elevated TCS levels have suppressed the release of β-human chorionic gonadotropin (β-hCG), despite increased oestradiol and progesterone production (104). By stimulating caspase-3 and fragmenting Hoechst 3342 labelled DNA, considerable cell mortality was detected as apoptotic in furthermore to decreased growth104. Likewise, Winitthana et al. (2014)105 found that 24-hour exposure to 10 M TCS caused cell mortality and suicide in human lung cancer anoikis-resistant H460 cells. Nevertheless, safe levels (to 7.5 μM) improved cell development without modifying the proliferation (elevated colony counts and decreased size). TCS also encouraged cell migration and invasion, as well as the epithelial-to-mesenchymal transition (EMT).
A research team used BG-1 ovarian cancer cells in various animals and laboratory experiments to determine how TCS influenced the development and proliferation of such cells. TCS stimulates cell proliferation and cyclin D1 gene expression and protein levels while lowering p21 and Bax genetic code appearance and protein level106. The ER antagonist ICI 182,780 greatly inhibited these impacts, implying that ER is involved in TCS-induced cell cycle development and its antiapoptotic function107. MCF-7 bosom malignancy cells and LNCaP prostate cancer cells both reacted to TCS in the same way, according to other researchers in the same group. 1 M of TCS raised development and multiplication in MCF-7 cells over six days, with elevated cyclin D1 and lower p21 expression108. Research on the effect of TCS on cell destiny has shown that it has estrogenic, proliferative, and apoptotic properties109. TCS regulation is especially susceptible to genes and proteins that control the cell cycle and apoptosis. In addition to other research data such as cell type and exposed length, the variation in ultimately cell destiny appears to imply interracial difference and dosage reaction. Extending our understanding of the presence and identifying a precise molecular "switch" that can shift the balance in support of apoptosis or persistence could be a crucial topic of upcoming research14.
Inflammation
TCS has long been known as a successful treatment for infectious dermatitis, with the compound's therapeutic ability ascribed entirely to its antimicrobial action110. Researchers didn't establish a relationship between TCS exposure and non-infectious inflammation remission until the last two decades of the previous century. The usage of antibacterial agents as anti-inflammatory therapeutic has gained much attention over the past two decades111. Anti-inflammatory activity has been demonstrated in many antibiotics, including quinolones and macrolides112.
According to Gaffar A et al.,113 TCS reduced LPO synthesis, 15-LPO, 5-lipoxygenase, IL-1-induced prostaglandin E2 (PGE2), and cyclooxygenase-1 (COX-1), COX-2 in gingival cells. Additionally, TCS has been found to inhibit a broader variety of inflammatory agents, such as arachidonic acid and prostaglandin I2 (PGI2) produced by TNF-induced PGE2, IL-1β, phospholipase A2 (PLA2), COX, and tumour necrosis factor114. Furthermore, individuals who received a mouth rinse containing 0.15 percent TCS had considerably fewer oral erythematous lesions than those who received a TCS-free mouth rinse in a crossover trial. TCS' anti-inflammatory effects had been proven and were generally acknowledged throughout the scientific and medical sectors by that time115.
To date, further research has emphasized the anti-inflammatory properties of TCS. In human gingival fibroblasts, Mustafa M et al.,15 found IL-1β, IFNγ, MHC class II, and PGE synthase-1 as TCS targets. Notably, investigations determining the subcellular location of TCS indicate priority for nucleus accumulation over cytosolic accumulation. Since the cytoplasmic TCS absorption was greater at first, following successive washing, a large percentage of cytosolic TCS was removed, whereas nuclear TCS was retained116. This may account for the altered inflammatory signaling seen in TCS. MicroRNA (miRNA) regulation of the TLR pathway was responsible for inhibiting LPS-induced cytokine production and antimicrobial activity in primary human oral epithelial cells117. The results were similar in cells obtained from diabetes individuals, where the TLR response was amplified118.
On the other hand, TCS has been shown to inhibit the TLR response induced by LPS via altering miRNAs (lowering miR155s but promoting miR146a). TLR4 induced changes in inflammatory responses in mice's skin and leukocytes when they were topically challenged with TCS119. Similarly, TCS suppressed PGE2-stimulated matrix metalloproteinase-13 (MMP-13) or parathyroid hormone (PTH) production in osteoblastic rat osteosarcoma cells120. Since hyperactive MMP-13 has been related to periodontal disease, it's been proposed that TCS may protect against the inflammatory condition of the mouth by acting on that same enzyme, besides others121.
Notably, TCS has proven beneficial in treating various inflammatory states, such as hidradenitis suppurativa (HS) and cardiovascular disease. Furthermore, the application of TCS-drenched ureteral stents appears to become a potential strategy for treating urinary tract infection (UTI) and inflammation. In pregnant women, an enhanced urinary TCS was linked to an elevation in serum IL-6, suggesting a potential pro- or anti-inflammatory function122. TCS is a mediator of immunological and inflammatory responses, as shown by the abundance of data available. Nonetheless, mounting evidence suggests that TCS significantly amplifies and worsens the ultimate result when a pre-existing unfavourable state, like inflammation or tumour, is present14.
Cellular Signalling
The ability to adapt to the continuously shifting intracellular and external environments is greatly facilitated by communicating efficiently. The transmission of data containing particular commands is carried out by carriers that work sequentially along a specified way. Moreover, tasks are often performed by sequentially transducing numerous signals via a complicated, intertwining network, including a diverse array of mediators123. As a result, the relevance of cell signaling cascades in response to xenobiotics must not be overstated.
Cell lines of humans have given a lot of knowledge, especially in researching stressors and xenobiotic-sensitive signalling molecules like TCS (Figure 1). The conventional MAPK has also been implicated as TCS targets124. The TCS-induced proliferation of JB6 Cl 41-5a cells was aided by the activation of c-Jun N-terminal kinases (JNK), p38 MAPKs, and ERK1/2, in addition to Akt125. Proliferation induced by TCS was significantly reduced when phosphoinositide 3-kinase (PI3K) or MEK1/2 were inhibited. According to the results of another study on rat neural stem cells, cytotoxicity and apoptosis induced by TCS were linked to stimulation of the JNK and p38 pathways, as well as blocking of the ERK, Akt, and PI3K pathways126. This indicates that these proteins are involved in both cellular stability and mortality due to TCS's action. Studies used the hypothalamus from Sprague-Dawley rats and Human Nthy-ori 3-1 thyroid follicular cells to demonstrate that TCS stimulates p38 and JNK127. In that research, TCS affected the amount of thyroid peroxidase (TPO) via stimulating the thyrotropin-releasing hormone receptor via p38 MAPK128. TCS reduced AP-1 sequence and Fos/Jun interaction within the C-fos promoters and Mmp-13, which reduced Mmp-13 synthesis in mouse osteoblastic osteosarcoma cells120.
The researchers were particularly interested in TCS's endocrine-disrupting properties, particularly its estrogenicity. Kim YS et al.,106 demonstrated that TCS induced BG-1 ovarian cancer cells proliferation via the ERα. Proving the function of ER, the application of ICI 182,780 restored TCS proliferation characteristics along with related modifications at the levels of cyclin D1, p21, Bax, and protein. Similarly, following TCS treatment, the ER was involved in MCF-7 cell proliferation and an increase in the size of breast tumours in mice109. The suppression of TCS by ICI 182,780 or kaempferol, as well as the activation of insulin-like growth factor (IGF), particularly pIRS-1, PKB, MAPK, and pERK1/2, supported this theory30. In addition, kaempferol reduced the development of VM7Luc4E2 cells stimulated by TCS29. These findings are consistent with those of Huang et al.,129, who previously described the estrogenic activity of nanomolar doses of TCS in identical cells.
The TCS has a dual impact on ER signaling, according to new research. For instance, Henry and Fair33 showed that TCS at concentrations ranging from 7nM to 700M shows estrogenic action when given solely to MCF7 cells but becomes antiestrogenic when combined with E2. According to research, TCS has little effect on rat uterine development, but it may help ethinylestradiol (EE) perform better130. TCS enhances EE-induced suppression of ER and ER expression in independent research but does not stimulate ER when given alone at dosages ranging from 30nM to 100M. According to the study, TCS also reduced E2 and oestrogen sulfotransferase efficiency in the sheep placenta131. In contrast, a TCS-derivative combination produced enhanced ERβ activity but not ERα, resulting in neurological and psychological problems in zebrafish132. ICI 182,780 and RU 486 restored both abnormalities, suggesting that the antimicrobials may have an estrogenic effect.
In terms of TCS's androgenic characteristics, it was discovered that TCS inhibits-TSN-associated transcription while promoting androgen-dependent transcription133. According to Riad MA et al.,134, TCS therapy alone or in combination with butylparaben reduced TSN, follicle-stimulating hormone (FSH), and luteinizing hormone (LH) levels in weanling male rats. At the same time, it increased E2 after a single TCS treatment. Bicalutamide, an androgen receptor (AR) antagonist, significantly inhibited TCS-induced proliferation and translocation of LNCaP cells.
Protein structure and dynamics are affected by calcium levels inside cells. Conversely, Ca2+ binding to proteins help maintain the ion's concentration in a physiological limit while simultaneously activating various cellular functions such as gene expression, movement, secretion, and longevity135. Apart from proteins, several factors, like xenobiotic exposure, affect intracellular Ca2+ concentration. TCS increased cytosolic Ca2+ in primary skeletal myotubes in a dose-dependent manner, regardless of exogenous Ca2+, via the Ca2+ channel ryanodine (Ry) receptor type 1 (RyR1)26. Accordingly, in vitro and in vivo exposure to TCS was impaired by muscle contractility136. TCS also disrupted the two-way communication in RyR1 channels and Ca2+ ions by inhibiting Ca2+ entry mediated by excitation137.
The data on the xenobiotic reaction to TCS has shown that TCS activates or suppresses many signaling pathways. Depending on the experimental circumstances and model under study, diverse results occur across species and even within the same species138. Although significant advancements in TCS signaling have been accomplished yet, there is still much to learn about TCS's modulatory impacts on cellular physiology, particularly in human-based systems. TCS therapy has an unknown impact on many human cell types and tissues, so it's critical to determine which signaling pathways are involved in cellular development, metabolism, and general activity14.
ESTROGENICITY
TCS, an antibacterial molecule, has a negative impact on human genotoxicity, and its antiandrogenic capability damages DNA. TCS has induced IP at a dosage of 15 mg/kg for two days in a row. TCS therapy results in a substantial reduction in testicular hormones (testosterone FSH and LH)139. TCS's impact on the environment and human health. TCS is prooxidant and cytotoxic in a variety of ways, according to cell research. TCS is involved in both estrogenicity and anti-estrogenicity in cancer development140. TCS research on surface water and wild fish. TCS and a binary combination with BE2 had a negative impact on testicular growth and reproduction in fish at a dosage level of TCS 117.9 mg/L141.
MUTAGENICITY
The mutagenic capability of TCS has been studied in several ways, including in vitro and in vivo tests that look for point mutations, recombination events, and frame shift mutations in prokaryotic and eukaryotic systems. On TCS, in vitro microbial reverse mutation tests (Ames Assays) were performed14. Various Salmonella typhimurium (S. typhimurium) strains, with or without S9 metabolic stimulation, were employed in vitro tests using TCS. Muller D et al.,142 also investigated the mutagenicity of TCS in a bacterial reverse mutation test using intrasanguineous hosts. All findings from these reverse mutation tests in vitro and host were negative. It showed that TCS did not induce gene mutations143.
Gene mutation investigations in mammalian cells via in vitro model suggested that metabolic stimulation of mouse lymphoma L5178Y cells with and without TCS has shown the ability to produce mutations in the TK region of a gene144. In host-mediated research in mice, Müller D et al.,145 investigated the mutagenicity of TCS at the TK gene in mouse lymphoma L5178Y cells, finding no treatment-related changes in malformation rate. There was no rise in the mutant rate at doses that did not cause cell death (up to 20 g/ml without S9 and up to 15 g/ml with S9).
However, De Salva et al.,146 and Bhargava et al.,147, continue to believe that TCS is not a mutagen, and antimicrobial individual care items are not harmful to one's health. Despite the positive results of the mammalian spot test, most of these tests revealed that TCS had no mutagenic ability. Fahrig R et al.,148 was the first to conduct the mammalian spot test and yielded a positive result, but was later replicated by Russell et al. (1980)149 yielded negative results. Fahrig R et al.,148 have been challenged, claiming that the optimal TCS dosage could produce maternal toxicity, preventing offspring evaluation. It's unclear why these two types of research, which used the identical methodology, produced such disparate findings. Fahrig R et al.,148 employed a larger dosage of TCS soluble in hank's balanced salt solution (HBSS). In contrast, Russell LB, and Montgomery C. et al.,149 found TCS insoluble in HBSS and consequently applied methanol to dissolve the antibiotic. Russell LB and Montgomery C.149 assumed that the Fahrig R et al.,148 investigation was unsuccessful in inserting one of the TCS dams because the absorption of the TCS in HBSS was restricted, explaining the toxicity at 50 mg kg−1. It was extremely hazardous to embryos149. Many scientists appear to endorse this study observations149 as TCS safety reviews.
