Abstract
Background: Monocytic microparticles (mMPs) are microparticles derived from activated human monocytes and play an important role in cell-to-cell communication in the circulation. Endothelial cells also exist in the circulation and are thought to have a complex interaction with the released mMPs. Both mMPs and endothelial cells are important players in inflammation. However, the underlying mechanism exerted by mMPs in modulating monocyte and endothelial cell activation during inflammation remains unclear.
Methods: Monocytic THP-1 and U937 cells, as well as human blood monocytes, were cultured in the presence or absence of their corresponding mMPs prior to assessing tumor necrosis factor-a (TNF-a), interleukin 1b (IL-1b ), and interleukin 6 (IL-6) secretion. Upon the culture of human umbilical vein endothelial cells (HUVECs) in the presence or absence of mMPs, the expression of CD31, intracellular adhesion molecule 1 (ICAM-1), and vascular cell adhesion molecule 1 (VCAM-1) by HUVECs and endothelial microparticles (eMPs) was assessed.
Results: Our results showed that THP-1 cells and stimulated monocyte-derived mMPs enhanced IL-1b and IL-6 secretion, respectively. Additionally, mMPs derived from stimulated monocytes enhanced the expression of CD31, ICAM-1, and VCAM-1 by HUVECs and increased the release of eMPs.
Conclusion: These data suggest that mMPs may exacerbate inflammation through IL-1b and IL-6 activity and facilitate monocyte recruitment to inflammatory sites via CD31, ICAM-1, and VCAM-1.
Introduction
Monocytic microparticles (mMPs) are small vesicles derived from the monocyte cell membrane following activation or apoptosis. Monocytic MP are round in shape with sizes of approximately 140 nm to 1000 nm1. Microparticles that have phenotypic and cytosolic contents similar to those of their origin cells have emerged as pivotal markers of inflammation. High levels of mMPs have been detected in various clinical conditions such as vascular inflammation2, thrombosis, and angiogenesis3, 4. Apart from participating in cellular interactions, mMPs also contribute to inflammation by enhancing the release of numerous inflammatory cytokines and chemokines by immune cells5 as well as upregulating the expression of adhesion molecules by endothelial cells6.Cytokines are small proteins, secreted by cells, that are capable of mediating cell signaling or cell communications. Interleukin 1β (IL-1β), interleukin 6 (IL-6), and tumor necrosis factor α (TNF-α) are known as the most potent proinflammatory cytokines that are released in the presence of pathogenic invaders during inflammation. A balanced cascade between pro- and anti-inflammatory cytokines is necessary to achieve well-regulated immune activity. It has been widely reported that overproduction of IL-1 and IL-6 contributes to chronic inflammatory diseases7, while upregulation of TNF-α has been observed in the pathology of several systemic diseases8.
Monocytic MPs exert an autocrine effect on their origin monocytes. The interaction of mMPs with monocytes induces the release of the proinflammatory cytokine IL-1β9. Monocytic MPs also exert a paracrine effect on endothelial cells to facilitate monocyte–endothelial cell interaction, which is the initial step in vascular inflammation. During inflammation, mMPs support the attachment of monocytes to intracellular adhesion molecule 1 (ICAM-1) expressed on activated endothelial cells10. These processes favor the transmigration and recruitment of activated leukocytes into the vascular intima during inflammation. Meanwhile, protein ligands such as P-selectin glycoprotein ligand-1 and P-selectin, which are expressed on mMPs, permit and increase their adhesiveness to adhesion molecules on endothelial cells11. Consequently, the TNF-α, IL-1β, IL-6, and IL-8 released upon monocyte binding to endothelial cells, together with mMP-endothelial cell binding, further enhance ICAM-1, vascular cell adhesion molecule 1 (VCAM-1), and E-selectin expression by endothelial cells, which are important for leukocyte chemotaxis12.
Additionally, the interaction between mMPs and endothelial cells may induce endothelial vesiculation, resulting in the release of endothelial microparticles (eMPs). The shedding of eMPs results from the activity of proinflammatory factors such as vascular endothelial growth factors, IL-6, and TNF-α, which are released following mMP–endothelial cell interactions13. Consequently, eMPs elicit a proinflammatory response in endothelial cells by enhancing adhesion molecule expression on endothelial cells, resulting in monocyte adhesion.However, the interaction of mMPs with monocytes and with endothelial cells in amplifying vascular inflammation remains unclear. Therefore, changes in cytokine secretion by monocytes in the presence of human blood monocyte-derived mMPs were assessed. Additionally, the expression of CD31 and the adhesion molecules ICAM-1 and VCAM-1 by endothelial cells and shed eMPs were determined in the presence of mMPs. We hypothesized that mMPs induce the release of proinflammatory cytokines by monocytes and enhance ICAM-1 and VCAM-1 expression on endothelial cells.
