Abstract
Introduction: The natural process of aging has an impact on people?s quality of life. The skin is among the body parts that age the fastest and is affected by both endogenous and external influences. Therefore, it is necessary to study the capacity of various natural extracts to protect against skin aging. This study aimed to evaluate the protective effects of Boesenbergia pandurata extract (BPE) against UVB-induced skin aging in a murine model.
Methods: The maceration process was used to isolate BPE, and its stability was assessed using liquid chromatography-mass spectrometry (LC-MS). Balb/C mice were treated with various doses of BPE (50 mg/kg, 100 mg/kg, and 150 mg/kg) after being exposed to UVB at a rate of 300 mJ/cm2 to establish a skin photoaging model. The skin?s appearance and tissue and cell morphology were evaluated using hematoxylin and eosin (H&E) staining and Masson?s trichrome staining, respectively.
Results: The results showed that the treatment groups did not exhibit any major signs of aging, such as wrinkles, dryness, or roughness, whereas the UVB-exposed group did. Due to the cleavage of collagen fibers, Masson?s trichrome staining revealed a lack of connective tissue in the UVB-only group; additionally, H&E staining revealed abnormalities in the cellular structure of the tissue. On the other hand, the treatment groups? outcomes were consistent and comparable to those of the control group according to the H&E and Masson?s trichrome staining results.
Conclusion: Overall, our findings show that BPE, especially at a dose of 50 mg/kg, can successfully protect skin from UVB-induced aging, providing a potential strategy for the development of natural anti-aging products.
Introduction
UV light is a notable form of radiation that can expedite the aging of the skin. Exposure to UVB radiation, a specific type of UV radiation that can harm DNA and produce oxidative stress in skin cells, can induce the accumulation of mutations and the breakdown of collagen and elastin filaments in the skin1, 2. Research on mice with aged skin has shown that exposure to UVB radiation can cause wrinkles, hanging skin, and hyperpigmentation3. To mitigate the adverse effects of UVB, researchers have evaluated a range of naturally occurring compounds with antioxidant and protective capacities. One such product is Boesenbergia pandurata, a plant local to Southeast Asia that has for quite some time been valued for its therapeutic properties. Boesenbergia pandurata extract (BPE) may help treat or prevent UVB-induced skin aging as recent research indicates that it has potent anti-inflammatory and antioxidant properties4.
To simulate the impacts of UVB radiation on human skin, researchers have created a mouse model of skin aging5. Reactive oxygen species (ROS) and DNA damage are produced because of UVB exposure according to studies done on mice, and these effects can speed up the aging process of the epidermis. According to one study, mice exposed to UVB developed wrinkles and had lower collagen levels and more elastin fragmentation—all signs of aging skin5.
Researchers have been able to identify numerous substances that can protect against UVB-induced skin damage using this model. Boesenbergia pandurata contains one such compound. This plant’s extracts have been found to have antioxidant and anti-inflammatory properties, which may help prevent skin aging and UVB-induced damage6, 7. The extract may have the ability to protect against UVB-induced skin damage and have cell-reinforcing qualities. Further research is needed to fully understand the potential benefits of Boesenbergia pandurata regarding skin aging. Therefore, this study aimed to evaluate its protective effects against UVB-induced skin aging in a murine model.
Methods
Experimental Animals
Male Balb/c mice, aged 6–8 weeks, with an average weight of 23–25 g, were used in this study. The mice were obtained from the Laboratory of Animal Care and Usage (LACU) of the Stem Cell Institute, University of Science, Viet Nam National University Ho Chi Minh City, and were housed in clean cages in a controlled environment with food and water. The light/dark cycle was maintained at 12/12. The experimental protocol involving the animals was reviewed and approved by the ethics committee of the Stem Cell Institute (HCMUS, VNUHCM, VN).