The researchers have carried out three-wide genomic DNA (0.2T-AMX, 0.2T-CHL, and 0.2T-TET) sequencing for reproduced mutants induced by TCS (n = 6) and for wild strain E. coli (n = 2) to find important genetic modifications of antibiotic resistance caused by TCS. When compared to non-treated E. coli, sequencing of 6 resistant mutants produced following treatment of TCS at 0.2 mg/L showed 14 genetic modifications in 11 genes and 9 alterations in intergenic regions. The +A insertion in the insB-1 gene was seen in all sequenced mutants, along with substitution alterations in the fabI genetic code150. Because TCS interacts with the enoyl reductase FabI, which is expressed by the fabI chromosome, genetic variations in the fabI chromosome may impair TCS effectiveness by altering the shape of the targeted fabI protein151. Apart from the typical mutations, several strain-specific mutations have been discovered. The citC (A346T), acrR (L65R), and soxR (R20S) substitute alterations, for example, were detected solely in the 0.2T CHL strains. In comparison to other strains, the 0.2 T-TET strain contains a 1 bp frameshift in the insl-1 genetic coding and a replacement variation in the marR chromosome (T72P)152. At the same time, transcriptional analysis was used to identify the molecular processes behind TCS-induced antibiotic resistance. Complete genome Illumina RNA sequencing was used to see how three mutant kinds (n = 9) and wild-variant E. coli (n = 3) responded to the 8-hour 0.2 mg/L TCS treatment. Acute TCS treatment leads to typical transcript alterations between mutants and wild-kind E. coli compared with untreated wild-kind E. coli (n = 3)151. In contrast, the cellular antioxidant genes soxS, yhcN, and YgiW and the membrane encoding porin gene ompX have decreased153. TCS induces oxidative stress in E. coli at a concentration of 0.2 mg/L, while simultaneously reducing the regulation of genes that encode antioxidants, activating the SIM response to DNA deterioration152.
TCS-induced genetic changes may have enhanced antibiotic tolerance by modulating the genetic code's appearance concerning antibiotic resistance. Since the promoter of the gene ampC encoding beta-lactamase overlaps the frdD gene space in E. coli K-12154. The frdD alteration observed in 0.2T-AMX mutants could have impacted the ampC supporter endurance155, resulting in greater ampC expression (log2 fold change (LFC) = 5.4) and elevated beta-lactam antibiotic tolerance156. Furthermore, soxR gene alteration may cause a spike in soxS expression157, leading to a rise in efflux via boosting acrAB regulation158. As a result of the overexpression of the AcrAB many drugs exporter channel, multiple antibiotic resistance is likely to occur159. Mutations in the marR gene may have reduced adhesion capacity in 0.2T-TET mutants, resulting in upregulation of marAB genes that control overall multidrug resistance160. As a result, MarAB may have sparked the development of an antibiotic resistance genes cascade, including GadAB-YadGH and AcrAB-TolC161.
CARCINOGENICITY
TCS is a diverse antibiotic negotiator often used in cosmetics, toothpaste, and other consumer goods. Queries have been expressed about the blend's wide-ranging use in customer goods and its recognition in bosom milk, pee, and sera. It’s possible linked to a variety of human health effects16. The compound's extensive use in consumer goods, as well as its presence in bosom milk, pee, and sera, has sparked worries about its possible link to a variety of human health effects. Recent data put forward that TCS can serve a part in cancer growth, possibly due to its estrogenic properties or propensity to suppress fatty acid production16.
The most common mechanism of endocrine disturbance by exogenous substances is the suppression of the internal secretion (hormones) from attaching its receptor sites by trying to compete for sense-organ linkage locations with the competitor140. This is one of the mechanisms via which TCS causes endocrine dysfunction26. It is well established that when a ligand binds to a receptor site, it induces conformational changes in the sense-organ, resulting in the synthesis of transcription factors essential for the representation of internal secretion sensitive genes162. Hypospadias, cryptorchidism, and cancer are uncontrollable physiological outcomes of the antagonist's representation of oestrogen-sensitive genes140.
Carcinogenicity and precocious puberty might be interpreted as a result of receptor overstimulation, presumably caused by the high TCS level, or as a result of TCS occupying the receptor's ligand engaging domain33. More research is needed to link TCS concentrations in the environment with observed physiological consequences in animals, such as unfavourable fertility impacts. However, the in vivo toxicity of TCS has not been established accurately, noticeable concentrations of the substance in exposed humans' body fluids. TCS bio-accumulates and is widely dispersed in human tissues, as evidenced by the increased TCS levels in tissues compared to ambient levels26.
There have been reports of human beings developing allergic responses to TCS. Extensive usage of TCS-containing hand detergents has been linked to dermatitis or subsequent exposure to sunshine163. Similarly, after extended usage of toothpaste containing TCS, lesions have been reported to occur in the oral cavity and on the lips of human patients164. Elevated TCS concentrations in urine have been linked to immunological malfunction, allergic responses, and the development of asthma in children165. TCS has been shown to modify the structure of human serum albumin166. Endogenous molecules are obstructed by toxic substances attached to serum albumin and alter the shape of the protein molecule, thereby impairing its function or even altering its physiological activity. Researchers found that greater urinary concentrations of TCS were associated with lower fecundity in women88.
Xenoestrogens are oestrogen-like chemicals widely present in cosmetics, insecticides, and plastic bottles. Xenoestrogens interact with oestrogen attachment to oestrogen receptors in the human body, resulting in oestrogen-dependent health consequences such as maturation, reproductive health, and fertility167. TCS is a less well-known xenoestrogen with antimicrobial properties often found in cosmetic products, toothpaste, detergent, and other consumer goods. The ubiquitous usage of TCS, along with its presence in pee, sera, and in women's bosom milk, has prompted concerns about its link to various health consequences, including cancer168. According to an existing study, TCS can be estrogenic and anti-estrogenic33. Recent evaluations imply that TCS is estrogenic at lower concentrations because it promotes female sex hormone-sensitive bosom cancer cells. TCS inhibits the development of these cells at increasing concentrations, implying that higher doses may have an anti-estrogenic impact169.
The impacts of TCS on bosom cancer cells in vitro may be affected by concentration and other variables like oestradiol (natural oestrogen)170. Untimely bosom development, antimicrobial opposition, and hypersensitivity are possible health consequences171. However, the existence of TCS in milk indicates that it has passed through the human bosom, raising worries about its potential role in developing bosom cancer170. Plasma and sewage from people indicate the systemic transfer of TCS to humans, although local absorption from cosmetic goods applied to the bosom region is another exposure mechanism. TCS is a type 1 FAS enoyl-reductase blocker that is impactful against the bosom cancer cell lines MCF-7 and SK Br-3 in cultured cells172. The disruption of human FAS through a different method and at various active sites reinforces the idea that type 1 FAS could be a chemotherapeutic goal. It also implies that inhibiting any of this multipurpose enzyme's activity could be useful31. When a substance is so widely diffused in the aquatic environment, it can induce an endocrine disruption in aquatic animals, which is a reason for concern. The early investigations in medaka fry (Oryziaslatipes) indicated that TCS could be slightly androgenic based on alterations in fin size and sex ratio fluctuations173. TCS has been demonstrated to bind to proteins in other investigations. In North American bullfrogs, it binds to thyroid hormone receptors and disrupts their endocrine system174. TCS can potentially cause endocrine disruption by raising thyroid hormone levels. Thyroid hormone levels above a certain threshold may indicate a greater chance of getting bosom cancer175. Thyroid dysfunction is more common in bosom cancer patients than in healthy people, although no clear link has been discovered176.
Environmental chemicals may harm human health and induce carcinogenesis, according to accumulating data. TCS enhances the release of the VEGF, a substance that promotes tumour development177. This process involves the direct stimulation of a membrane ion channel which causes an elevation in intracellular calcium levels. In primary cultivated human prostate cancer, stromal cells show that ecologically significant levels of TCS activate a TRP family, TRPA1 (Transient Receptor Potential Ankyrin 1), using calcium imaging and electrophysiological approaches. TRPA1 activation of TCS raised baseline calcium in stromal cells, boosted VEGF production, and enhanced epithelial cell proliferation178. Immunofluorescence labelling of prostate tissue in formalin fixation and paraffin embedding revealed that the TRPA1 channel was expressed only in prostatic adenocarcinoma stromal cells179. Although the tumour’s androgen reliance has long been known, epidemiological studies imply that elements from a Western lifestyle can also take part in its growth180. Prostate cancer growth and development are thought to be influenced by epithelial-stromal interconnections181. Carcinomas are two interconnected parts: neoplasia epithelia cells and the supportive tumour stroma, which secretes cytokines and growth factors to control key procedures such as tumour propagation, vasculature, and penetration182.
GENOTOXICITY
TCS and triclocarban effect on the Tetrahymena thermophila (T. thermopohila) inhibit T. thermpohila with 24 h EC50 values of 1063 and 295 μg/L-1. Respectively both TCS and TCC significantly damage DNA183. The study of TCS on goldfish for 28 days results in TCS damage the erythrocyte tail DNA184. The dose of 0.125 mg/L-1 did not affect the size and shape of the cell. But the dose of 0.5 mg L-1 affects sexual reproduction and damages dependent DNA standards184. The broad spectrum TCS study on the zebrafish had a ratio of 0, 17, 34, 68 μg/L TCS for 42 days. Antioxidant-related gene at 34 the gills were significantly down-regulated as compared to 68 μg/L. In the 34 and 68 μg/L TSC groups, the Bax gene was substantially up-regulated in the ovary. In zebrafish, a greater dosage of TSC may induce oxidative damage in the gills and ovaries, as well as a faster ROS-dependent ovary opposite185.
The pharmaceutical, personal care products found in the aquatic ecosystem for a decade have a potent biological effect in the non-target organism. TCS genotoxicity increased dose-dependent at different dose levels TCS 0.1, 0.15, 0.2, 0.3 M used to prevent the effect of antibacterial TCS and antibiotic trimethoprim (TMP). Significant DNA damage at extremely low levels affects haemocyte functioning186. The trail TCS was conducted on goldfish (Carassius qurtus) the dose level (control, DMSO control, and ½, ¼ and 1/8 LC50) effect of genotoxicity and micronucleus (MN) and nuclear abnormalities (NA) frequencies in peripheral blood. TCS 96 h median lethal concentration was 1111.9 μg/L significantly increase MN and NA frequencies TCS cause oxidative stress and a genotoxicity response in goldfish9.
Different doses of TCS response increased in the proliferation of MCF7 DOS cells elicited by E1. The TCS is at 76 – 87 % and 68 — 95% at the maximum level. MCF7 Bos cell significantly increased by PFOS and 0.01 and 30 kg.ml-1 proliferative response of 116% of the maximum E233. According to research, TCS at 0.5 mg/L reduced the development of the unicellular alga Closterium ehrenbergii and caused DNA damage187. The MN test TCS caused substantial DNA genetic damage in single-cell gel electrophoresis at all doses (1, 2, 3 M)187. TCS increases hepatocyte proliferation-induced fibrogenesis, produces oxidative stress, and boosts inflammatory response, according to in vivo and in vitro studies using different biomarkers188.
Micronucleus (MN)
The MN and NA are the most significant cytogenetic damage assays for genotoxicity189. A most sensitive method for DNA strand breakdown individual cell genotoxicity test in fish. MN frequencies in zebrafish subjected to the maximal TCS dosage.
Nuclear Abnormalities (NA )
The smear was fixed in absolute methanol for 10 minutes, air-dried, and stained with 10% Giemsa stain for 8 minutes in a study of MN and NA. MN and NA rates were significantly different in the TSC-treated group190. The haemocytes' genetic damage was substantial at all three TCS doses, and it followed a concentration- and time-dependent pattern. The comet test in Artemia salina was also used to assess TCS's genotoxicity191.
Concentration of TCS | Effects | References |
---|---|---|
0.0028 – 28.9 µg/ml | Estradiol antagonism | 26 |
0.00002 – 28.9 μg/mL | Cell proliferation, estradiol antagonism | 170 |
0.002 – 200 μg/mL | Cell proliferation, estradiol antagonism, cytotoxicity | 33 |
0 – 20 μg/mL | FAS inhibition, reduced cell viability | 35 66 |
0 – 100 μg/mL | FAS inhibition reduced cell viability non-toxic to normal cells | 36 , 32 |
1 µM | Activate transient receptor potential Ankirin-1 (TRPA-1) in human prostate cancer stromal cells | 178 |
0.1 – 10 µM | Proliferation and anti-apoptosis inhibit ROS production | 109 |
10 – 6 M | Up regulate pIRS-1, PKB, and MAPK in breast tumor growth | 192 |
10, 100 and 200 mg/kg | Induce mouse liver tumor via CAR and PPARα activation | 147 |
10 - 5 – 10 - 7 M | Induce proliferation of breast cancer cells through ER pathway and activated CXCR4 receptor involved in metastatic behavior | 29 |
NOVEL TREATMENT STRATEGIES
Normal cells can convert into cancer cells due to a variety of genetic and environmental factors. It causes them to develop abnormally and propagate to many other body areas by disrupting regular cellular mechanisms such as DNA synthesis, cell division, and suicide, resulting in fatal disorders193. The basic cancer treatments are surgery, radiotherapy, chemotherapy, and adjuvant medicines such as biological gene or hormone therapies194. TCS's initial particular action strategy in prokaryotic cells was only discovered twenty years ago when it was shown that TCS inhibited fatty acid production in E. coli 195. By replicating its native substrate in vivo, TCS blocked the fatty acid manufacturing enzyme enoyl–ACP reductase permanently (Figure 2). TCS resistance in the bacteria was also conferred by a mutant or overexposed ACP expressed by fabI. ACP be identified as an intracellular TCS goal as a result of these studies. Cerulenin, mycotoxin effectiveness that inhibits fat formation in inhibiting tumour development in vivo has inspired multiple papers in favour of fat formulation suppression as a new approach for chemotherapy196. FAS appearance and action vary in normal and cancerous tissues, with the latter being increased, implying a potentially high therapeutic index. TCS is a good option for chemotherapy because of its long history of human use and widespread presence in consumer goods, as well as promising in vivo outcomes197.