Methods
Cell lines
The human monocytic cell lines U937 and THP-1 were purchased from American Type Culture Collection (ATCC, Manassas, VA, USA). Both cell lines were cultured in complete RPMI-1640 medium (Sigma-Aldrich, St. Louis, Missouri, USA) supplemented with 10% heat-inactivated fetal calf serum (Sigma-Aldrich, St. Louis, Missouri, USA) and 1% penicillin–streptomycin–glutamine (GIBCO, Carlsbad, CA, USA) in a 37°C, 5% CO2/air atmosphere incubator.Human umbilical vein endothelial cells (HUVECs) (PromoCell, Heidelberg, Germany) were cultured in a flask coated with 0.2% gelatin type B solution (Sigma-Aldrich, St. Louis, Missouri, USA) and were maintained in complete Endothelial Cell Growth Medium 2 (PromoCell, Heidelberg, Germany). All cell washing steps were performed using 1X phosphate-buffered saline (PBS) (Amresco, Solon, OH, USA).
Blood collection and monocyte subset isolations
Human blood samples were collected from healthy donors with appropriate informed consent as approved by the Research Ethics Committee (Human) USM (USM/JEPeM/14120522). Briefly, 30–50 ml of blood was collected from each healthy donor. Adult peripheral blood mononuclear cells (PBMCs) were isolated by density gradient centrifugation using Lymphocyte Separation Medium (LSM) (Corning, Manassas, VA, USA). PBMCs were then collected from the plasma–LSM interface layer and washed with 1X PBS before being resuspended in the appropriate buffer or media.
Isolation of human whole-blood monocytes from PBMCs was performed using a Pan Monocyte Isolation Kit (Miltenyi Biotec, Auburn, CA, USA) according to the manufacturer’s protocol.
Microparticle generation and isolation
U937s, THP-1s, and blood monocytes were stimulated with 1 µg/ml lipopolysaccharide (LPS) from Escherichia coli (E. coli) 026:B6 (Sigma-Aldrich, St. Louis, Missouri, USA) for 18 hours at 37°C in a 5% humidified CO2 incubator.
HUVECs were seeded onto a treated 6-well plate at 1x105 cells/ml in complete Endothelial Cell Growth Medium 2. Cells were grown for 24 hours. To mimic inflammatory conditions, the confluent endothelial monolayer was either stimulated with 1 µg/ml LPS or incubated with 300 µg/ml9 unstimulated or LPS-stimulated monocytes derived mMPs for 18 hours at 37°C in a 5% CO2/air atmosphere incubator. Trypsinization was performed using 0.25% trypsin-EDTA (Sigma-Aldrich, St. Louis, Missouri, USA) for 5 minutes at room temperature to detach the cells.
To isolate mMPs or eMPs, all culture supernatants were centrifuged at 500 xg for 5 minutes and 1,500 xg for 5 minutes, and finally ultracentrifuged at 20,000 xg for 60 minutes at 4°C to pellet down the MPs.
HUVECs and eMPs were stained with anti-CD31-PerCP/Cy5.5 (BioLegend, San Diego, CA, USA) and Annexin-V-FITC (BD Bioscience, San Jose, CA, USA) for 20 minutes in 1X binding buffer. Samples were analyzed by a FACS CANTO II flow cytometer (BD Bioscience, San Jose, CA, USA). HUVECs and eMPs were assessed on SSC/FSC profiles before being further gated based on the expression of CD31/Annexin-V.
Endotoxin detection
Isolated mMPs were assessed for endotoxin interference using a ToxinSensor Chromogenic Limulus Amebocyte Lysate Endotoxin Kit (GenScript, Piscataway, NJ, USA).
Monocyte culture in the presence of mMPs
THP-1 and U937 cells at a concentration of 5x105 cells were cultured with their derived mMPs at a concentration of 3x106 at a ratio of 1:6 (monocytic cell lines:mMPs). Whole monocytes at a concentration of 5x105 cells were cultured in the presence of 1 µg/mL LPS with or without 1x106 monocyte-derived mMPs in a 1:2 ratio (monocytes:mMPs)14. After 18 hours of culture, the supernatants were collected via centrifugation at 200 xg for 5 minutes and subsequently used for cytokine quantification using ELISA.
Confluent monolayers of HUVECs were serum-starved for 4 hours prior to culture with 300 µg/ml unstimulated or LPS-stimulated mMPs derived from monocytes for 2 hours in serum-free medium9 followed by RT–qPCR analysis.