Preparation of Boesenbergia pandurata
Boesenbergia pandurata plants were collected from gardens in the Southwest region and identified at the Southern Institute of Ecology. The extract was evaluated by high-pressure liquid chromatography (LC-MS) at the central laboratory of the University of Natural Sciences, Vietnam National University, Ho Chi Minh City (Supplement).
Boesenbergia pandurata extract preparation
The collected plant material was cleaned, dried, and then ground into a fine powder. Five hundred grams of powder was extracted with 1500 mL of absolute ethanol using a macerated method for 72h with constant shaking. The extract was then filtered and freezing dry at condition 1 atm, -40 oC. The dry extract was dissolved in absolute to obtain a final concentration of 50 mg/mL, 100 mg/mL and 150 mg/mL. The solution was stored at 4 oC until use.
Mouse model of skin aging
Fifty four male Balb/c mice aged 6 – 8 weeks with an average weight of 23–25 g were used to create a mouse model of skin aging. The mice were housed in a controlled environment for 2 weeks and were randomly divided into six groups (Figure 1). UVB irradiation was used to induce skin aging. The UVB source had a wavelength of 275 – 380 nm, was placed 30 cm away from the mice, and had an intensity of 300 mJ/cm2. The experimental design and UVB intensity were based on studies by Kim et al. (2012)8. The mice were exposed to UVB every 3 days. The experimental and placebo groups received 10 µL of the BPE and solvent, respectively; the agent was applied to the skin. The mice in the negative control group were shaved every two weeks to maintain hairlessness but received no further treatment, while the positive control group received only UVB irradiation.
Experimental design
The experimental group consisted of three groups, each comprising three randomly chosen mice, according to the concentration of the extract used (50 mg/mL, 100 mg/mL, and 150 mg/mL) (Figure 1). The mice were shaved every 2 weeks to maintain hairlessness, and the extract was applied at the designated concentration and time for each batch at this location throughout the experiment. In addition, the mice were exposed to UVB simultaneously at the same intensity and frequency as in the experimental setup of the mouse model of aging.
After 4 and 8 weeks of experiments, three mice from each group were randomly selected and evaluated for skin aging parameters such as skin tension, wrinkles, and skin thickness in the experimental area. The mice were euthanized according to the animal ethics committee’s requirements by cervical dislocation, and skin samples were collected.
Administration of macerated Boesenbergia pandurata extract
The macerated BPE was administered topically to the mice in the experimental groups at concentrations of 50 mg/mL, 100 mg/mL, and 150 mg/mL. The extract was treated every three day before applied UVB on skin designated area for 4 weeks and 8 weeks. The mice were weighed weekly, and calipers with an error of 0.01 cm were used to measure mouse skin thickness every week. The level of skin aging of the mice was also visually assessed.
H&E staining
Skin samples were collected from the experimental and control groups and immediately fixed in 10% buffered formalin solution for 24 hours. Thereafter, the samples were dehydrated in ascending grades of alcohol and embedded in paraffin wax. The paraffin blocks were then cut into 5-μm sections and mounted on glass slides. The sections were then deparaffinized in xylene and rehydrated in descending grades of alcohol, following which they were stained with hematoxylin and eosin (H&E) for 10 minutes, rinsed in water, dehydrated, and mounted with a coverslip. The slides were then observed under a light microscope, and images were captured using a high-resolution camera. The images were analyzed using ImageJ software to quantify the epidermal thickness, dermal thickness, and number of fibroblasts.
Masson’s trichrome staining
Skin samples were collected from the experimental and control groups and immediately fixed in 10% buffered formalin solution for 24 hours. After that, the samples were dehydrated in ascending grades of alcohol and embedded in paraffin wax. The paraffin blocks were then cut into 5-μm sections and mounted on glass slides. The sections were then deparaffinized in xylene and rehydrated in descending grades of alcohol, after which they were stained with Masson’s trichrome stain for 10 minutes, rinsed in water, dehydrated, and mounted with a coverslip. The slides were then observed under a light microscope, and images were captured using a high-resolution camera. The images were analyzed using ImageJ software to quantify the collagen content and collagen fiber distribution.