Phytoestrogens generated from vegetables and fruits have long been considered alternative remedies for human disorders as natural chemicals. Phytoestrogens as hormone replacement therapy (HRT) are used in protracted therapies to prevent oestrogen-responsive malignancies, such as bosom cancer198. Polyphenolic substances, such as flavonoids, have been shown in vitro and in vivo to limit bosom cancer cell proliferation by challenging with 17β-oestradiol (E2) for ER attraction sites199 as shown in Figure 2. Further comprehensive underlying mechanisms have to be researched as interest in phytoestrogens' positive effects rises200. Flavonoid-based phytoestrogen kaempferol occurs mainly in fruit and plants like apples, tomatoes, and green tea29. The anti-cancer and osteosarcoma effect of kaempferol has been described in current studies192. It has been reported in many studies that extensive usage of TCS can have cancerogenic potential, as shown in Table 1. Significant research has also highlighted the mechanisms involved in kaempferol anticancer action associated with the cell cycle, cell death and angiogenic, inflammatory reactions, and oxygen radicals production201. In U-2 OS human osteogenic sarcoma cells, kaempferol also reduced the appearance of ERK, JNK, and p38202. Kaempferol inhibited the PI3K/Akt signal transduction by adhering directly to PI3K and inhibiting the capabilities of AP-1, and protein kinase b (NF-β), which affect a variety of cellular activities such as growth, angiogenesis, and death203. According to an in vitro study, TCS can enhance the growth of BG-1 ovarian cancer cells by modulating the expression of cell cycle and death genes19.
To investigate for signalling-related genes in MCF-7 cells treated with Dimethyl sulfoxide (vehicle), E2 (109 M), TCS (106 M), and a blend of kaempferol (50 M) and TCS, we conducted western blotting on specimens of proteins recovered from the cells204. Given the discoveries, E2 was up-regulated. IRS-1, PKB, MAPK, and phosphorylated versions of IRS-1 are all expressed in phosphorylated forms (Figure 2). The major proteins of insulin-like growth factor type 1 receptor (IGF-1R) signalling are the ERK proteins. E2 stimulates MCF-7 cell growth through both the ER and IGF-1R signalling pathways. E2 and TCS were used to see how they affected IGF expression (Figure 2). TCS, like E2, was found to exhibit an estrogenic effect by modulating the protein appearance of pIRS-1, PKB, MAPK, and ERK. Kaempferol also had an antiestrogenic impact by inhibiting the protein production of pIRS-1, PKB, MAPK, and ERK, which were all induced by E2 or TCS30. As previously stated, changes in the result of TCS therapy are primarily dependent on the experimental setup. Furthermore, limited evidence from animal research suggests that TCS treatment exacerbates the disease in the existence of a previously established tumour29.
FUTURE PERSPECTIVE
TCS is not effectively controlled, as seen in numerous environmental media, human bodies, and wildlife. Its reckless usage and disposal may endanger humans and the ecology as a whole. TCS has been demonstrated to be harmful to a variety of cells in cell-based investigations. Cell-based assays are time-limited and so cannot rightly examine the consequence of persistent expose. TCS recognition in human fluid or tissue may not have been a reliable predictor of the extended period because the evidence on its bioaccumulation in the flesh is lacking205.
Furthermore, TCS is considered to block enzymes involved in its decomposition206. There is currently a scarcity of information about TCS's pharmacokinetics and pharmacodynamics. Enough knowledge would allow for more flexibility in determining TCS's toxicity. However, its anti-growth influence has been noted in certain malignant cells, the toxicological importance of TCS' inhibitory impact on human FAS is not fully grasped104. TCS has been found in significant amounts in mammalian tissues, raising the likelihood that the molecule has an adverse effect on mammalian anatomy. Its negative impact on birth resistance has been documented, as clinical reports claim it can treat human allergic skin conditions207.
TCS's exact function in selecting antibiotic resistance genes and many drugs protection genetic codes in the surroundings remains resolute. The level of the TCS needed for ecological tolerance choices also needs to be determined. The correlation between TCS introduction and bioaccumulation in an earthly organism is still unclear208. More research is needed, such as the movement of soil-based TCS processing and earth organisms absorption, including Invertebrates and slug, essential to crop production and diet208. TCS increased MCF-7 bosom cancer reproduction via modulating cell division, death, and tumour-linked genetic codes through nongenomic ER signalling linked to IGF-1R signalling.
On the other hand, Kaempferol showed an anti-proliferative effect against bosom cancer by inhibiting TCS and E2-induced cancer growth by serving as a competitor for ER and IGF-1R signaling. It's the first research to demonstrate that kaempferol has anticancer action against the pro-cancer action of endogenous oestrogen and xenoestrogen in bosom cancer. It also recommends kaempferol as a major drug to modify TCS-induced malignancy hazard30. Future research should concentrate on recognizing TCS-controlled signaling molecules and their responsibility for poisonous or preventive effects of different cell types. The information obtained from such revelations will be invaluable in validating therapeutic strategies or developing potential TCS adjuvants or blockers209. In ongoing studies, animal and human growth investigations and mammalian experiments with susceptible endocrine/reproductive outcomes should be included. Systematic evaluations of these areas, particularly through deriving human health risk inferences from TCS consequences, can enable upcoming studies and regulations to safeguard people's health more efficiently210.
CONCLUSION
TCS is a synthesized antimicrobic that has been used in humans for a long time. Humans are subjected to TCS as a result of environmental and consumer good consumption. TCS exposure can cause various problems, including thyroid dysfunction, liver tumorigenesis, endocrine disruption, growth issues, muscle weakness, and oxidative stress. It is also necessary to estimate the TCS level required for tolerance choice in environmental groups. Precocious puberty and carcinogenicity could be produced by receptor overstimulation, presumably caused by the high TCS level. TCS has been linked to allergic reactions in people, according to certain research. TCS is a type 1 FAS enoyl-reductase inhibitor that has been proven to be effective in cultured cells against the MCF-7 and SK Br-3 bosom cancer cell lines. Because of its long history of human usage and ubiquitous prevalence in consumer items, as well as promising in vivo results, TCS is a viable alternative for chemotherapy.
According to extensive studies, the kaempferol anticancer effect has also been linked to the cell cycle, cell death and angiogenic, inflammatory reactions, and the formation of oxygen radicals. By adhering directly to PI3K and limiting the capacities of protein kinase b (NF-β) and AP-1, kaempferol suppressed the PI3K/Akt signal transduction, which affects a range of cellular activities such as growth, angiogenesis, and death. TCS's environmental survivability in sediments demonstrates that antimicrobial compounds can spread and endure breakdown mechanisms in anaerobic conditions. In addition, hydrogen peroxide speeds up the oxidation process. This system contains enzymatic and nonenzymatic antioxidants such CAT, SOD, GPx, GR, GSH, and GST. As a result, ecologists may examine the amount of oxidative stress caused in organisms exposed to specific substances by measuring antioxidant levels. The current study's lower GSH/GSSG levels might be explained because GSH could not be restored to its standard concentration in the liver after introducing TCS concentrations due to impaired GPx and GR actions.
Abbreviations
2,4-DCP: 2,4-dichlorophenol; 2,8-DCDD: 2,8-dichlorodibenzo-p-dioxin; A. difformis: Anchomanes difformis; A. tumefaciens: Agrobacterium tumefaciens; APND: aminopyrine N-demethylase; AR: androgen receptor; B. subtilis: Bacillus subtilis; β-hCG: β-human chorionic gonadotropin; CAT: Catalase; COX-1: cyclooxygenase-1; CTD: chlorinated TCS derivative; D. magna: Daphnia magna; E. coli: Escherichia coli; Enoyl-ACP: acyl carrier protein; EE: ethinylestradiol; EMT: epithelial-to-mesenchymal transition; ER: estrogen-receptor; ERK: extracellular signal-regulated kinase; EROD: ethoxyresorufin-O-deethylase; ERND: erythromycin N-demethylase; FAS: enoyl-fatty acid synthesis; FSH: follicle-stimulating hormone; GAPDH: glyceraldehyde 3-phosphate dehydrogenase; GPx: Glutathione peroxidase; GR: Glutathione reductase; GSH: Glutathione; GSSG: Glutathione disulfide; GST: glutathione S-transferase; HBSS: hank's balanced salt solution; HRT: hormone replacement therapy; HS: hidradenitis suppurativa; hsp-70: heat shock protein 70; IL-1β: Interleukin-1β; JNK: Jun N-terminal kinases; LFC: log2 fold change; LH: luteinizing hormone; LPO: Lipid peroxidation; LPS: lipopolysaccharides; M. smegmatis: Mycobacterium smegmatis; M. tuberculosis: Mycobacterium tuberculosis; MAPK: mitogen-activated protein kinase; MCF-7: Michigan Cancer Foundation-7; MDA: Malondialdehyde; MHC-II: Major histocompatibility complex class II; miRNA: MicroRNA; MN: micronucleus; MMP-13: matrix metalloproteinase-13; NA: nuclear abnormalities; Nik: NF-B inducing kinase; NMR: nuclear magnetic resonance; P. aeruginosa: Pseudomonas aeruginosa; P. falciparum: Plasmodium falciparum; PGES-1: Prostaglandin E synthase-1; PGE2: prostaglandin E2; PGI2: prostaglandin I2; PI3K: phosphoinositide 3-kinase; pIRS-1: phosphorylated insulin receptor substrate-1; PKB: protein kinase B; PLA2: phospholipase A2; PTH: parathyroid hormone; R. rubrum: Rhodospirillum rubrum; ROS: Reactive oxygen species; RyR1: ryanodine receptor type 1; S. aureus: Staphylococcus aureus; S. pneumoniae: Staphylococcus pneumoniae; S. typhimurium: Salmonella typhimurium; SAM: S-adenosylmethionine; SOD: Superoxide dismutase; T. gondii: Toxoplasma gondii; T. thermopohila: Tetrahymena thermophila; TAC: Total antioxidant capacity; TCS: Triclosan; TK: thymidine kinase; TLR-4: Toll-like receptor-4; TMP: trimethoprim; TPO: thyroid peroxidase; TRPA1: Transient Receptor Potential Ankyrin 1; TSN: Testosterone; UTI: urinary tract infection; VEGF: vascular endothelial growth factor.
Acknowledgments
The authors concede the support of the Cholistan University of Veterinary & Animal Sciences-Bahawalpur, Pakistan, during the write-up.
Author’s contributions
Kamal Niaz: Conceptualization, outlines and editing; Furqan Shafqat, Shafeeq Ur Rehman, and Muhammad Usman: Data curation, writing- original draft preparation and drawing figures; Kamal Niaz: Supervision, reviewing and editing. All authors read and approved the final manuscript.
Funding
None.
Availability of data and materials
Not applicable.
Ethics approval and consent to participate
Not applicable.
Consent for publication
Not applicable.
Competing interests
The authors declare that they have no competing interests.
References
-
Dhillon
G.S.,
Kaur
S.,
Pulicharla
R.,
Brar
S.K.,
Cledón
M.,
Verma
M.,
Triclosan: current status, occurrence, environmental risks and bioaccumulation potential. International Journal of Environmental Research and Public Health.
2015;
12
(5)
:
5657-84
.
View Article PubMed Google Scholar -
Hipwell
A.E.,
Kahn
L.G.,
Factor-Litvak
P.,
Porucznik
C.A.,
Siegel
E.L.,
Fichorova
R.N.,
Exposure to non-persistent chemicals in consumer products and fecundability: a systematic review. Human Reproduction Update.
2018;
25
(1)
:
51-71
.
View Article PubMed Google Scholar -
Escalada
M.G.,
Harwood
J.L.,
Maillard
J.Y.,
Ochs
D.,
Triclosan inhibition of fatty acid synthesis and its effect on growth of Escherichia coli and Pseudomonas aeruginosa. The Journal of Antimicrobial Chemotherapy.
2005;
55
(6)
:
879-82
.
View Article PubMed Google Scholar -
Khan
R.,
Kong
H.G.,
Jung
Y.H.,
Choi
J.,
Baek
K.Y.,
Hwang
E.C.,
Triclosan resistome from metagenome reveals diverse enoyl acyl carrier protein reductases and selective enrichment of triclosan resistance genes. Scientific Reports.
2016;
6
(1)
:
32322
.
View Article PubMed Google Scholar -
Fan
C.,
Zhou
M.,
Tang
X.,
Zeng
G.,
Xu
Q.,
Song
B.,
Triclosan enhances short-chain fatty acid production from sludge fermentation by elevating transcriptional activity of acidogenesis bacteria. Chemical Engineering Journal.
2020;
384
:
123285
.
View Article Google Scholar -
Latch
D.E.,
Packer
J.L.,
Stender
B.L.,
VanOverbeke
J.,
Arnold
W.A.,
McNeill
K.,
Aqueous photochemistry of triclosan: formation of 2,4-dichlorophenol, 2,8-dichlorodibenzo-p-dioxin, and oligomerization products. Environmental Toxicology and Chemistry.
2005;
24
(3)
:
517-25
.
View Article PubMed Google Scholar -
Sánchez-Prado
L.,
Llompart
M.,
Lores
M.,
Fernández-Alvarez
M.,
García-Jares
C.,
Cela
R.,
Further research on the photo-SPME of triclosan. Analytical and Bioanalytical Chemistry.