Cytokine quantification
ELISA was performed to quantify the levels of cytokines released using the Human TNF-α ELISA Max Deluxe and Human IL-1β ELISA Max Deluxe kits (Biolegend, San Diego, CA, USA). The level of cytokines released in the supernatants was measured using a spectrophotometer at 450 nm and analyzed by SkanIt Software 2.4.3 RE (Thermo Scientific, Waltham, MA, USA).
Quantitative real-time polymerase chain reaction (RT–qPCR)
Total RNA was extracted using the RNeasy Mini Kit (Qiagen, Germantown, MD, USA) according to the manufacturer’s instructions, and the RNA concentration was determined using a NanoDrop ND-2000 spectrophotometer (Thermo Scientific, Waltham, MA, USA) at 450 nm. The detection of ICAM-1 and VCAM-1 was performed using RT–qPCR. Samples were prepared using the LUNA Universal One-Step RT–qPCR Kit (New England Biolabs, Ipswich, MA, USA) as recommended by the manufacturer. The primer sequences for RT–qPCR were as follows: GAPDH (forward: 5’ CCT GCA CCA ACT GCT TA 3’, reverse: 5’ GGC CAT CCA CAG TCT TCT GAG 3’) (NCBI, NM_002046.5), ICAM-1 (forward: 5’ GGC CGG CCA GCT TAT ACA C 3’, reverse: 5’ TAG ACA CTT GAG CTC GGG CA 3’) (NCBI, NM_000201.2), and VCAM-1 (forward: 5’ TCA GAT TGG AGA CTC AGT CAT GT 3’, reverse: 5’ ACT CCT CAC CTT CCC GCT C 3’) (NCBI, NM_001078.3). Data acquisition was performed using an Applied Biosystems 7500 RT–PCR machine (Applied Biosystems, Foster City, CA, USA), and the cycle threshold was obtained by ABi 7500 Real Time-PCR software (Applied Biosystems, Foster City, CA, USA).
Statistical analyses
The data obtained were analyzed using GraphPad 7 Prism software (De Novo, San Diego, CA, USA). Data are shown as the mean ± SD. Mean differences between the two groups were tested using a paired t-test. *P < 0.05, **P < 0.01, ***P < 0.001 and ****P < 0.0001 were considered significant.
Results
Monocyte subtypes exhibit different cytokine secretion in the presence of mMPs6 mMPs. We found that culturing mMPs derived from stimulated U937 cells with their origin cells resulted in low secretion of IL-1β compared to U937 cells alone (p < 0.05) (Figure 1 A). In contrast, the secretion of IL-1β in the supernatants of stimulated THP-1-derived mMPs cultured with their origin cells was significantly increased compared to that of THP-1 cells cultured alone (p < 0.01) (Figure 1 B). However, mMPs derived from both stimulated U937 and THP-1 cells cultured with U937 and THP-1 cells failed to induce TNF-α secretion despite TNF-α being detected in the culture supernatants of U937 and THP-1 cells alone (data not shown).
We then tested whether mMPs derived from monocytes enhanced TNF-α and IL-6 secretion. The release of TNF-α from unstimulated monocytes in the presence of 1x106 mMPs was low compared to that from unstimulated monocytes without mMPs (p < 0.01) (Figure 1 C). Similarly, TNF-α production from stimulated monocytes cultured with mMPs was also low compared to that from stimulated monocytes in the absence of mMPs (p < 0.01). IL-6 production by unstimulated monocytes cultured with mMPs was significantly higher than that from unstimulated monocytes cultured without mMPs (p < 0.05) (Figure 1 D). Meanwhile, IL-6 production from the supernatants of stimulated monocytes cultured with mMPs was also higher than that from stimulated monocytes cultured without mMPs, although the difference was not significant.
Monocytic MPs enhance CD31, ICAM-1, and VCAM-1 expression by endothelial cells
Monocyte–endothelial cell interactions are crucial in inflammation. We therefore assessed whether monocyte-derived mMPs alter the expression of CD31, ICAM-1, and VCAM-1 by endothelial cells. Under resting conditions, CD31 was highly expressed (up to 97.98%) on endothelial cells, but Annexin-V expression was low (Figure 2 A). Upon stimulation with mMPs derived from LPS-stimulated monocytes, the expression of CD31+/Annexin-V+ was increased to 11.72% (Figure 2 B). Endothelial cells also expressed high levels of CD31 in the presence of mMPs derived from LPS-stimulated monocytes, which were 46.15-fold ± 2.555-fold (p < 0.05) higher than in the presence of LPS alone (Figure 2 C). Under serum-free conditions, mMPs were able to govern the expression of adhesion molecules by endothelial cells. The expression levels of ICAM-1 (Figure 2 D) and VCAM-1 (Figure 2 E) were increased 24.42-fold ± 8.989-fold and 86.49-fold ± 14.76-fold (p < 0.05), respectively, in the presence of mMPs derived from LPS-stimulated monocytes compared to those modulated by LPS alone.