Statistical analysis
All data were expressed as mean ± standard deviation (SD). Statistical analysis was performed using two-way ANOVA followed by Tukey’s multiple comparison test. Differences were considered statistically significant at P < 0.05. All statistical analyses were performed using GraphPad Prism software (version 9.0).
Results
Effects of UVB and Boesenbergia pandurata extract on skin hydration
From week 0 to week 8, the group 1’s skin moisture levels slightly increased, possibly due to cyclical changes in the environment or a study-uncontrolled environmental component (Figure 2A-C). However, from week 4 to week 8, the skin moisture levels in the UVB (group 2) (Figure 2D-F) and placebo (group 3) (Figure 2G-I) groups both decreased. This indicates that epidermal moisture levels in mice were adversely affected by UVB exposure and the application of a placebo.
At week 4, the T50 (group 4) and T150 (group 6) groups displayed a small rise in skin hydration levels, which may have been brought on by the skin hydration extract’s effects (Figure 2K-M, Q-S). This improvement was not long-lasting, as week 8 revealed a decline in skin moisture in both groups. Thus, the skin hydration extract may have provided some short-term advantages that were not sustained over time.
The T100 group (group 5) showed a consistent decrease in skin hydration levels over the course of the study, indicating that a higher dose of the skin hydration extract may have had a negative effect on skin hydration levels (Figure 2N-P).
Week | Control | UVB | Placebo | T50 | T100 | T150 |
---|---|---|---|---|---|---|
0 | 22.4 ± 0.66 | 24.8 ± 2.4 | 24.7 ± 2.1 | 25.1 ± 0.2 | 24.8 ± 0.2 | 24.4 ± 0.9 |
4 | 28.1 ± 0.5 | 29.1 ± 1.1 | 27.6 ± 3.4 | 28.3 ± 1.2 | 27.5 ± 0.8 | 28.8 ± 2.6 |
8 | 31.4 ± 2.2 | 29.9 ± 1.9 | 30.2 ± 1.2 | 31.6 ± 0.6 | 34.2 ± 0.8 | 31.7 ± 2.6 |
Effects of UVB and extracts on mouse weight
Table 1 and Figure 3 show that in week 0, mice in the UVB group (Group 2) had a higher mean weight than those in the control group (Group 1), although mice in the treatment groups (Group 4, 5 and 6) had a mean weight that was similar to that of the control group (Group 1) (p > 0.05). At this starting time point, plant extract and UVB do not show any effect on mice skin.
At week 4, the mice in the UVB group (Group 2) continued to weigh more on average than the control group’s mice, while the weights of the mice in the treatment groups remained practically identical to those of the control group (Group 1). This shows that while UVB exposure continued to affect weight, various concentrations of plant extract did not.
While the mean weight of mice in the T100 group (Group 5) had significantly risen in contrast with the control group (Group 1) by week 8, the mean weight of mice in the UVB group (Group 2) had decreased relative to the previous week. This shows that while a plant extract concentration of 100 mg/mL might positively affect weight over time, UVB exposure might have adverse consequences over the long term.
Overall, it seems that UVB radiation initially stimulated weight gain but had a negative impact over time. On the other hand, the plant extract doses had no discernible impact on weight gain, with the exception of the 100 mg/mL dose, which at week 8 induced a modest increase in weight.