2006;
384
(7-8)
:
1548-57
.
View Article PubMed Google Scholar -
Son
H.S.,
Choi
S.B.,
Zoh
K.D.,
Khan
E.,
Effects of ultraviolet intensity and wavelength on the photolysis of triclosan. Water Science and Technology.
2007;
55
(1-2)
:
209-16
.
View Article PubMed Google Scholar -
Wang
F.,
Xu
R.,
Zheng
F.,
Liu
H.,
Effects of triclosan on acute toxicity, genetic toxicity and oxidative stress in goldfish (Carassius auratus). Experimental Animals.
2018;
67
(2)
:
219-227
.
View Article PubMed Google Scholar -
Park
J.C.,
Han
J.,
Lee
M.C.,
Seo
J.S.,
Lee
J.S.,
Effects of triclosan (TCS) on fecundity, the antioxidant system, and oxidative stress-mediated gene expression in the copepod Tigriopus japonicus. Aquatic Toxicology (Amsterdam, Netherlands).
2017;
189
:
16-24
.
View Article PubMed Google Scholar -
Ne
A.,
Hm
A.E.,
Ha
E.,
Ar
M.,
A
NE,
Effect of oxymatrine on triclosan-induced ovarian toxicity in female rats. Egyptian Journal of Occupational Medicine.
2021;
45
(2)
:
101-16
.
View Article Google Scholar -
Aliyu
A.B.,
Ibrahim
M.A.,
Musa
A.M.,
Musa
A.O.,
Kiplimo
J.J.,
Oyewale
A.O.,
Free radical scavenging and total antioxidant capacity of root extracts of Anchomanes difformis Engl. (Araceae). Acta Poloniae Pharmaceutica.
2013;
70
(1)
:
115-21
.
PubMed Google Scholar -
Benzie
I.F.,
Strain
J.J.,
The ferric reducing ability of plasma (FRAP) as a measure of antioxidant power right: the FRAP assay. Analytical Biochemistry.
1996;
239
(1)
:
70-6
.
View Article PubMed Google Scholar -
Alfhili
M.A.,
Lee
M.-H.,
Triclosan: an update on biochemical and molecular mechanisms. Oxid. Med. Cell. Longev.
2019;
2019
:
1607304
.
View Article Google Scholar -
Mustafa
M.,
Bakhiet
M.,
Wondimu
B.,
Modéer
T.,
Effect of triclosan on interferon-γ production and major histocompatibility complex class II expression in human gingival fibroblasts. Journal of Clinical Periodontology.
2000;
27
(10)
:
733-7
.
View Article PubMed Google Scholar -
Dinwiddie
M.T.,
Terry
P.D.,
Chen
J.,
Recent evidence regarding triclosan and cancer risk. International Journal of Environmental Research and Public Health.
2014;
11
(2)
:
2209-17
.
View Article PubMed Google Scholar -
Xue
J.,
Wu
Q.,
Sakthivel
S.,
Pavithran
P.V.,
Vasukutty
J.R.,
Kannan
K.,
Urinary levels of endocrine-disrupting chemicals, including bisphenols, bisphenol A diglycidyl ethers, benzophenones, parabens, and triclosan in obese and non-obese Indian children. Environmental Research.
2015;
137
:
120-8
.
View Article PubMed Google Scholar -
Di Donato
M.,
Cernera
G.,
Giovannelli
P.,
Galasso
G.,
Bilancio
A.,
Migliaccio
A.,
Recent advances on bisphenol-A and endocrine disruptor effects on human prostate cancer. Molecular and Cellular Endocrinology.
2017;
457
:
35-42
.
View Article PubMed Google Scholar -
Kim
J.Y.,
Yi
B.R.,
Go
R.E.,
Hwang
K.A.,
Nam
K.H.,
Choi
K.C.,
Methoxychlor and triclosan stimulates ovarian cancer growth by regulating cell cycle- and apoptosis-related genes via an estrogen receptor-dependent pathway. Environmental Toxicology and Pharmacology.
2014;
37
(3)
:
1264-74
.
View Article PubMed Google Scholar -
Hwang
K.A.,
Park
M.A.,
Kang
N.H.,
Yi
B.R.,
Hyun
S.H.,
Jeung
E.B.,
Anticancer effect of genistein on BG-1 ovarian cancer growth induced by 17 β-estradiol or bisphenol A via the suppression of the crosstalk between estrogen receptor α and insulin-like growth factor-1 receptor signaling pathways. Toxicology and Applied Pharmacology.
2013;
272
(3)
:
637-46
.
View Article PubMed Google Scholar -
Rodricks
J.V.,
Swenberg
J.A.,
Borzelleca
J.F.,
Maronpot
R.R.,
Shipp
A.M.,
Triclosan: a critical review of the experimental data and development of margins of safety for consumer products. Critical Reviews in Toxicology.
2010;
40
(5)
:
422-84
.
View Article PubMed Google Scholar -
Yueh
M.F.,
Tukey
R.H.,
Triclosan: a widespread environmental toxicant with many biological effects. Annual Review of Pharmacology and Toxicology.
2016;
56
(1)
:
251-72
.
View Article PubMed Google Scholar -
Wang
C.,
Chen
L.,
Zhao
S.,
Hu
Y.,
Zhou
Y.,
Gao
Y.,
Impacts of prenatal triclosan exposure on fetal reproductive hormones and its potential mechanism. Environment International.
2018;
111
:
279-86
.
View Article PubMed Google Scholar -
Calafat
A.M.,
Ye
X.,
Wong
L.Y.,
Reidy
J.A.,
Needham
L.L.,
Urinary concentrations of triclosan in the U.S. population: 2003-2004. Environmental Health Perspectives.
2008;
116
(3)
:
303-7
.
View Article PubMed Google Scholar -
Yang
H.,
Wang
W.,
Romano
K.A.,
Gu
M.,
Sanidad
K.Z.,
Kim
D.,
A common antimicrobial additive increases colonic inflammation and colitis-associated colon tumorigenesis in mice. Science Translational Medicine.
2018;
10
(443)
.
View Article PubMed Google Scholar -
Ahn
K.C.,
Zhao
B.,
Chen
J.,
Cherednichenko
G.,
Sanmarti
E.,
Denison
M.S.,
In vitro biologic activities of the antimicrobials triclocarban, its analogs, and triclosan in bioassay screens: receptor-based bioassay screens. Environmental Health Perspectives.
2008;
116
(9)
:
1203-10
.
View Article PubMed Google Scholar -
Nikitkova
A.E.,
Haase
E.M.,
Vickerman
M.M.,
Gill
S.R.,
Scannapieco
F.A.,
Response of fatty acid synthesis genes to the binding of human salivary amylase by Streptococcus gordonii. Applied and Environmental Microbiology.
2012;
78
(6)
:
1865-75
.
View Article PubMed Google Scholar -
Sadowski
M.C.,
Pouwer
R.H.,
Gunter
J.H.,
Lubik
A.A.,
Quinn
R.J.,
Nelson
C.C.,
The fatty acid synthase inhibitor triclosan: repurposing an anti-microbial agent for targeting prostate cancer. Oncotarget.
2014;
5
(19)
:
9362-81
.
View Article PubMed Google Scholar -
Lee
G.A.,
Choi
K.C.,
Hwang
K.A.,
Treatment with phytoestrogens reversed triclosan and bisphenol A-induced anti-apoptosis in breast cancer cells. Biomolecules {&}amp; Therapeutics.
2018;
26
(5)
:
503-11
.
View Article PubMed Google Scholar -
Kim
S.H.,
Hwang
K.A.,
Choi
K.C.,
Treatment with kaempferol suppresses breast cancer cell growth caused by estrogen and triclosan in cellular and xenograft breast cancer models. The Journal of Nutritional Biochemistry.
2016;
28
:
70-82
.
View Article PubMed Google Scholar -
Liu
B.,
Wang
Y.,
Fillgrove
K.L.,
Anderson
V.E.,
Triclosan inhibits enoyl-reductase of type I fatty acid synthase in vitro and is cytotoxic to MCF-7 and SKBr-3 breast cancer cells. Cancer Chemotherapy and Pharmacology.
2002;
49
(3)
:
187-93
.
View Article PubMed Google Scholar -
Vandhana
S.,
Coral
K.,
Jayanthi
U.,
Deepa
P.R.,
Krishnakumar
S.,
Biochemical changes accompanying apoptotic cell death in retinoblastoma cancer cells treated with lipogenic enzyme inhibitors. Biochimica et Biophysica Acta.
2013;
1831
(9)
:
1458-66
.
View Article PubMed Google Scholar -
Henry
N.D.,
Fair
P.A.,
Comparison of in vitro cytotoxicity, estrogenicity and anti-estrogenicity of triclosan, perfluorooctane sulfonate and perfluorooctanoic acid. Journal of Applied Toxicology.
2013;
33
(4)
:
265-72
.
View Article PubMed Google Scholar -
Dann
A.B.,
Hontela
A.,
Triclosan: environmental exposure, toxicity and mechanisms of action. Journal of Applied Toxicology.
2011;
31
(4)
:
285-311
.
View Article PubMed Google Scholar -
Deepa
P.R.,
Vandhana
S.,
Muthukumaran
S.,
Umashankar
V.,
Jayanthi
U.,
Krishnakumar
S.,
Chemical inhibition of fatty acid synthase: molecular docking analysis and biochemical validation in ocular cancer cells. Journal of Ocular Biology, Diseases, and Informatics.
2010;
3
(4)
:
117-28
.
View Article PubMed Google Scholar -
Deepa
P.R.,
Vandhana
S.,
Jayanthi
U.,
Krishnakumar
S.,
Therapeutic and toxicologic evaluation of anti-lipogenic agents in cancer cells compared with non-neoplastic cells. Basic {&}amp; Clinical Pharmacology {&}amp; Toxicology.
2012;
110
(6)
:
494-503
.
View Article PubMed Google Scholar -
Rana
P.,
Ghouse
S.M.,
Akunuri
R.,
Madhavi
Y.V.,
Chopra
S.,
Nanduri
S.,
FabI (enoyl acyl carrier protein reductase) - A potential broad spectrum therapeutic target and its inhibitors. European Journal of Medicinal Chemistry.
2020;
208
:
112757
.
View Article PubMed Google Scholar -
Maiden
M.M.,
Hunt
A.M.,
Zachos
M.P.,
Gibson
J.A.,
Hurwitz
M.E.,
Mulks
M.H.,
Triclosan is an aminoglycoside adjuvant for eradication of Pseudomonas aeruginosa biofilms. Antimicrobial Agents and Chemotherapy.
2018;
62
(6)
:
e00146-18
.
View Article PubMed Google Scholar -
Yogiara
Mordukhova
E.A.,
Kim
D.,
Kim
W.G.,
Hwang
J.K.,
Pan
J.G.,
The food-grade antimicrobial xanthorrhizol targets the enoyl-ACP reductase (FabI) in Escherichia coli. Bioorganic {&}amp; Medicinal Chemistry Letters.
2020;
30
(24)
:
127651
.
View Article PubMed Google Scholar -
Vosátka
R.,
Krátký
M.,
Vinšová
J.,
Triclosan and its derivatives as antimycobacterial active agents. European Journal of Pharmaceutical Sciences.
2018;
114
:
318-31
.
View Article PubMed Google Scholar -
Taira
J.,
Umei
T.,
Inoue
K.,
Kitamura
M.,
Berenger
F.,
Sacchettini
J.C.,
Improvement of the novel inhibitor for Mycobacterium enoyl-acyl carrier protein reductase (InhA): a structure-activity relationship study of KES4 assisted by in silico structure-based drug screening. The Journal of Antibiotics.
2020;
73
(6)
:
372-81
.
View Article PubMed Google Scholar -
Bilsland
E.,
van Vliet
L.,
Williams
K.,
Feltham
J.,
Carrasco
M.P.,
Fotoran
W.L.,
Plasmodium dihydrofolate reductase is a second enzyme target for the antimalarial action of triclosan. Scientific Reports.
2018;
8
(1)
:
1038
.
View Article PubMed Google Scholar -
Rae
C.,
Fragkoulis
G.I.,
Chalmers
A.J.,
Cytotoxicity and radiosensitizing activity of the fatty acid synthase inhibitor C75 is enhanced by blocking fatty acid uptake in prostate cancer cells. Advances in Radiation Oncology.
2020;
5
(5)
:
994-1005
.
View Article PubMed Google Scholar -
Singer
H.,
Müller
S.,
Tixier
C.,
Pillonel
L.,
Triclosan: occurrence and fate of a widely used biocide in the aquatic environment: field measurements in wastewater treatment plants, surface waters, and lake sediments. Environmental Science & Technology.
2002;
36
(23)
:
4998-5004
.
View Article PubMed Google Scholar -
Miller
T.R.,
Heidler
J.,
Chillrud
S.N.,
DeLaquil
A.,
Ritchie
J.C.,
Mihalic
J.N.,
Fate of triclosan and evidence for reductive dechlorination of triclocarban in estuarine sediments. Environmental Science & Technology.
2008;
42
(12)
:
4570-6
.
View Article PubMed Google Scholar -
Hedman
J.E.,
Tocca
J.S.,
Gunnarsson
J.S.,
Remobilization of polychlorinated biphenyl from Baltic Sea sediment: comparing the roles of bioturbation and physical resuspension. Environmental Toxicology and Chemistry.
2009;
28
(11)
:
2241-9
.