Monocytic MPs enhance eMP production by endothelial cells
After demonstrating the capability of mMPs to enhance the expression of endothelial cell markers, we assessed whether mMPs were capable of inducing vesiculation in eMPs. In the absence of stimulants, resting endothelial cells expressed 14.99% CD31+/Annexin-V+ eMPs (Figure 3 A). Following LPS stimulation, endothelial cells expressed 22.88% CD31+/Annexin-V+ eMPs (Figure 3 B). Interestingly, endothelial cells cultured with unstimulated monocyte-derived mMPs resulted in 17.31% CD31+/Annexin-V+ eMPs (Figure 3 C). As expected, the stimulation of endothelial cells with mMPs derived from LPS-stimulated monocytes increased CD31+/Annexin-V+ eMP shedding by approximately 31.28% (Figure 3 D), which was higher than that released upon LPS stimulation.
Discussion
Recently, the study of mMPs has become important due to their association with monocyte and endothelial cell activation, particularly in inflammation. Emerging evidence has demonstrated the proinflammatory effects of MPs derived from distinct origin cells on monocytes and macrophages5, 14, 15. However, the interaction between mMPs and monocytes at inflammatory sites has not been explored. Therefore, the effects of mMPs during culture with their origin cells were assessed. In this study, LPS was used to induce the activation of CD14 on monocytes and mimic cellular inflammation, which subsequently produces mMPs. To isolate mMPs, cultured supernatants containing mMPs were subjected to two-step differential centrifugation at 500 xg and 1,500 xg, followed by ultracentrifugation at 20,000 xg as previously recommended16. Two-step differential centrifugation at 500 xg and 1,500 xg is crucial for removing cells and apoptotic bodies17, respectively, from mMPs in supernatants. Meanwhile, ultracentrifugation at 20,000 xg during mMP isolation eliminates exosome contamination and ensures proper separation, since mMPs are pelleted while exosomes remain in the supernatants18.
Our data showed that in the presence of mMPs derived from THP-1 cells and blood monocytes, IL-1β and IL-6, respectively, were secreted. In contrast, mMPs derived from U937 cells were unable to modulate the secretion of IL-1β by U937 cells despite having an mMP concentration comparable to THP-1 cells. In addition, the presence of mMPs did not trigger TNF-α secretion by U937, THP-1, or monocytes. These data indicate that mMPs may modulate proinflammatory cytokine secretion in a cell-origin-dependent manner. It has been previously reported that upon culture with MP, distinct cell types may release varying levels of different cytokines19. The variations in cytokine expression detected in our study were not surprising since U937 and THP-1 cells show different proteomic and cytokine profiles than blood monocytes20 and thus play different roles in inflammation. Similar to our study, a previous finding showed that mMPs containing IL-1 trigger the release of IL-1β from monocytes in an autocrine manner9. Although the exact underlying mechanism of monocyte activation by mMPs remains unknown, it is possible that mMPs contribute to an inflammatory response via their lipid fraction, which triggers the activation of Toll-like receptor 4 on monocytes as previously reported21. Moreover, the influences of mMP concentration on their biological effect have been greatly emphasized previously22. In this study, 3x106 THP-1-derived mMPs and 1x106 blood monocyte-derived mMPs activated their origin cells at different levels. Nevertheless, the correlations between different concentrations of mMPs and their biological effects on the activation of U937, THP-1, and blood monocytes were not directly assessed in this study. Several variables, including cell types, cell concentrations, and types of cytokines measured, should be considered for proper assessment in future concentration-response studies.
As elevated numbers of mMPs have been observed in inflammation23, the proinflammatory properties of mMPs in this study may be explained by their potential to enhance IL-1β and IL-6 release from monocytes, thereby exacerbating inflammation. In addition, our data have shown that mMPs inhibit the release of TNF-α by monocytes upon culture with mMPs. This finding suggests that mMPs may initiate a different mechanism that promotes an anti-inflammatory response in effector cells, as has been previously shown3. However, the pathway by which mMPs inhibit TNF-α has not been explored. A previous report has suggested that mMPs may increase the production of prostaglandin E224, resulting in the inhibition of TNF-α release by monocytes to resolve inflammation25. These data suggest that mMPs may act as TNF inhibitors, thus reducing inflammation.