Week | Control | UVB | Placebo | T50 | T100 | T150 |
---|---|---|---|---|---|---|
0 | 0.57 ± 0.06 | 0.53 ± 0.06 | 0.63 ± 0.06 | 0.63 ± 0.06 | 0.63 ± 0.1 | 0.67 ± 0.1 |
4 | 0.63 ± 0.06 | 0.9 ± 0.1 d | 0.73 ± 0.58 b | 0.61 ± 0.05 | 0.51 ± 0.07 | 0.61 ± 0.1 |
8 | 0.73 ± 0.1 | 1.1 ± 0.1 d | 0.9 ± 0.1 | 0.73 ± 0.1 | 0.73 ± 0.06 | 0.77 ± 0.06 |
Effects of UVB and extracts on mouse skin thickness
Table 2 and Figure 4 show that there were no discernible variations in skin thickness between the treatment groups and the control group at week 0. Even though the level of the increase in thickness varied depending on the dose, all treatment groups (Group 4, 5 and 6) at week 4 demonstrated increases in skin thickness in comparison to the control group (Group 1). In particular, the skin thickness increased in the T50 (Group 4) and T150 (Group 6) groups by 0.08 to 0.14 mm, whereas it decreased somewhat in the T100 (Group 5) group.
At week 4, the UVB group experienced the greatest increase in skin thickness (0.37 to 0.1 mm). At week 8, the treatment groups (Groups 4, 5 and 6) showed continued increases in skin thickness compared with the control group (Group 1), with the highest increase in the T150 group (Group 6) (0.14 ± 0.06 mm). The T50 group (Group 4) likewise showed a significant increase in skin thickness compared with the control group (Group 1) (0.16 ± 0.1 mm), while the T100 group showed no significant change in skin thickness. Again, the UVB group (Group 2) showed the greatest increase in skin thickness of all groups at week 8 (0.53 ± 0.1 mm). The UVB group (Group 2) showed a continuous increase in skin thickness, resulting in a two-fold increase compared to the initial thickness after 2 months. Moreover, the UVB group (Group 2) had skin 1.5 times thicker than that of the control (Group 1) and placebo (Group 3) groups.
Week | Control | UVB | Placebo | T50 | T100 | T150 |
---|---|---|---|---|---|---|
0 | 1.03 ± 0.17 | 1.39 ± 0.23 | 0.93 ± 0.1 | 0.68 ± 0.13 | 0.58 ± 0.027 | 0.69 ± 0.02 |
4 | 0.89 ± 0.11 | 0.83 ± 0.04 | 0.81 ± 0.05 | 0.69 ± 0.09 | 0.85 ± 0.037 | 0.71 ± 0.09 |
8 | 0.78 ± 0.07 | 0.98 ± 0.18 | 0.88 ± 0.03 | 0.89 ± 0.1 | 0.81 ± 0.057 | 0.77 ± 0.0391 |
Impact of UVB on mouse skin elasticity
Based on the data in Table 3 and Figure 5, we can analyze the effect of the treatments on the recovery time of mouse skin elasticity after exposure to UV radiation and explore the potential of BPE to treat skin aging. The control group was not exposed to any treatment or intervention, and therefore, there were no extraneous factors that could have interfered with skin recovery.
The UVB group (Group 2) consistently had the longest recovery time at all time points. This finding is consistent with the well-known harmful effects of UV radiation on the skin, including the disruption of collagen fibers and the breakdown of elastin, which can lead to decreased skin elasticity and increased signs of aging.
The groups treated with the BPE (Group 4, 5 and 6) had recovery times that were similar to those of the control group (Group 1) and lower than group 2 and group 3. This suggests that the extract may have a protective effect on the skin and could potentially be useful for preventing or treating skin damage caused by UV radiation. The ability of the extract to improve the recovery time of mouse skin elasticity after UV radiation exposure is a promising indication of its potential anti-aging properties.
Based on the data table provided, there appear to be some differences in the effects of different doses of BPE (Groups 4, 5 and 6) on skin elasticity recovery after UV radiation exposure.
At week 0, the baseline measurement, the T50 group (Group 4) had a recovery time of 0.68 ± 0.13, which was slightly lower than that of the T100 (Group 5) and T150 (Group 6) groups (0.58 ± 0.027 and 0.69 ± 0.02, respectively). However, it is important to note that the differences between the groups at this time point were not statistically significant (p > 0.05), as the confidence intervals for each group overlapped with one another.