View Article PubMed Google Scholar -
Ingerslev
F.,
Vaclavik
E.,
Halling-S∅rensen
B.,
Pharmaceuticals and personal care products-A source of endocrine disruption in the environment?. Pure and Applied Chemistry.
2003;
75
(11-12)
:
1881-93
.
View Article Google Scholar -
Iovino
P.,
Chianese
S.,
Prisciandaro
M.,
Musmarra
D.,
Triclosan photolysis: operating condition study and photo-oxidation pathway. Chemical Engineering Journal.
2019;
377
:
121045
.
View Article Google Scholar -
Fiss
E.M.,
Rule
K.L.,
Vikesland
P.J.,
Formation of chloroform and other chlorinated byproducts by chlorination of triclosan-containing antibacterial products. Environmental Science & Technology.
2007;
41
(7)
:
2387-94
.
View Article PubMed Google Scholar -
Greyshock
A.E.,
Vikesland
P.J.,
Triclosan reactivity in chloraminated waters. Environmental Science & Technology.
2006;
40
(8)
:
2615-22
.
View Article PubMed Google Scholar -
Nabeshima
Y.,
Hasegawa
J.,
Matsuda
M.,
Kawano
M.,
Wakimoto
T.,
Morita
M.,
Determination of triclosan and its related compounds in aquatic environment. Organohalogen Compounds.
2007;
69
:
1503-6
.
-
Leiker
T.J.,
Abney
S.R.,
Goodbred
S.L.,
Rosen
M.R.,
Identification of methyl triclosan and halogenated analogues in male common carp (Cyprinus carpio) from Las Vegas Bay and semipermeable membrane devices from Las Vegas Wash, Nevada. The Science of the Total Environment.
2009;
407
(6)
:
2102-14
.
View Article PubMed Google Scholar -
Kanetoshi
A.,
Katsura
E.,
Ogawa
H.,
Ohyama
T.,
Kaneshima
H.,
Miura
T.,
Acute toxicity, percutaneous absorption and effects on hepatic mixed function oxidase activities of 2,4,4'-trichloro-2'-hydroxydiphenyl ether (Irgasan DP300) and its chlorinated derivatives. Archives of Environmental Contamination and Toxicology.
1992;
23
(1)
:
91-8
.
View Article PubMed Google Scholar -
Buth
J.M.,
Grandbois
M.,
Vikesland
P.J.,
McNeill
K.,
Arnold
W.A.,
Aquatic photochemistry of chlorinated triclosan derivatives: potential source of polychlorodibenzo-p-dioxins. Environmental Toxicology and Chemistry.
2009;
28
(12)
:
2555-63
.
View Article PubMed Google Scholar -
Lee
G.F.,
Morris
J.C.,
Kinetics of chlorination of phenolchlorophenolic tastes and odors. Air and Water Pollution.
1962;
6
(567)
:
419-31
.
PubMed Google Scholar -
Gallard
H.,
von
G.U.,
Chlorination of phenols: kinetics and formation of chloroform. Environmental Science {&}amp; Technology.
2002;
36
(5)
:
884-90
.
View Article PubMed Google Scholar -
Lopez-Avila
V.,
Hites
R.A.,
Organic compounds in an industrial wastewater. Their transport into sediments. Environmental Science {&}amp; Technology.
1980;
14
(11)
:
1382-90
.
View Article Google Scholar -
Meade
M.J.,
Waddell
R.L.,
Callahan
T.M.,
Soil bacteria Pseudomonas putida and Alcaligenes xylosoxidans subsp. denitrificans inactivate triclosan in liquid and solid substrates. FEMS Microbiology Letters.
2001;
204
(1)
:
45-8
.
View Article PubMed Google Scholar -
Neilson
A.H.,
Allard
A.S.,
Hynning
P.\AA.,
Remberger
M.,
Landner
L.,
Bacterial methylation of chlorinated phenols and guaiacols: formation of veratroles from guaiacols and high-molecular-weight chlorinated lignin. Applied and Environmental Microbiology.
1983;
45
(3)
:
774-83
.
View Article PubMed Google Scholar -
Field
J.A.,
Sierra-Alvarez
R.,
Microbial degradation of chlorinated dioxins. Chemosphere.
2008;
71
(6)
:
1005-18
.
View Article PubMed Google Scholar -
Son
H.S.,
Ko
G.,
Zoh
K.D.,
Kinetics and mechanism of photolysis and TiO2 photocatalysis of triclosan. Journal of Hazardous Materials.
2009;
166
(2-3)
:
954-60
.
View Article PubMed Google Scholar -
Yu
J.C.,
Kwong
T.Y.,
Luo
Q.,
Cai
Z.,
Photocatalytic oxidation of triclosan. Chemosphere.
2006;
65
(3)
:
390-9
.
View Article PubMed Google Scholar -
Coogan
M.A.,
La Point
T.W.,
Snail bioaccumulation of triclocarban, triclosan, and methyltriclosan in a North Texas, USA, stream affected by wastewater treatment plant runoff. Environmental Toxicology and Chemistry.
2008;
27
(8)
:
1788-93
.
View Article PubMed Google Scholar -
Boehmer
W.,
Ruedel
H.,
Wenzel
A.,
Schroeter-Kermani
C.,
Retrospective monitoring of triclosan and methyl-triclosan in fish. Results from the German Environmental Specimen Bank. Chemosphere.
2004
.
-
Wang
C.,
Huang
W.,
Lin
J.,
Fang
F.,
Wang
X.,
Wang
H.,
Triclosan-induced liver and brain injury in zebrafish (Danio rerio) via abnormal expression of miR-125 regulated by PKCα/Nrf2/p53 signaling pathways. Chemosphere.
2020;
241
:
125086
.
View Article PubMed Google Scholar -
Liu
T.,
Zhu
L.,
Han
Y.,
Wang
J.,
Wang
J.,
Zhao
Y.,
The cytotoxic and genotoxic effects of metalaxy-M on earthworms (Eisenia fetida). Environmental Toxicology and Chemistry.
2014;
33
(10)
:
2344-50
.
View Article PubMed Google Scholar -
Gonzalez-Hunt
C.P.,
Wadhwa
M.,
Sanders
L.H.,
DNA damage by oxidative stress: measurement strategies for two genomes. Current Opinion in Toxicology.
2018;
7
:
87-94
.
View Article Google Scholar -
Ni
H.,
Peng
L.,
Gao
X.,
Ji
H.,
Ma
J.,
Li
Y.,
Effects of maduramicin on adult zebrafish (Danio rerio): acute toxicity, tissue damage and oxidative stress. Ecotoxicology and Environmental Safety.
2019;
168
:
249-59
.
View Article PubMed Google Scholar -
Wu
M.,
Xu
H.,
Shen
Y.,
Qiu
W.,
Yang
M.,
Oxidative stress in zebrafish embryos induced by short-term exposure to bisphenol A, nonylphenol, and their mixture. Environmental Toxicology and Chemistry.
2011;
30
(10)
:
2335-41
.
View Article PubMed Google Scholar -
Gomes
M.F.,
de Carvalho Soares de Paula
V.,
Rocha Martins
L.R.,
Esquivel Garcia
J.R.,
Yamamoto
F.Y.,
Martins de Freitas
A.,
Sublethal effects of triclosan and triclocarban at environmental concentrations in silver catfish (Rhamdia quelen) embryos. Chemosphere.
2021;
263
:
127985
.
View Article PubMed Google Scholar -
Bao
S.,
He
C.,
Ku
P.,
Xie
M.,
Lin
J.,
Lu
S.,
Effects of triclosan on the RedoximiRs/Sirtuin/Nrf2/ARE signaling pathway in mosquitofish (Gambusia affinis). Aquatic Toxicology (Amsterdam, Netherlands).
2021;
230
:
105679
.
View Article PubMed Google Scholar -
Maulvault
A.L.,
Camacho
C.,
Barbosa
V.,
Alves
R.,
Anacleto
P.,
Cunha
S.C.,
Bioaccumulation and ecotoxicological responses of juvenile white seabream (Diplodus sargus) exposed to triclosan, warming and acidification. Environmental Pollution.
2019;
245
:
427-42
.
View Article PubMed Google Scholar -
Butterfield
D.A.,
Koppal
T.,
Howard
B.,
Subramaniam
R.,
Hall
N.,
Hensley
K.,
Structural and functional changes in proteins induced by free radical-mediated oxidative stress and protective action of the antioxidants N-tert-butyl-α-phenylnitrone and vitamin E. Annals of the New York Academy of Sciences.
1998;
854
(1)
:
448-62
.
View Article PubMed Google Scholar -
Gyimah
E.,
Dong
X.,
Qiu
W.,
Zhang
Z.,
Xu
H.,
Sublethal concentrations of triclosan elicited oxidative stress, DNA damage, and histological alterations in the liver and brain of adult zebrafish. Environmental Science and Pollution Research International.
2020;
27
(14)
:
17329-38
.
View Article PubMed Google Scholar -
Elia
A.C.,
Galarini
R.,
Taticchi
M.I.,
Dörr
A.J.,
Mantilacci
L.,
Antioxidant responses and bioaccumulation in Ictalurus melas under mercury exposure. Ecotoxicology and Environmental Safety.
2003;
55
(2)
:
162-7
.
View Article PubMed Google Scholar -
Freitas
R.,
Coppola
F.,
Costa
S.,
Pretti
C.,
Intorre
L.,
Meucci
V.,
The influence of temperature on the effects induced by Triclosan and Diclofenac in mussels. The Science of the Total Environment.
2019;
663
:
992-9
.
View Article PubMed Google Scholar -
Sun
C.,
Dudley
S.,
Wang
J.,
Gan
J.,
Nitric oxide regulates triclosan-induced redox disequilibrium by enhancing glutathione metabolism in wheat seedlings. Environmental Science & Technology Letters.
2019;
6
(5)
:
313-7
.
View Article Google Scholar -
Oliveira
R.,
Domingues
I.,
Koppe Grisolia
C.,
Soares
A.M.,
Effects of triclosan on zebrafish early-life stages and adults. Environmental Science and Pollution Research International.
2009;
16
(6)
:
679-88
.
View Article PubMed Google Scholar -
Falisse
E.,
Voisin
A.S.,
Silvestre
F.,
Impacts of triclosan exposure on zebrafish early-life stage: toxicity and acclimation mechanisms. Aquatic Toxicology (Amsterdam, Netherlands).
2017;
189
:
97-107
.
View Article PubMed Google Scholar -
Chen
Q.L.,
Sun
Y.L.,
Liu
Z.H.,
Li
Y.W.,
Sex-dependent effects of subacute mercuric chloride exposure on histology, antioxidant status and immune-related gene expression in the liver of adult zebrafish (Danio rerio). Chemosphere.
2017;
188
:
1-9
.
View Article PubMed Google Scholar -
Lin
D.,
Zhou
Q.,
Xie
X.,
Liu
Y.,
Potential biochemical and genetic toxicity of triclosan as an emerging pollutant on earthworms (Eisenia fetida). Chemosphere.
2010;
81
(10)
:
1328-33
.
View Article PubMed Google Scholar -
Li
C.,
Qu
R.,
Chen
J.,
Zhang
S.,
Allam
A.A.,
Ajarem
J.,
The pH-dependent toxicity of triclosan to five aquatic organisms (Daphnia magna, Photobacterium phosphoreum, Danio rerio, Limnodrilus hoffmeisteri, and Carassius auratus). Environmental Science and Pollution Research International.
2018;
25
(10)
:
9636-46
.
View Article PubMed Google Scholar -
Peng
Y.,
Luo
Y.,
Nie
X.P.,
Liao
W.,
Yang
Y.F.,
Ying
G.G.,
Toxic effects of triclosan on the detoxification system and breeding of Daphnia magna. Ecotoxicology (London, England).
2013;
22
(9)
:
1384-94
.
View Article PubMed Google Scholar -
Kovacevic
V.,
Simpson
A.J.,
Simpson
M.J.,
(1)H NMR-based metabolomics of Daphnia magna responses after sub-lethal exposure to triclosan, carbamazepine and ibuprofen. Comparative Biochemistry and Physiology. Part D, Genomics {&}amp; Proteomics.
2016;
19
:
199-210
.
View Article PubMed Google Scholar -
Riva
C.,
Cristoni
S.,
Binelli
A.,
Effects of triclosan in the freshwater mussel Dreissena polymorpha: a proteomic investigation. Aquatic Toxicology (Amsterdam, Netherlands).
2012;
118-119
:
62-71
.
View Article PubMed Google Scholar -
Ku
P.,
Wu
X.,
Nie
X.,
Ou
R.,
Wang
L.,
Su
T.,
Effects of triclosan on the detoxification system in the yellow catfish (Pelteobagrus fulvidraco): expressions of CYP and GST genes and corresponding enzyme activity in phase I, II and antioxidant system. Comparative Biochemistry and Physiology. Toxicology & Pharmacology : CBP.
2014;
166
:
105-14
.
View Article PubMed Google Scholar -
Banerjee
P.,
Dey
T.K.,
Sarkar
S.,
Swarnakar
S.,
Mukhopadhyay
A.,
Ghosh
S.,
Treatment of cosmetic effluent in different configurations of ceramic UF membrane based bioreactor: toxicity evaluation of the untreated and treated wastewater using catfish (Heteropneustes fossilis). Chemosphere.
2016;
146
:
133-44
.
View Article PubMed Google Scholar -
Pirone
G.,
Coppola
F.,
Pretti
C.,
Soares
A.M.,
Solé
M.,
Freitas
R.,
The effect of temperature on Triclosan and Lead exposed mussels. Comparative Biochemistry and Physiology. Part B, Biochemistry & Molecular Biology.