Apart from cytokine release, the interaction between mMPs and endothelial cells is important in the progression of inflammation26. In this study, we therefore assessed the expression of the endothelial cell activation markers CD31, ICAM-1, and VCAM-1. Our data demonstrated that mMPs derived from LPS-stimulated monocytes upregulated CD31, ICAM-1, and VCAM-1, which is similar to a previous report on THP-19. This suggests the potential role of mMPs in activating endothelial cells in addition to exosomes27. A previous study suggested that mMPs may mediate the activation of endothelial cells via an IL-1β-dependent pathway9, although the exact mechanism was not assessed in our study. It has been previously shown that THP-1-derived mMP regulation of endothelial cells involves the activation of intracellular signaling pathways by ERK1/2 phosphorylation and IĸB-α degradation, resulting in NF-ĸB translocation and leading to the increased expression of ICAM-1, VCAM-1, and E-selectin by endothelial cells9. Additionally, our results suggest that mMPs may contribute to the amplification of inflammation and vascular injury, as previously reported28, by elevating the expression of adhesion molecules and by facilitating monocyte migration through endothelial cells to sites of inflammation29.
Additionally, as mMPs are also released constitutively under physiological conditions, especially during cell growth30, 31, their roles in physiology during steady state should not be ignored. It has been previously reported that under normal conditions, MPs derived from platelets mediate the transfer of adhesion molecules from platelets to hematopoietic cells and endothelial cells, enhancing their adhesion capacity and engraftment32. Similarly, we found that CD31, ICAM-1, and VCAM-1 expression by endothelial cells can be enhanced by mMPs derived from unstimulated monocytes. This finding suggests that this mMP may aid in cell adhesion to endothelial cells.
The ability of mMPs to induce vesiculation in eMPs, in addition to CD31 and adhesion molecule expression in endothelial cells, was also assessed. This study demonstrates that mMPs enhance the release of eMPs in culture conditions with endothelial cells, suggesting that they may be involved in the exacerbation of pulmonary and capillary leakage as previously demonstrated in vivo33. A previous report showed that eMP elevation is a hallmark of endothelial cell activation34, while others have demonstrated that mMPs are able to induce the release of eMPs in an in vitro brain inflammation model9. Taken together, mMPs are not only cell residues but are also capable of altering the responses of their effector cells by acting as biological mediators in cell-to-cell interactions22.
Conclusions
This is the first study conducted using human blood monocyte-derived mMPs on monocyte and endothelial cell activation. Monocytic MPs cause deleterious effects, particularly under pathological conditions. Monocytic MPs derived from different origin cells may display distinct cytokine profiles, thus regulating effector cells via different cytokine mechanisms. Additionally, the ability of mMPs derived under pathogenic conditions to elicit high expression of adhesion molecules by endothelial cells and to induce vesiculation of eMPs may be the key to worsening inflammation, thus promoting the progression of endothelial dysfunction. However, further studies are necessary to better understand the underlying mechanism mediated by mMPs in inflammation.
Abbreviations
eMP: Endothelial microparticles, ERK1/2: Extracellular regulated kinase 1/2, HUVEC: Human umbilical vein endothelial cells, ICAM-1: Intracellular adhesion molecule 1, IL-1: Interleukin 1, LPS: Lipopolysaccharides, MP: Microparticles, mMP: Monocytic microparticles, NF-ĸB: Nuclear Factor ĸB, TNF- α: Tumor necrosis factor α, TLR: Toll-like receptor VCAM-1: Vascular cell adhesion molecule 1
Acknowledgments
NAMN was supported by the USM Fellowship scheme and USM Graduate Assistance scheme. NAFMA was supported by MyBrain15 and USM Graduate Assistance scheme.
Author’s contributions
Conceptualization and design of the study: Rapeah Suppian and Maryam Azlan
Acquisition of data: Nur Azira Mohd Noor, Siti Zulaiha Marhalim, and Nur Azrah Fazera Mohd Ariffin.
Analysis and/or interpretation of data: Nur Azira Mohd Noor, Siti Zulaiha Marhalim and Nur Azrah Fazera Mohd Ariffin.
Drafting the manuscript: Nur Azira Mohd Noor, Siti Zulaiha Marhalim, and Nur Azrah Fazera Mohd Ariffin.
Revising the manuscript critically for important intellectual content: Nur Azira Mohd Noor and Maryam Azlan
Approval of the version of the manuscript to be published: Nur Azira Mohd Noor, Siti Zulaiha Marhalim, Nur Azrah Fazera Mohd Ariffin, Rapeah Suppian, and Maryam Azlan
All authors read and approved the final manuscript.
Funding
This work was supported by the Short Term Grant, Universiti Sains Malaysia (Grant number: 304/PPSK/61313135).