At week 4, there appeared to be a trend towards shorter recovery times in the T100 (Group 5) and T150 (Group 6) groups compared to the T50 group (Group 4). Specifically, the T100 group had a recovery time of 0.85 ± 0.037, which was higher than that of the T150 group (0.71 ± 0.09). However, both these groups had lower recovery times than the T50 group (0.69 ± 0.09). Again, the differences between the groups at this time point were not statistically significant (p > 0.05).
At week 8, the differences between the groups became more apparent. The T50 (Group 4) group had a recovery time of 0.89 ± 0.1, which was higher than that of both the T100 group (Group 5) (0.81 ± 0.057) and the T150 group (Group 6) (0.77 ± 0.0391) (p < 0.05). In fact, the groups 5 and 6 had recovery times that were similar to that of the control group (Group 1) (0.78 ± 0.07), indicating that these doses of BPE may be effective in promoting skin elasticity recovery after UV radiation exposure.
Week | Control | UVB | Placebo | T50 | T100 | T150 |
---|---|---|---|---|---|---|
0 | 0 | 0 | 0 | 0 | 0 | 0 |
4 | 0 | 2.33 ± 0.57 | 2.67 ± 0.57 | 1.67 ± 0.57 | 1 | 1 |
8 | 0 | 2.67 ± 0.57 | 3 | 1 | 1 | 1 |
A general evaluation of mouse skin aging
Table 4 and Figure 6 present the results of an experiment on skin aging in which different treatments were applied to different groups, and the effects of these treatments were measured at different time points. The groups included a control group (Group 1), a group exposed to UVB radiation (Group 2), a group treated with a placebo (Group 3), and three groups treated with different doses of the extract (T50, T100, and T150) (Group 4, 5 and 5, respectively).
At the initial time point (week 0), all groups had a score of 0 for all the assessed criteria, indicating no signs of skin aging. However, at later time points (weeks 4 and 8), the groups began to show differences in their skin aging scores.
The group 2 showed an increase in skin aging scores compared to the control group (Group 1), suggesting that UVB radiation accelerates skin aging. The placebo group (Group 3) did not show any significant changes in skin aging scores compared to the group 2, suggesting that the placebo had no effect on skin aging.
At week 4, the T100 (Group 5) and T150 (Group 6) groups showed improvement in some of the assessed criteria, whereas the group 4 did not show any improvement. Specifically, the groups 4 and 5 showed improvements, but not in the group 6. This suggests that the doses of 100 mg/mL and 150 mg/mL of BPE may be more effective than the dose of 50 mg/mL of BPE in improving certain aspects of skin aging at an earlier time point.
However, at week 8, all three extract-treated groups (Groups 4, 5 and 6) showed improvement in some of the assessed criteria. This suggests that all three doses of extract may have a positive effect on skin aging at a later time point.
Overall, the experiment suggests that UVB radiation accelerates skin aging, while some doses of the plant extract may have a positive effect on skin aging. However, further studies are needed to confirm these findings and determine the optimal doses and time points for treatment.
Histological assessment
Histological evaluation was performed to investigate the effects of UVB and the extracts on the histological features of mouse skin. Figure 7, Figure 8 show representative images of skin sections from each group.
The control group showed normal histological features throughout the study, with no signs of damage or abnormalities. The UVB (Group 2) and placebo (Group 3) groups showed an increase in the number of infiltrating inflammatory cells at week 8. The T50 (Group 4) and T150 (Group 6) groups showed a slight decrease in epidermal thickness at week 8 but no increase in inflammatory cells. The T100 group (Group 5) showed a significant decrease in epidermal thickness and a significant increase in inflammatory cell infiltration at week 8. Overall, these results suggest that both exposure to UVB and the use of the extract had negative effects on the histological features of mouse skin, with the T100 group experiencing the most severe damage.