2019;
232
:
42-50
.
View Article PubMed Google Scholar -
Berger
K.,
Gunier
R.B.,
Chevrier
J.,
Calafat
A.M.,
Ye
X.,
Eskenazi
B.,
Associations of maternal exposure to triclosan, parabens, and other phenols with prenatal maternal and neonatal thyroid hormone levels. Environmental Research.
2018;
165
:
379-86
.
View Article PubMed Google Scholar -
Ak
T.,
Gülçin
I.,
Antioxidant and radical scavenging properties of curcumin. Chemico-Biological Interactions.
2008;
174
(1)
:
27-37
.
View Article PubMed Google Scholar -
Garcia, Ashley Marie, "Effect of Triclosan-Tolerant Plant Growth-Promoting Rhizobacteria on Triclosan Degradation in Soils" (2020). Theses and Dissertations. 113. https://rio.tamiu.edu/etds/113.
.
-
Aderogba
M.,
Okoh
E.,
Idowu
T.,
Aderogba
M.A.,
Okoh
E.K.,
Idowu
T.O.,
Evaluation of the antioxidant activity of the secondary metabolites from Piliostigma reticulatum (DC.) Hochst. Journal of Biological Sciences (Faisalabad, Pakistan).
2005;
5
(2)
:
239-42
.
View Article Google Scholar -
Kähkönen
M.P.,
Hopia
A.I.,
Vuorela
H.J.,
Rauha
J.P.,
Pihlaja
K.,
Kujala
T.S.,
Antioxidant activity of plant extracts containing phenolic compounds. Journal of Agricultural and Food Chemistry.
1999;
47
(10)
:
3954-62
.
View Article PubMed Google Scholar -
Horie
Y.,
Yamagishi
T.,
Takahashi
H.,
Iguchi
T.,
Tatarazako
N.,
Effects of triclosan on Japanese medaka (Oryzias latipes) during embryo development, early life stage and reproduction. Journal of Applied Toxicology.
2018;
38
(4)
:
544-51
.
View Article PubMed Google Scholar -
Cho
E.J.,
Yokozawa
T.,
Rhyu
D.Y.,
Kim
S.C.,
Shibahara
N.,
Park
J.C.,
Study on the inhibitory effects of Korean medicinal plants and their main compounds on the 1,1-diphenyl-2-picrylhydrazyl radical. Phytomedicine.
2003;
10
(6-7)
:
544-51
.
View Article PubMed Google Scholar -
Regös
J.,
Zak
O.,
Solf
R.,
Vischer
W.A.,
Weirich
E.G.,
Antimicrobial spectrum of triclosan, a broad-spectrum antimicrobial agent for topical application. II. Comparison with some other antimicrobial agents. Dermatologica.
1979;
158
(1)
:
72-9
.
View Article PubMed Google Scholar -
Regös
J.,
Hitz
H.R.,
Investigations on the mode of action of Triclosan, a broad spectrum antimicrobial agent. Zentralblatt f{&}{#}x00FC;r Bakteriologie, Parasitenkunde, Infektionskrankheiten und Hygiene. Erste Abteilung Originale. Reihe A: Medizinische Mikrobiologie und Parasitologie.
1974;
226
(3)
:
390-401
.
PubMed Google Scholar -
Betts
J.C.,
McLaren
A.,
Lennon
M.G.,
Kelly
F.M.,
Lukey
P.T.,
Blakemore
S.J.,
Signature gene expression profiles discriminate between isoniazid-, thiolactomycin-, and triclosan-treated Mycobacterium tuberculosis. Antimicrobial Agents and Chemotherapy.
2003;
47
(9)
:
2903-13
.
View Article PubMed Google Scholar -
Srinivasan
V.B.,
Singh
B.B.,
Priyadarshi
N.,
Chauhan
N.K.,
Rajamohan
G.,
Role of novel multidrug efflux pump involved in drug resistance in Klebsiella pneumoniae. PLoS One.
2014;
9
(5)
:
e96288
.
View Article PubMed Google Scholar -
McMurry
L.M.,
Oethinger
M.,
Levy
S.B.,
Overexpression of marA, soxS, or acrAB produces resistance to triclosan in laboratory and clinical strains of Escherichia coli. FEMS Microbiology Letters.
1998;
166
(2)
:
305-9
.
View Article PubMed Google Scholar -
Miller
T.L.,
Deinzer
M.L.,
Effects of nonachloropredioxin and other hydroxychlorodiphenyl ethers on biological membranes. Journal of Toxicology and Environmental Health.
1980;
6
(1)
:
11-25
.
View Article PubMed Google Scholar -
Villalaín
J.,
Mateo
C.R.,
Aranda
F.J.,
Shapiro
S.,
Micol
V.,
Membranotropic effects of the antibacterial agent Triclosan. Archives of Biochemistry and Biophysics.
2001;
390
(1)
:
128-36
.
View Article PubMed Google Scholar -
Guillén
J.,
Bernabeu
A.,
Shapiro
S.,
Villalaín
J.,
Location and orientation of Triclosan in phospholipid model membranes. European Biophysics Journal.
2004;
33
(5)
:
448-53
.
View Article PubMed Google Scholar -
Honkisz
E.,
Zieba-Przybylska
D.,
Wojtowicz
A.K.,
The effect of triclosan on hormone secretion and viability of human choriocarcinoma JEG-3 cells. Reproductive Toxicology (Elmsford, N.Y.).
2012;
34
(3)
:
385-92
.
View Article PubMed Google Scholar -
Winitthana
T.,
Lawanprasert
S.,
Chanvorachote
P.,
Triclosan potentiates epithelial-to-mesenchymal transition in anoikis-resistant human lung cancer cells. PLoS One.
2014;
9
(10)
:
e110851
.
View Article PubMed Google Scholar -
Kim
Y.S.,
Seo
H.W.,
Lee
M.H.,
Kim
D.K.,
Jeon
H.,
Cha
D.S.,
Protocatechuic acid extends lifespan and increases stress resistance in Caenorhabditis elegans. Archives of Pharmacal Research.
2014;
37
(2)
:
245-52
.
View Article PubMed Google Scholar -
Shin
M.K.,
Jeon
Y.D.,
Hong
S.H.,
Kang
S.H.,
Kee
J.Y.,
Jin
J.S.,
In Vivo and In Vitro Effects of Tracheloside on Colorectal Cancer Cell Proliferation and Metastasis. Antioxidants.
2021;
10
(4)
:
513
.
View Article PubMed Google Scholar -
Farasani
A.,
Darbre
P.D.,
Long-term exposure to triclosan increases migration and invasion of human breast epithelial cells in vitro. Journal of Applied Toxicology.
2021;
41
(7)
:
1115-26
.
View Article PubMed Google Scholar -
Lee
H.R.,
Hwang
K.A.,
Nam
K.H.,
Kim
H.C.,
Choi
K.C.,
Progression of breast cancer cells was enhanced by endocrine-disrupting chemicals, triclosan and octylphenol, via an estrogen receptor-dependent signaling pathway in cellular and mouse xenograft models. Chemical Research in Toxicology.
2014;
27
(5)
:
834-42
.
View Article PubMed Google Scholar -
Aliaga
A.,
Castells
A.,
Kriznik
D.,
Lalosević
J.,
Marrón
J.,
Moragas
J.,
An overview of two comparative multicentre trials with halometasone/triclosan cream in acute superficial bacterial skin infections. The Journal of International Medical Research.
1983;
11
:
53-7
.
PubMed Google Scholar -
Huber
L.,
Role of Klion ointment in the treatment of crural ulcer. Therapia Hungarica (English Edition).
1991;
39
(3)
:
148-150
.
PubMed Google Scholar -
Culić
O.,
Eraković
V.,
Parnham
M.J.,
Anti-inflammatory effects of macrolide antibiotics. European Journal of Pharmacology.
2001;
429
(1-3)
:
209-29
.
View Article PubMed Google Scholar -
Gaffar
A.,
Scherl
D.,
Afflitto
J.,
Coleman
E.J.,
The effect of triclosan on mediators of gingival inflammation. Journal of Clinical Periodontology.
1995;
22
(6)
:
480-4
.
View Article PubMed Google Scholar -
Modéer
T.,
Bengtsson
A.,
Rölla
G.,
Triclosan reduces prostaglandin biosynthesis in human gingival fibroblasts challenged with interleukin-1 in vitro. Journal of Clinical Periodontology.
1996;
23
(10)
:
927-33
.
View Article PubMed Google Scholar -
Skaare
A.B.,
Rölla
G.,
Barkvoll
P.,
The influence of triclosan, zinc or propylene glycol on oral mucosa exposed to sodium lauryl sulphate. European Journal of Oral Sciences.
1997;
105
(5 Pt 2)
:
527-33
.
View Article PubMed Google Scholar -
Mustafa
M.,
Wondimu
B.,
Hultenby
K.,
Yucel-Lindberg
T.,
Modéer
T.,
Uptake, distribution and release of 14C-triclosan in human gingival fibroblasts. Journal of Pharmaceutical Sciences.
2003;
92
(8)
:
1648-53
.
View Article PubMed Google Scholar -
Wallet
M.A.,
Calderon
N.,
Alonso
T.R.,
Choe
C.S.,
Catalfamo
D.,
Lalane
C.J.,
Triclosan alters antimicrobial and inflammatory responses of epithelial cells. Oral Diseases.
2013;
19
(3)
:
296-302
.
View Article PubMed Google Scholar -
Neiva
K.G.,
Calderon
N.L.,
Alonso
T.R.,
Panagakos
F.,
Wallet
S.M.,
Type 1 diabetes-associated TLR responsiveness of oral epithelial cells. Journal of Dental Research.
2014;
93
(2)
:
169-74
.
View Article PubMed Google Scholar -
Marshall
N.B.,
Lukomska
E.,
Nayak
A.P.,
Long
C.M.,
Hettick
J.M.,
Anderson
S.E.,
Topical application of the anti-microbial chemical triclosan induces immunomodulatory responses through the S100A8/A9-TLR4 pathway. Journal of Immunotoxicology.
2017;
14
(1)
:
50-9
.
View Article PubMed Google Scholar -
Barnes
V.M.,
Xu
T.,
Shimizu
E.,
Nakatani
T.,
Jefcoat
S.,
Vasilov
A.,
Triclosan blocks MMP-13 expression in hormone-stimulated osteoblasts. Journal of Periodontology.
2013;
84
(11)
:
1683-9
.
PubMed Google Scholar -
Pancer
B.A.,
Kott
D.,
Sugai
J.V.,
Panagakos
F.S.,
Braun
T.M.,
Teles
R.P.,
Effects of triclosan on host response and microbial biomarkers during experimental gingivitis. Journal of Clinical Periodontology.
2016;
43
(5)
:
435-44
.
View Article PubMed Google Scholar -
Watkins
D.J.,
Ferguson
K.K.,
Anzalota Del Toro
L.V.,
Alshawabkeh
A.N.,
Cordero
J.F.,
Meeker
J.D.,
Associations between urinary phenol and paraben concentrations and markers of oxidative stress and inflammation among pregnant women in Puerto Rico. International Journal of Hygiene and Environmental Health.
2015;
218
(2)
:
212-9
.
View Article PubMed Google Scholar -
Uings
I.J.,
Farrow
S.N.,
Cell receptors and cell signalling. Molecular Pathology.
2000;
53
(6)
:
295-9
.
View Article PubMed Google Scholar -
Wu
Y.,
Beland
F.A.,
Chen
S.,
Fang
J.L.,
Extracellular signal-regulated kinases 1/2 and Akt contribute to triclosan-stimulated proliferation of JB6 Cl 41-5a cells. Archives of Toxicology.
2015;
89
(8)
:
1297-311
.
View Article PubMed Google Scholar -
Dubey
D.,
Srivastav
A.K.,
Singh
J.,
Chopra
D.,
Qureshi
S.,
Kushwaha
H.N.,
Photoexcited triclosan induced DNA damage and oxidative stress via p38 MAP kinase signaling involving type I radicals under sunlight/UVB exposure. Ecotoxicology and Environmental Safety.
2019;
174
:
270-82
.
View Article PubMed Google Scholar -
Park
B.K.,
Gonzales
E.L.,
Yang
S.M.,
Bang
M.,
Choi
C.S.,
Shin
C.Y.,
Effects of triclosan on neural stem cell viability and survival. Biomolecules {&}amp; Therapeutics.
2016;
24
(1)
:
99-107
.
View Article PubMed Google Scholar -
Zhang
P.,
Yang
M.,
Zeng
L.,
Liu
C.,
P38/TRHr-dependent regulation of TPO in thyroid cells contributes to the hypothyroidism of triclosan-treated rats. Cellular Physiology and Biochemistry.
2018;
45
(4)
:
1303-15
.
View Article PubMed Google Scholar -
Barros
S.P.,
Wirojchanasak
S.,
Barrow
D.A.,
Panagakos
F.S.,
Devizio
W.,
Offenbacher
S.,
Triclosan inhibition of acute and chronic inflammatory gene pathways. Journal of Clinical Periodontology.
2010;
37
(5)
:
412-8
.
View Article PubMed Google Scholar -
Huang
H.,
Du
G.,
Zhang
W.,
Hu
J.,
Wu
D.,
Song
L.,
The in vitro estrogenic activities of triclosan and triclocarban. Journal of Applied Toxicology.
2014;
34
(9)
:
1060-7
.