Availability of data and materials
Data and materials used and/or analyzed during the current study are available from the corresponding author on reasonable request.
Ethics approval and consent to participate
Not applicable.
Consent for publication
Not applicable.
Competing interests
The authors declare that they have no competing interests.
References
-
Mutalib
M.N. Abd,
Marhalim
S.Z.,
Bakar
N. Abu,
Azlan
M.,
Monocytic microparticles (mMP) derived from different monocyte subsets display similar morphology and size. Malaysian Journal of Microscopy.
2020;
16
(1)
:
188-95
.
-
Favretto
G.,
Cunha
R.S.,
Dalboni
M.A.,
Oliveira
R.B.,
Barreto
F.C.,
Massy
Z.A.,
Endothelial microparticles in uremia: biomarkers and potential therapeutic targets. Toxins.
2019;
11
(5)
:
267
.
View Article PubMed Google Scholar -
Halim
A.T.,
Ariffin
N.A.,
Azlan
M.,
The multiple roles of monocytic microparticles. Inflammation.
2016;
39
(4)
:
1277-84
.
View Article PubMed Google Scholar -
Dignat-George
F.,
Boulanger
C.M.,
The many faces of endothelial microparticles. Arteriosclerosis, Thrombosis, and Vascular Biology.
2011;
31
(1)
:
27-33
.
View Article PubMed Google Scholar -
Hezel
M.E.,
Nieuwland
R.,
Bruggen
R.V.,
Juffermans
N.P.,
The ability of extracellular vesicles to induce a pro-inflammatory host response. International Journal of Molecular Sciences.
2017;
18
(6)
:
1285
.
View Article PubMed Google Scholar -
Cloutier
N.,
Tan
S.,
Boudreau
L.H.,
Cramb
C.,
Subbaiah
R.,
Lahey
L.,
The exposure of autoantigens by microparticles underlies the formation of potent inflammatory components: the microparticle-associated immune complexes. EMBO Molecular Medicine.
2013;
5
(2)
:
235-49
.
View Article PubMed Google Scholar -
Kaneko
N.,
Kurata
M.,
Yamamoto
T.,
Morikawa
S.,
Masumoto
J.,
The role of interleukin-1 in general pathology. Inflammation and Regeneration.
2019;
39
(1)
:
12
.
View Article PubMed Google Scholar -
Turner
M.D.,
Nedjai
B.,
Hurst
T.,
Pennington
D.J.,
Cytokines and chemokines: at the crossroads of cell signalling and inflammatory disease. Biochimica et Biophysica Acta.
2014;
1843
(11)
:
2563-82
.
View Article PubMed Google Scholar -
Wang
J.G.,
Williams
J.C.,
Davis
B.K.,
Jacobson
K.,
Doerschuk
C.M.,
Ting
J.P.,
Monocytic microparticles activate endothelial cells in an IL-1β-dependent manner. Blood.
2011;
118
(8)
:
2366-74
.
View Article PubMed Google Scholar -
Edrissi
H.,
Schock
S.C.,
Hakim
A.M.,
Thompson
C.S.,
Microparticles generated during chronic cerebral ischemia increase the permeability of microvascular endothelial barriers in vitro. Brain Research.
2016;
1634
:
83-93
.
View Article PubMed Google Scholar -
Bernimoulin
M.,
Waters
E.K.,
Foy
M.,
Steele
B.M.,
Sullivan
M.,
Falet
H.,
Differential stimulation of monocytic cells results in distinct populations of microparticles. Journal of Thrombosis and Haemostasis.
2009;
7
(6)
:
1019-28
.
View Article PubMed Google Scholar -
Lovren
F.,
Verma
S.,
Evolving role of microparticles in the pathophysiology of endothelial dysfunction. Clinical Chemistry.
2013;
59
(8)
:
1166-74
.
View Article PubMed Google Scholar -
Deng
F.,
Wang
S.,
Zhang
L.,
Endothelial microparticles act as novel diagnostic and therapeutic biomarkers of circulatory hypoxia-related diseases: a literature review. Journal of Cellular and Molecular Medicine.
2017;
21
(9)
:
1698-710
.
View Article PubMed Google Scholar -
Schildberger
A.,
Rossmanith
E.,
Eichhorn
T.,
Strassl
K.,
Weber
V.,
Monocytes, peripheral blood mononuclear cells, and THP-1 cells exhibit different cytokine expression patterns following stimulation with lipopolysaccharide. Mediators of Inflammation.
2013;
2013
:
697972
.
View Article Google Scholar -
Joerger-Messerli
M.S.,
Hoesli
I.M.,
Rusterholz
C.,
Lapaire
O.,
Stimulation of monocytes by placental microparticles involves toll-like receptors and nuclear factor kappa-light-chain-enhancer of activated B cells. Frontiers in Immunology.