Discussion
Aging is a natural and inevitable process that begins sometime after birth. The skin, in particular, is the organ that ages the fastest since it is most exposed to the environment. Both intrinsic and extrinsic causes of skin aging have been identified9. Now, more and more external factors, such as global warming and pollution, cause skin aging, whereas internal influences are irreversible and occur over a long period during the life of the organism. UV intensity rises in the air. The biggest contributing component among them is photoaging, which is brought on by prolonged direct exposure to strong UV rays10. To better understand and evaluate the aging process of the skin, numerous aging models and methodologies have been created. Similarly, a skin aging model using Balb/C mice was developed in this work to assess BPE’s capacity to protect the skin, and the mice were divided into the UVB, T50, T100, T150, and placebo groups. Using various techniques, including imaging of the mouse skin, calipers, and common criteria, the effects of UVB radiation and plant extract on the mouse skin surface, mouse weight, skin thickness, and the overall degree of aging were investigated. Moreover, Masson’s trichrome staining and H&E staining were used to evaluate the histology.
The assessment of mouse skin thickness revealed a significant increase in the UVB-only group at weeks 0, 4, and 8, while the control and placebo groups showed slight increases over time. However, the treatment group maintained a stable skin thickness similar to that of the control group, indicating the extract’s ability to protect against UVB damage. In contrast, the UVB group’s skin thickness was 1.5 times higher than the control and treatment groups after 8 weeks, suggesting that UVB irradiation can substantially increase skin thickness. These findings are consistent with previous studies by Kim et al. (2012) and Gyöngyösi et al. (2016)8, 11. Additionally, the evaluation of skin aging revealed that the extract positively contributed to protecting the skin against UVB-induced aging, as indicated by the milder signs of aging observed in the treatment groups compared to the UVB and placebo groups at week 8. Histological analysis of H&E images showed that the UVB and placebo groups had less cohesive connective tissue structures in the dermis and loose subcutaneous tissue at week 8. In contrast, the treatment group maintained dense and tight connective tissue in the dermis and a stable epidermis with five cell layers. Therefore, the study’s results suggest that UVB irradiation and BPE use have different effects on skin structure.
Similar studies have looked into the impact of herbal extracts and UVB radiation on the structure and function of the skin. UVB light has been linked to skin melanogenesis, cell damage, and death, according to research by Ji et al.12. According to additional research done by Imokawa in 2009, UVB radiation plays a role in the development of elastic fibers, which are crucial for preserving skin integrity13. The results of a study conducted in 2020 by Torabian et al., which examined the effects of UVB radiation on mouse skin, were consistent with those of the present study. That study showed that skin thickness doubled after exposure to UVB light. Moreover, compared to the placebo and control groups, the groups that received UV irradiation and medication had higher quantities of collagen indicated by Masson’s trichrome staining. These findings are consistent with the current study and provide more evidence that UVB radiation significantly affects the structure of rat skin14. Listyawati et al. (2020) showed that BPE protected the skin of hairless rats from UVB damage. These results imply that BPE can be a potent therapeutic for UVB-induced skin damage15.
Our investigation revealed that BPE treatment plays a significant protective role in maintaining the structural and functional integrity of mouse skin tissue. It demonstrated that exposure to UVB radiation increases skin thickness and skin aging risk. It also showed that BPE helps to retain the skin’s structure and integrity.
Conclusions
The present study yields valuable insights into the potential benefits of BPE in protecting mouse skin against UVB-induced aging. The findings demonstrate that BPE, particularly at a concentration of 50 mg/kg, can effectively protect mouse skin against UVB-induced aging without causing any adverse effects or weight loss. Notably, the extract can maintain stable skin thickness, preserving tissue structure and cells.