View Article PubMed Google Scholar -
Stoker
T.E.,
Gibson
E.K.,
Zorrilla
L.M.,
Triclosan exposure modulates estrogen-dependent responses in the female wistar rat. Toxicological Sciences.
2010;
117
(1)
:
45-53
.
View Article PubMed Google Scholar -
James
M.O.,
Li
W.,
Summerlot
D.P.,
Rowland-Faux
L.,
Wood
C.E.,
Triclosan is a potent inhibitor of estradiol and estrone sulfonation in sheep placenta. Environment International.
2010;
36
(8)
:
942-9
.
View Article PubMed Google Scholar -
Liu
J.,
Sun
L.,
Zhang
H.,
Shi
M.,
Dahlgren
R.A.,
Wang
X.,
Response mechanisms to joint exposure of triclosan and its chlorinated derivatives on zebrafish (Danio rerio) behavior. Chemosphere.
2018;
193
:
820-32
.
View Article PubMed Google Scholar -
Chen
J.,
Ahn
K.C.,
Gee
N.A.,
Gee
S.J.,
Hammock
B.D.,
Lasley
B.L.,
Antiandrogenic properties of parabens and other phenolic containing small molecules in personal care products. Toxicology and Applied Pharmacology.
2007;
221
(3)
:
278-84
.
View Article PubMed Google Scholar -
Riad
M.A.,
Abd-Rabo
M.M.,
Abd El Aziz
S.A.,
El Behairy
A.M.,
Badawy
M.M.,
Reproductive toxic impact of subchronic treatment with combined butylparaben and triclosan in weanling male rats. Journal of Biochemical and Molecular Toxicology.
2018;
32
(3)
:
e22037
.
View Article PubMed Google Scholar -
Clapham
D.E.,
Calcium signaling. Cell.
2007;
131
(6)
:
1047-58
.
View Article PubMed Google Scholar -
Cherednichenko
G.,
Zhang
R.,
Bannister
R.A.,
Timofeyev
V.,
Li
N.,
Fritsch
E.B.,
Triclosan impairs excitation-contraction coupling and Ca2+ dynamics in striated muscle. Proceedings of the National Academy of Sciences of the United States of America.
2012;
109
(35)
:
14158-63
.
View Article PubMed Google Scholar -
Fritsch
E.B.,
Connon
R.E.,
Werner
I.,
Davies
R.E.,
Beggel
S.,
Feng
W.,
Triclosan impairs swimming behavior and alters expression of excitation-contraction coupling proteins in fathead minnow (Pimephales promelas). Environmental Science & Technology.
2013;
47
(4)
:
2008-17
.
View Article PubMed Google Scholar -
Li
S.J.,
Chen
P.,
Peres
T.V.,
Villahoz
B.F.,
Zhang
Z.,
Miah
M.R.,
Triclosan induces PC12 cells injury is accompanied by inhibition of AKT/mTOR and activation of p38 pathway. Neurotoxicology.
2019;
74
:
221-9
.
View Article PubMed Google Scholar -
Hussien
N.A.,
Hamdi
H.,
Genotoxic and hypogonadism effect of triclosan treatment and the mitigating effect of vitamin e in male albino mice. International Journal of Clinical Pharmacy.
2017;
8
(4)
.
-
Olaniyan
L.W.,
Mkwetshana
N.,
Okoh
A.I.,
Triclosan in water, implications for human and environmental health. SpringerPlus.
2016;
5
(1)
:
1639
.
View Article PubMed Google Scholar -
Wang
C.,
Li
Y.,
Zheng
G.,
Zhang
S.,
Wan
Y.,
Hu
J.,
Adverse effects of triclosan and binary mixtures with 17β-estradiol on testicular development and reproduction in Japanese medaka (Oryzias latipes) at environmentally relevant concentrations. Environmental Science & Technology Letters.
2018;
5
(3)
:
136-41
.
View Article Google Scholar -
Muller
D.,
Nelles
J.,
Deparade
E.,
Arni
P.,
Studies of the activity of S9-liver fractions from various species in the Ames test. Mutation Research - Environmental Mutagenesis and Related Subjets.
1979;
64
(2)
:
104-5
.
View Article Google Scholar -
Wang
Z.,
Li
X.,
Klaunig
J.E.,
Investigation of the mechanism of triclosan induced mouse liver tumors. Regulatory Toxicology and Pharmacology.
2017;
86
:
137-47
.
View Article PubMed Google Scholar -
Strasser
F.,
Müller
D.,
Chromosome Studies on Somatic Cells-GP 41 353 (Triclosan)Chinese Hamster. Ciba-Geigy Ltd. 1973.
Google Scholar -
Müller
D.,
Strasser
F.F.,
Röhrborn
G.,
Fränz
J.,
Grafe
A.,
Mittenburger
H.G.,
Chromosome analysis of bone marrow in mammals after treatment with isoniazid. Human Genetics.
1978;
42
(1)
:
15-25
.
View Article PubMed Google Scholar -
DeSalva
S.J.,
Kong
B.M.,
Lin
Y.J.,
Triclosan: a safety profile. American Journal of Dentistry.
1989;
2
(Spec No)
:
185-96
.
PubMed Google Scholar -
Bhargava
H.N.,
Leonard
P.A.,
Triclosan: applications and safety. American Journal of Infection Control.
1996;
24
(3)
:
209-18
.
View Article PubMed Google Scholar -
Fahrig
R.,
Nilsson
C.A.,
Rappe
C.,
Genetic activity of chlorophenols and chlorophenol impurities. PentachlorophenolSpringer 1978.
Google Scholar -
Russell
L.B.,
Montgomery
C.S.,
Use of the mouse spot test to investigate the mutagenic potential of triclosan (Irgasan DP300). Mutation Research.
1980;
79
(1)
:
7-12
.
View Article PubMed Google Scholar -
Heath
R.J.,
Rubin
J.R.,
Holland
D.R.,
Zhang
E.,
Snow
M.E.,
Rock
C.O.,
Mechanism of triclosan inhibition of bacterial fatty acid synthesis. The Journal of Biological Chemistry.
1999;
274
(16)
:
11110-4
.
View Article PubMed Google Scholar -
Sivaraman
S.,
Zwahlen
J.,
Bell
A.F.,
Hedstrom
L.,
Tonge
P.J.,
Structure-activity studies of the inhibition of FabI, the enoyl reductase from Escherichia coli, by triclosan: kinetic analysis of mutant FabIs. Biochemistry.
2003;
42
(15)
:
4406-13
.
View Article PubMed Google Scholar -
Lu
J.,
Jin
M.,
Nguyen
S.H.,
Mao
L.,
Li
J.,
Coin
L.J.,
Non-antibiotic antimicrobial triclosan induces multiple antibiotic resistance through genetic mutation. Environment International.
2018;
118
:
257-65
.
View Article PubMed Google Scholar -
Yasir
M.,
Turner
A.K.,
Bastkowski
S.,
Page
A.J.,
Telatin
A.,
Phan
M.D.,
A new massively-parallel transposon mutagenesis approach comparing multiple datasets identifies novel mechanisms of action and resistance to triclosan. bioRxiv.
2019;
2019
.
View Article Google Scholar -
Grundström
T.,
Jaurin
B.,
Overlap between ampC and frd operons on the Escherichia coli chromosome. Proceedings of the National Academy of Sciences of the United States of America.
1982;
79
(4)
:
1111-5
.
View Article PubMed Google Scholar -
Tracz
D.M.,
Boyd
D.A.,
Bryden
L.,
Hizon
R.,
Giercke
S.,
Van Caeseele
P.,
Increase in ampC promoter strength due to mutations and deletion of the attenuator in a clinical isolate of cefoxitin-resistant Escherichia coli as determined by RT-PCR. The Journal of Antimicrobial Chemotherapy.
2005;
55
(5)
:
768-72
.
View Article PubMed Google Scholar -
Castellanos
L.R.,
Donado-Godoy
P.,
León
M.,
Clavijo
V.,
Arevalo
A.,
Bernal
J.F.,
High heterogeneity of Escherichia coli sequence types harbouring ESBL/AmpC genes on IncI1 plasmids in the Colombian poultry chain. PLoS One.
2017;
12
(1)
:
e0170777
.
View Article PubMed Google Scholar -
Koutsolioutsou
A.,
Peña-Llopis
S.,
Demple
B.,
Constitutive soxR mutations contribute to multiple-antibiotic resistance in clinical Escherichia coli isolates. Antimicrobial Agents and Chemotherapy.
2005;
49
(7)
:
2746-52
.
View Article PubMed Google Scholar -
White
D.G.,
Goldman
J.D.,
Demple
B.,
Levy
S.B.,
Role of the acrAB locus in organic solvent tolerance mediated by expression of marA, soxS, or robA in Escherichia coli. Journal of Bacteriology.
1997;
179
(19)
:
6122-6
.
View Article PubMed Google Scholar -
Liu
Y.,
Li
R.,
Xiao
X.,
Wang
Z.,
Antibiotic adjuvants: an alternative approach to overcome multi-drug resistant Gram-negative bacteria. Critical Reviews in Microbiology.
2019;
45
(3)
:
301-14
.
View Article PubMed Google Scholar -
Bhandol
H.J.,
Alindogan
J.,
de Guzman
A.,
Lim
R.,
Structure and regulation of the Acr efflux pumps and their role in antibiotic resistance in Escherichia coli. Undergraduate J Exp Microbiol Immunol..
2020;
6
:
1-16
.
-
Duarte
N.A.,
de Lima
L.E.,
Maraslis
F.T.,
Kundi
M.,
Nunes
E.A.,
Barcelos
G.R.,
Acute toxicity and DNA instability induced by exposure to low doses of triclosan and phthalate DEHP, and their combinations, in vitro. Frontiers in Genetics.
2021;
12
:
649845
.
View Article PubMed Google Scholar -
Sun
L.,
Gao
M.,
Qian
Q.,
Guo
Z.,
Zhu
P.,
Wang
X.,
Triclosan-induced abnormal expression of miR-30b regulates fto-mediated m6A methylation level to cause lipid metabolism disorder in zebrafish. The Science of the Total Environment.
2021;
770
:
145285
.
View Article PubMed Google Scholar -
Schena
D.,
Papagrigoraki
A.,
Girolomoni
G.,
Sensitizing potential of triclosan and triclosan-based skin care products in patients with chronic eczema. Dermatologic Therapy.
2008;
21
:
35-8
.
View Article PubMed Google Scholar -
Robertshaw
H.,
Leppard
B.,
Contact dermatitis to triclosan in toothpaste. Contact Dermatitis.
2007;
57
(6)
:
383-4
.
View Article PubMed Google Scholar -
Spanier AJ, Fausnight T, Camacho TF, Braun JM, editors. The associations of triclosan and paraben exposure with allergen sensitization and wheeze in children. Allergy Asthma Proc; 2014: OceanSide Publications.
.
-
Chen
Y.,
Fang
J.,
Ren
L.,
Fan
R.,
Zhang
J.,
Liu
G.,
Urinary bisphenol analogues and triclosan in children from south China and implications for human exposure. Environmental Pollution.
2018;
238
:
299-305
.
View Article PubMed Google Scholar -
Roy
J.R.,
Chakraborty
S.,
Chakraborty
T.R.,
Estrogen-like endocrine disrupting chemicals affecting puberty in humans - review. Medical Science Monitor.
2009;
15
(6)
:
137-45
.
PubMed Google Scholar -
Darbre
P.D.,
Environmental oestrogens, cosmetics and breast cancer. Best Practice & Research. Clinical Endocrinology & Metabolism.
2006;
20
(1)
:
121-43
.
View Article PubMed Google Scholar -
Wilson
A.S.,
Power
B.E.,
Molloy
P.L.,
DNA hypomethylation and human diseases. Biochimica et Biophysica Acta.
2007;
1775
(1)
:
138-62
.
PubMed Google Scholar -
Gee
R.H.,
Charles
A.,
Taylor
N.,
Darbre
P.D.,
Oestrogenic and androgenic activity of triclosan in breast cancer cells. Journal of Applied Toxicology.
2008;
28
(1)
:
78-91
.
View Article PubMed Google Scholar -
Bertelsen
R.J.,
Longnecker
M.P.,
L∅vik
M.,
Calafat
A.M.,
Carlsen
K.H.,
London
S.J.,
Triclosan exposure and allergic sensitization in Norwegian children. Allergy.
2013;
68
(1)
:
84-91
.
View Article PubMed Google Scholar -
Lin
Z.,
Zhang
X.,
Zhao
F.,
Ru
S.,
Bisphenol S promotes the cell cycle progression and cell proliferation through ERα-cyclin D-CDK4/6-pRb pathway in MCF-7 breast cancer cells. Toxicology and Applied Pharmacology.
2019;
366
:
75-82
.
View Article PubMed Google Scholar -
Dayan
A.D.,
Risk assessment of triclosan [Irgasan] in human breast milk. Food and Chemical Toxicology.
2007;
45
(1)
:
125-9
.
View Article PubMed Google Scholar -
Veldhoen
N.,
Skirrow
R.C.,
Osachoff
H.,
Wigmore
H.,
Clapson
D.J.,
Gunderson
M.P.,
The bactericidal agent triclosan modulates thyroid hormone-associated gene expression and disrupts postembryonic anuran development. Aquatic Toxicology (Amsterdam, Netherlands).
2006;
80
(3)
:
217-27
.
View Article PubMed Google Scholar -
Koeppe
E.S.,
Ferguson
K.K.,
Colacino
J.A.,
Meeker
J.D.,
Relationship between urinary triclosan and paraben concentrations and serum thyroid measures in NHANES 2007-2008. The Science of the Total Environment.