2014;
5
:
173
.
View Article PubMed Google Scholar -
Crompot
E.,
Van Damme
M.,
Duvillier
H.,
Pieters
K.,
Vermeesch
M.,
Perez-Morga
D.,
Avoiding false positive antigen detection by flow cytometry on blood cell derived microparticles: the importance of an appropriate negative control. PLoS One.
2015;
10
(5)
:
e0127209
.
View Article PubMed Google Scholar -
Weilner
S.,
Schraml
E.,
Redl
H.,
Grillari-Voglauer
R.,
Grillari
J.,
Secretion of microvesicular miRNAs in cellular and organismal aging. Exp Gerontol.
2012;
48
(7)
:
626-633
.
View Article PubMed Google Scholar -
Menck
K.,
Bleckmann
A.,
Schulz
M.,
Ries
L.,
Binder
C.,
Isolation and characterization of microvesicles from peripheral blood. Journal of Visualized Experiments.
2017;
:
55057
.
View Article Google Scholar -
Paudel
K.R.,
Panth
N.,
Kim
D.W.,
Circulating endothelial microparticles: A key hallmark of atherosclerosis progression. Scientifica (Cairo).
2016;
2016
:
8514056
.
View Article PubMed Google Scholar -
Distler
J.H.,
Pisetsky
D.S.,
Huber
L.C.,
Kalden
J.R.,
Gay
S.,
Distler
O.,
Microparticles as regulators of inflammation: novel players of cellular crosstalk in the rheumatic diseases. Arthritis and Rheumatism.
2005;
52
(11)
:
3337-48
.
View Article PubMed Google Scholar -
Thomas
L.M.,
Salter
R.D.,
Activation of macrophages by P2X7-induced microvesicles from myeloid cells is mediated by phospholipids and is partially dependent on TLR4. Journal of Immunology (Baltimore, Md.: 1950).
2010;
185
(6)
:
3740-9
.
View Article PubMed Google Scholar -
Wen
B.,
Combes
V.,
Bonhoure
A.,
Weksler
B.B.,
Couraud
P.O.,
Grau
G.E.,
Endotoxin-induced monocytic microparticles have contrasting effects on endothelial inflammatory responses. PLoS One.
2014;
9
(3)
:
e91597
.
View Article PubMed Google Scholar -
Bardelli
C.,
Amoruso
A.,
Federici Canova
D.,
Fresu
L.,
Balbo
P.,
Neri
T.,
Autocrine activation of human monocyte/macrophages by monocyte-derived microparticles and modulation by PPARγ ligands. British Journal of Pharmacology.
2012;
165
(3)
:
716-28
.
View Article PubMed Google Scholar -
Jüngel
A.,
Distler
O.,
Schulze-Horsel
U.,
Huber
L.C.,
Ha
H.R.,
Simmen
B.,
Microparticles stimulate the synthesis of prostaglandin E(2) via induction of cyclooxygenase 2 and microsomal prostaglandin E synthase 1. Arthritis and Rheumatism.
2007;
56
(11)
:
3564-74
.
View Article PubMed Google Scholar -
Linke
B.,
Schreiber
Y.,
Picard-Willems
B.,
Slattery
P.,
Nüsing
R.M.,
Harder
S.,
Activated platelets induce an anti-inflammatory response of monocytes/macrophages through cross-regulation of PGE2 and cytokines. Mediators Inflamm.
2017;
2017
:
1463216
.
View Article PubMed Google Scholar -
Morel
O.,
Toti
F.,
Morel
N.,
Freyssinet
J.M.,
Microparticles in endothelial cell and vascular homeostasis: are they really noxious?. Haematologica.
2009;
94
(3)
:
313-7
.
View Article PubMed Google Scholar -
Sáez
T.,
de Vos
P.,
Kuipers
J.,
Sobrevia
L.,
Faas
M.M.,
Exosomes derived from monocytes and from endothelial cells mediate monocyte and endothelial cell activation under high d-glucose conditions. Immunobiology.
2019;
224
(2)
:
325-33
.
View Article PubMed Google Scholar -
Reid
V.L.,
Webster
N.R.,
Role of microparticles in sepsis. British Journal of Anaesthesia.
2012;
109
(4)
:
503-13
.
View Article PubMed Google Scholar -
Vestweber
D.,
How leukocytes cross the vascular endothelium. Nature Reviews. Immunology.
2015;
15
(11)
:
692-704
.