To further advance our understanding of the skin-protective ability of BPE, larger-scale and longer-term experiments should be conducted. Molecular biology and immunohistochemistry experiments may also be performed to elucidate the mechanism of action of the extract. Additionally, building emulsifying formulations of the extracts can lead to the development of market-ready products based on these extracts. Further research in these areas has the potential to yield significant benefits for the food and cosmetic industries.
Abbreviations
BPE: Boesenbergia pandurata extract, ROS: Reative Oxygen Species, UV: UtraViolet, UVB: UtraViolet B
Acknowledgments
Thank you Dr. Nguyen Tran Quoc Trung (Southern Institute of Ecology, Ho Chi Minh City, Viet Nam) to check and confirm the Boesenbergia pandurata plant used in this study.
Author’s contributions
Nghia Minh Do performs in vivo experiments on mice. Thuy Thi Truong helps take care of mice. Phuc Hong Vo performs plant extraction. Sinh Truong Nguyen designs experiments, check results, and draft the manuscript. Phuc Van Pham supervised the study, designed the experimental design, prepared the figure 1, edited the manuscript. All authors read and approved the final manuscript.
Funding
This study was granted by Stem Cell Institute, University of Science, Viet Nam National University Ho Chi Minh City, Viet Nam under grant number 11/2019/QĐ-KHCN-SCI.
Availability of data and materials
Data and materials used and/or analyzed during the current study are available from the corresponding author on reasonable request.
Ethics approval
The study on animals was approved by ethical institutional committee of Stem Cell Institute, University of Science, Viet Nam National University Ho Chi Minh City, Viet Nam.
Consent for publication
Not applicable.
Competing interests
The authors declare that they have no competing interests.
References
-
Budden
T.,
Gaudy-Marqueste
C.,
Porter
A.,
Kay
E.,
Gurung
S.,
Earnshaw
C.H.,
Ultraviolet light-induced collagen degradation inhibits melanoma invasion. Nature Communications.
2021;
12
(1)
:
2742
.
View Article PubMed Google Scholar -
Jariashvili
K.,
Madhan
B.,
Brodsky
B.,
Kuchava
A.,
Namicheishvili
L.,
Metreveli
N.,
UV damage of collagen: insights from model collagen peptides. Biopolymers.
2012;
97
(3)
:
189-98
.
View Article PubMed Google Scholar -
Geng
R.,
Kang
S.G.,
Huang
K.,
Tong
T.,
Boosting the Photoaged Skin: The Potential Role of Dietary Components. Nutrients.
2021;
13
(5)
:
1691
.
View Article PubMed Google Scholar -
Kim
D.U.,
Chung
H.C.,
Kim
C.,
Hwang
J.K.,
Oral intake of Boesenbergia pandurata extract improves skin hydration, gloss, and wrinkling: A randomized, double-blind, and placebo-controlled study. Journal of Cosmetic Dermatology.
2017;
16
(4)
:
512-9
.
View Article PubMed Google Scholar -
Her
Y.,
Shin
B.N.,
Lee
Y.L.,
Park
J.H.,
Kim
D.W.,
Kim
K.S.,
Oenanthe Javanica Extract Protects Mouse Skin from UVB Radiation via Attenuating Collagen Disruption and Inflammation. International Journal of Molecular Sciences.
2019;
20
(6)
:
1435
.
View Article PubMed Google Scholar -
Ongwisespaiboon
O.,
Jiraungkoorskul
W.,
Fingerroot, Boesenbergia rotunda and its Aphrodisiac Activity. Pharmacognosy Reviews.
2017;
11
(21)
:
27-30
.
View Article PubMed Google Scholar -
Eng-Chong
T.,
Yean-Kee
L.,
Chin-Fei
C.,
Choon-Han
H.,
Sher-Ming
W.,
Li-Ping
C.T.,
Boesenbergia rotunda: From Ethnomedicine to Drug Discovery. Evidence-Based Complementary and Alternative Medicine.