2013;
445-446
:
299-305
.
View Article PubMed Google Scholar -
Heublein
S.,
Mayr
D.,
Meindl
A.,
Angele
M.,
Gallwas
J.,
Jeschke
U.,
Thyroid hormone receptors predict prognosis in BRCA1 associated breast cancer in opposing ways. PLoS One.
2015;
10
(6)
:
e0127072
.
View Article PubMed Google Scholar -
Badv
M.,
Bayat
F.,
Weitz
J.I.,
Didar
T.F.,
Single and multi-functional coating strategies for enhancing the biocompatibility and tissue integration of blood-contacting medical implants. Biomaterials.
2020;
258
:
120291
.
View Article PubMed Google Scholar -
Derouiche
S.,
Mariot
P.,
Warnier
M.,
Vancauwenberghe
E.,
Bidaux
G.,
Gosset
P.,
Activation of TRPA1 channel by antibacterial agent triclosan induces VEGF secretion in human prostate cancer stromal cells. Cancer Prevention Research (Philadelphia, Pa.).
2017;
10
(3)
:
177-87
.
View Article PubMed Google Scholar -
Cunha
G.R.,
Hayward
S.W.,
Wang
Y.Z.,
Role of stroma in carcinogenesis of the prostate. Differentiation.
2002;
70
(9-10)
:
473-85
.
View Article PubMed Google Scholar -
Guo
Z.,
Yang
X.,
Sun
F.,
Jiang
R.,
Linn
D.E.,
Chen
H.,
A novel androgen receptor splice variant is up-regulated during prostate cancer progression and promotes androgen depletion-resistant growth. Cancer Research.
2009;
69
(6)
:
2305-13
.
View Article PubMed Google Scholar -
Jankevicius
F.,
Miller
S.M.,
Ackermann
R.,
Nutrition and risk of prostate cancer. Urologia Internationalis.
2002;
68
(2)
:
69-80
.
View Article PubMed Google Scholar -
Liotta
L.A.,
Kohn
E.C.,
The microenvironment of the tumour-host interface. Nature.
2001;
411
(6835)
:
375-9
.
View Article PubMed Google Scholar -
Gao
L.,
Yuan
T.,
Cheng
P.,
Bai
Q.,
Zhou
C.,
Ao
J.,
Effects of triclosan and triclocarban on the growth inhibition, cell viability, genotoxicity and multixenobiotic resistance responses of Tetrahymena thermophila. Chemosphere.
2015;
139
:
434-40
.
View Article PubMed Google Scholar -
Sethuraman
S.,
Kumar
T.R.,
Triclosan induced genotoxic effect on zebra fish, brachydanio rerio by using alkaline comet assay. Int J Modn Res Revs..
2014;
2
(11)
:
473-7
.
-
Wang
F.,
Zheng
F.,
Liu
F.,
Effects of triclosan on antioxidant-and apoptosis-related genes expression in the gill and ovary of zebrafish. Experimental Animals.
2019;
69
(2)
:
199-206
.
View Article Google Scholar -
Binelli
A.,
Cogni
D.,
Parolini
M.,
Riva
C.,
Provini
A.,
Cytotoxic and genotoxic effects of in vitro exposure to triclosan and trimethoprim on zebra mussel (Dreissena polymorpha) hemocytes. Comparative Biochemistry and Physiology. Toxicology {&}amp; Pharmacology : CBP.
2009;
150
(1)
:
50-6
.
View Article PubMed Google Scholar -
Ciniglia
C.,
Cascone
C.,
Giudice
R.L.,
Pinto
G.,
Pollio
A.,
Application of methods for assessing the geno- and cytotoxicity of Triclosan to C. ehrenbergii. Journal of Hazardous Materials.
2005;
122
(3)
:
227-32
.
View Article PubMed Google Scholar -
Yueh
M.F.,
Taniguchi
K.,
Chen
S.,
Evans
R.M.,
Hammock
B.D.,
Karin
M.,
The commonly used antimicrobial additive triclosan is a liver tumor promoter. Proceedings of the National Academy of Sciences of the United States of America.
2014;
111
(48)
:
17200-5
.
View Article PubMed Google Scholar -
Cavas
T.,
Garanko
N.N.,
Arkhipchuk
V.V.,
Induction of micronuclei and binuclei in blood, gill and liver cells of fishes subchronically exposed to cadmium chloride and copper sulphate. Food and Chemical Toxicology.
2005;
43
(4)
:
569-74
.
View Article PubMed Google Scholar -
Cavaş
T.,
Ergene-Gözükara
S.,
Induction of micronuclei and nuclear abnormalities in Oreochromis niloticus following exposure to petroleum refinery and chromium processing plant effluents. Aquatic Toxicology (Amsterdam, Netherlands).
2005;
74
(3)
:
264-71
.
View Article PubMed Google Scholar -
Xu
X.,
Lu
Y.,
Zhang
D.,
Wang
Y.,
Zhou
X.,
Xu
H.,
Toxic assessment of triclosan and triclocarban on Artemia salina. Bulletin of Environmental Contamination and Toxicology.
2015;
95
(6)
:
728-33
.
View Article PubMed Google Scholar -
Kim
S.H.,
Choi
K.C.,
Anti-cancer effect and underlying mechanism (s) of kaempferol, a phytoestrogen, on the regulation of apoptosis in diverse cancer cell models. Toxicological Research.
2013;
29
(4)
:
229-34
.
View Article PubMed Google Scholar -
Danial
N.N.,
Korsmeyer
S.J.,
Cell death: critical control points. Cell.
2004;
116
(2)
:
205-19
.
View Article PubMed Google Scholar -
Klein
G.,
Klein
E.,
Immune surveillance against virus-induced tumors and nonrejectability of spontaneous tumors: contrasting consequences of host versus tumor evolution. Proceedings of the National Academy of Sciences of the United States of America.
1977;
74
(5)
:
2121-5
.
View Article PubMed Google Scholar -
McDonnell
G.,
Russell
A.D.,
Antiseptics and disinfectants: activity, action, and resistance. Clinical Microbiology Reviews.
2001;
14
(1)
:
227
.
View Article PubMed Google Scholar -
Pizer
E.S.,
Jackisch
C.,
Wood
F.D.,
Pasternack
G.R.,
Davidson
N.E.,
Kuhajda
F.P.,
Inhibition of fatty acid synthesis induces programmed cell death in human breast cancer cells. Cancer Research.
1996;
56
(12)
:
2745-7
.
PubMed Google Scholar -
Wilentz
R.E.,
Witters
L.A.,
Pizer
E.S.,
Lipogenic enzymes fatty acid synthase and acetyl-coenzyme A carboxylase are coexpressed with sterol regulatory element binding protein and Ki-67 in fetal tissues. Pediatric and Developmental Pathology.
2000;
3
(6)
:
525-31
.
View Article PubMed Google Scholar -
Branca
F.,
Lorenzetti
S.,
Health effects of phytoestrogens. Forum of Nutrition.
2005;
57
(57)
:
100-11
.
View Article PubMed Google Scholar -
Ren
W.,
Qiao
Z.,
Wang
H.,
Zhu
L.,
Zhang
L.,
Flavonoids: promising anticancer agents. Medicinal Research Reviews.
2003;
23
(4)
:
519-34
.
View Article PubMed Google Scholar -
Adlercreutz
H.,
Mousavi
Y.,
Clark
J.,
Höckerstedt
K.,
Hämäläinen
E.,
Wähälä
K.,
Dietary phytoestrogens and cancer: in vitro and in vivo studies. The Journal of Steroid Biochemistry and Molecular Biology.
1992;
41
(3-8)
:
331-7
.
View Article PubMed Google Scholar -
Chen
A.Y.,
Chen
Y.C.,
A review of the dietary flavonoid, kaempferol on human health and cancer chemoprevention. Food Chemistry.
2013;
138
(4)
:
2099-107
.
View Article PubMed Google Scholar -
Chen
H.J.,
Lin
C.M.,
Lee
C.Y.,
Shih
N.C.,
Peng
S.F.,
Tsuzuki
M.,
Kaempferol suppresses cell metastasis via inhibition of the ERK-p38-JNK and AP-1 signaling pathways in U-2 OS human osteosarcoma cells. Oncology Reports.
2013;
30
(2)
:
925-32
.
View Article PubMed Google Scholar -
Lee
K.M.,
Lee
D.E.,
Seo
S.K.,
Hwang
M.K.,
Heo
Y.S.,
Lee
K.W.,
Phosphatidylinositol 3-kinase, a novel target molecule for the inhibitory effects of kaempferol on neoplastic cell transformation. Carcinogenesis.
2010;
31
(8)
:
1338-43
.
View Article PubMed Google Scholar -
Lenz
K.A.,
Pattison
C.,
Ma
H.,
Triclosan (TCS) and triclocarban (TCC) induce systemic toxic effects in a model organism the nematode Caenorhabditis elegans. Environmental Pollution.
2017;
231
(Pt 1)
:
462-70
.
View Article PubMed Google Scholar -
Geens
T.,
Neels
H.,
Covaci
A.,
Distribution of bisphenol-A, triclosan and n-nonylphenol in human adipose tissue, liver and brain. Chemosphere.
2012;
87
(7)
:
796-802
.
View Article PubMed Google Scholar -
Wang
L.Q.,
Falany
C.N.,
James
M.O.,
Triclosan as a substrate and inhibitor of 3'-phosphoadenosine 5'-phosphosulfate-sulfotransferase and UDP-glucuronosyl transferase in human liver fractions. Drug Metabolism and Disposition: the Biological Fate of Chemicals.
2004;
32
(10)
:
1162-9
.
View Article PubMed Google Scholar -
Palmer
R.K.,
Hutchinson
L.M.,
Burpee
B.T.,
Tupper
E.J.,
Pelletier
J.H.,
Kormendy
Z.,
Antibacterial agent triclosan suppresses RBL-2H3 mast cell function. Toxicology and Applied Pharmacology.
2012;
258
(1)
:
99-108
.
View Article PubMed Google Scholar -
Higgins
C.P.,
Paesani
Z.J.,
Chalew
T.E.,
Halden
R.U.,
Hundal
L.S.,
Persistence of triclocarban and triclosan in soils after land application of biosolids and bioaccumulation in Eisenia foetida. Environmental Toxicology and Chemistry.
2011;
30
(3)
:
556-63
.
View Article PubMed Google Scholar -
Xie
X.,
Lu
C.,
Wu
M.,
Liang
J.,
Ying
Y.,
Liu
K.,
Association between triclocarban and triclosan exposures and the risks of type 2 diabetes mellitus and impaired glucose tolerance in the National Health and Nutrition Examination Survey (NHANES 2013-2014). Environment International.
2020;
136
:
105445
.
View Article PubMed Google Scholar -
Huang
C.L.,
Abass
O.K.,
Yu
C.P.,
Triclosan: A review on systematic risk assessment and control from the perspective of substance flow analysis. The Science of the Total Environment.
2016;
566-567
:
771-85
.
View Article PubMed Google Scholar
Comments
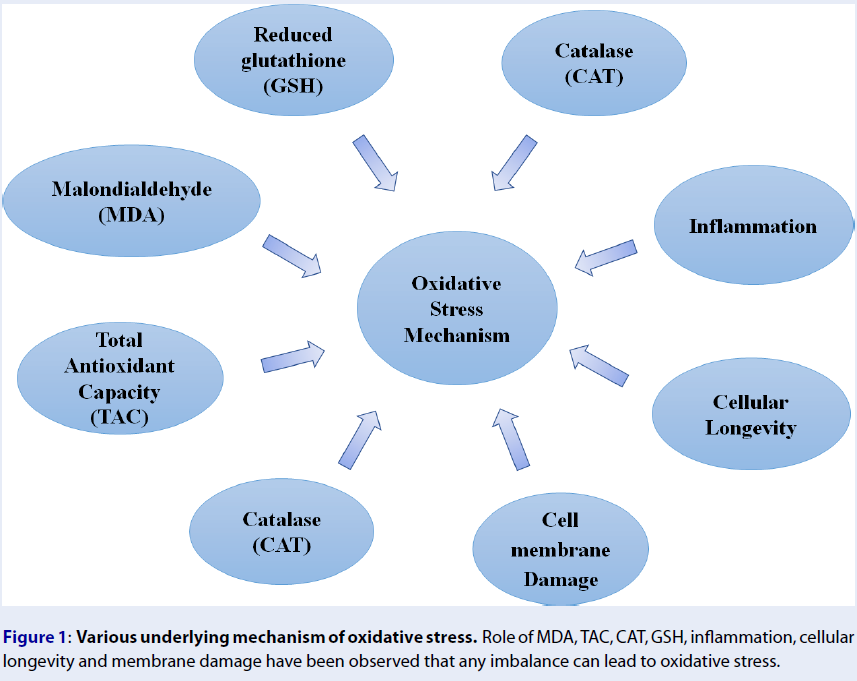
Article Details
Volume & Issue : Vol 8 No 12 (2021)
Page No.: 4750-4774
Published on: 2021-12-30
Citations
Copyrights & License

This work is licensed under a Creative Commons Attribution 4.0 International License.
Search Panel
Pubmed
Google Scholar
Pubmed
Google Scholar
Pubmed
Google Scholar
Pubmed
Search for this article in:
Google Scholar
Researchgate
- HTML viewed - 6718 times
- PDF downloaded - 1614 times
- XML downloaded - 0 times