View Article PubMed Google Scholar -
Camussi
G.,
Deregibus
M.C.,
Bruno
S.,
Cantaluppi
V.,
Biancone
L.,
Exosomes/microvesicles as a mechanism of cell-to-cell communication. Kidney International.
2010;
78
(9)
:
838-48
.
View Article PubMed Google Scholar -
Ratajczak
J.,
Wysoczynski
M.,
Hayek
F.,
Janowska-Wieczorek
A.,
Ratajczak
M.Z.,
Membrane-derived microvesicles: important and underappreciated mediators of cell-to-cell communication. Leukemia.
2006;
20
(9)
:
1487-95
.
View Article PubMed Google Scholar -
Baj-Krzyworzeka
M.,
Majka
M.,
Pratico
D.,
Ratajczak
J.,
Vilaire
G.,
Kijowski
J.,
Platelet-derived microparticles stimulate proliferation, survival, adhesion, and chemotaxis of hematopoietic cells. Experimental Hematology.
2002;
30
(5)
:
450-9
.
View Article PubMed Google Scholar -
Densmore
J.C.,
Signorino
P.R.,
Ou
J.,
Hatoum
O.A.,
Rowe
J.J.,
Shi
Y.,
Endothelium-derived microparticles induce endothelial dysfunction and acute lung injury. Shock (Augusta, Ga.).
2006;
26
(5)
:
464-71
.
View Article PubMed Google Scholar -
Wassmer
S.C.,
Combes
V.,
Candal
F.J.,
Juhan-Vague
I.,
Grau
G.E.,
Platelets potentiate brain endothelial alterations induced by Plasmodium falciparum. Infection and Immunity.
2006;
74
(1)
:
645-53
.
View Article PubMed Google Scholar
Comments
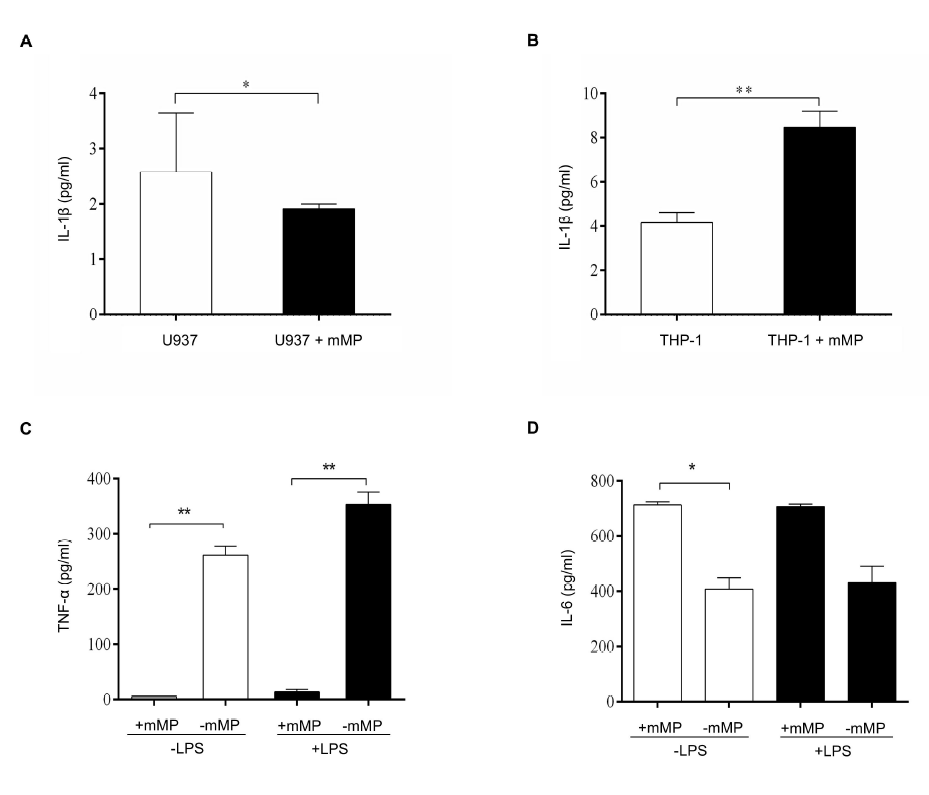
Article Details
Volume & Issue : Vol 9 No 6 (2022)
Page No.: 5121-5129
Published on: 2022-06-30
Citations
Copyrights & License

This work is licensed under a Creative Commons Attribution 4.0 International License.
Search Panel
Pubmed
Google Scholar
Pubmed
Google Scholar
Pubmed
Google Scholar
Pubmed
Google Scholar
Pubmed
Search for this article in:
Google Scholar
Researchgate
- HTML viewed - 3231 times
- PDF downloaded - 1195 times
- XML downloaded - 0 times