2012;
2012
:
473637
.
View Article PubMed Google Scholar -
Kim
S.,
H.I. Oh,
J.K. Hwang,
Oral administration of fingerroot (Boesenbergia pandurata) extract reduces ultraviolet b-induced skin aging in hairless mice. Food Science and Biotechnology.
2012;
21
:
1753-60
.
View Article Google Scholar -
Kazanci
A.,
M. Kurus,
A. Atasever,
Analyses of changes on skin by aging. Skin Research and Technology.
2017;
23
(1)
:
48-60
.
View Article Google Scholar -
Zhang
S.,
Duan
E.J.C.t.,
Fighting against skin aging: the way from bench to bedside. Cell transplantation.
2018;
27
(5)
:
729-38
.
View Article Google Scholar -
Gyöngyösi
N.,
K. Lőrincz,
A. Keszeg,
D. Haluszka,
A. Bánvölgyi,
E. Tátrai,
S. Kárpáti,
N.M. Wikonkál,
Photosensitivity of murine skin greatly depends on the genetic background: clinically relevant dose as a new measure to replace minimal erythema dose in mouse studies. Experimental dermatolog.
2016;
25
(7)
:
519-25
.
View Article Google Scholar -
C. Ji,
B. Yang,
Z. Yang,
Y. Tu,
Y.L. Yang,
L. He,
Z.G. Bi,
Ultra-violet B (UVB)-induced skin cell death occurs through a cyclophilin D intrinsic signaling pathway. Biochemical and Biophysical Research Communications.
2012;
425
(4)
:
825-9
.
View Article PubMed Google Scholar -
G. Imokawa,
Mechanism of UVB-induced wrinkling of the skin: paracrine cytokine linkage between keratinocytes and fibroblasts leading to the stimulation of elastase. Journal of Investigative Dermatology Symposium Proceedings.
2009;
14
(1)
:
36-43
.
View Article PubMed Google Scholar -
Choi
H. J.,
Alam
M. B.,
Baek
M. E.,
Kwon
Y. G.,
Lim
J. Y.,
Lee
S. H.,
Protection against UVB-induced photoaging by Nypa fruticans via inhibition of MAPK/AP-1/MMP-1 signaling. Oxidative Medicine and Cellular Longevity.
2020;
2020
:
2905362
.
View Article PubMed Google Scholar -
S. Listyawati,
S. Sismindari,
S. Mubarika,
Y.B. Murti,
Protective effect ethanolic extract of Boesenbergia pandurata (ROXB.) Schlecht. against UVB-induced DNA damages in Balb/c mice. Indonesian Journal of Pharmacy.
2015;
26
(2)
:
108
.
View Article Google Scholar
Comments
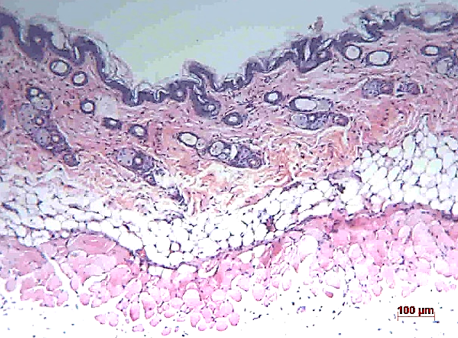
Downloads
Article Details
Volume & Issue : Vol 10 No 4 (2023)
Page No.: 5654-5665
Published on: 2023-04-30
Citations
Copyrights & License

This work is licensed under a Creative Commons Attribution 4.0 International License.
Search Panel
Pubmed
Google Scholar
Pubmed
Google Scholar
Pubmed
Google Scholar
Pubmed
Google Scholar
Pubmed
Search for this article in:
Google Scholar
Researchgate
- HTML viewed - 4142 times
- PDF downloaded - 1214 times
- XML downloaded - 146 times
- Supplement downloaded - 1120 times