Abstract
Introduction: Ensete glaucum seeds have been shown to possess antidiabetic properties. However, the specific hypoglycemic activities of the compounds they contain remain poorly understood. This study aimed to elucidate the hypoglycemic effects of E. glaucum seed compounds and to explore their mechanisms of action, particularly their interaction with pancreatic islets.
Methods: We employed spectroscopic techniques to determine the chemical structures of the isolated compounds, which we then compared with structures reported in the literature. In a streptozotocin (STZ)-induced diabetic mouse model, we evaluated the hypoglycemic response to an ethanol extract of E. glaucum seeds. Further, we assessed the islet-protective effects and the capacity to stimulate insulin release of both the crude extract and its individual compounds in STZ-challenged pancreatic islets.
Results: We isolated two novel compounds from E. glaucum seeds: afzelechin (a flavan-3-ol) and coniferaldehyde (a phenolic aldehyde), marking the first such report. Administration of the extract in doses of 25 and 50 mg/kg/day led to a significant reduction in blood glucose levels over a period of seven days in STZ-induced hyperglycemic mice. Notably, the extract and the isolated compounds afzelechin and coniferaldehyde exhibited a protective effect on islet β cells, mitigating STZ-induced cytotoxicity. Both the extract, at concentrations of 50 and 100 µg/mL, and the individual compounds, at 100 µM, demonstrated an ability to counteract STZ's inhibition of glucose-stimulated insulin secretion in isolated pancreatic islets. Importantly, molecular docking studies suggest that these compounds may stimulate insulin release from β cells by inhibiting the K_ATP channel, a hypothesis that warrants further experimental investigation.
Conclusion: Our findings suggest that E. glaucum seeds, along with their bioactive compounds, show promise in reducing blood sugar levels and enhancing insulin secretion, offering potential therapeutic avenues for diabetes management.
Introduction
Diabetes represents a significant global health issue, with its prevalence escalating rapidly. Type 2 diabetes, which accounts for the majority of cases, is marked by impaired pancreatic β-cell function and persistent insulin resistance. Pancreatic β cells are essential, as they regulate blood glucose by producing insulin. When these cells malfunction or die, insulin scarcity ensues, disrupting glucose homeostasis and causing blood glucose levels to rise1. It's therefore vital to maintain β cells' ability to secrete insulin. For treating diabetes, it's necessary to increase insulin levels to facilitate glucose uptake into cells, particularly for insulin-sensitive individuals, to decrease circulating blood glucose levels. Insulin and sulfonylurea treatments are typical examples of therapies that amplify this process. However, these treatments can have significant adverse effects. Insulin therapy can lead to various complications, including hypoglycemia, the Somogyi effect (a form of reactive hyperglycemia), insulin allergy, adipose tissue dystrophy, weight gain, and immune resistance to insulin2. Similarly, sulfonylureas may cause hypoglycemia, weight gain, and gastrointestinal issues, such as abdominal pain, nausea, and diarrhea. Particular caution is advised when prescribing these medications to patients with kidney failure, as drugs like glibenclamide and gliclazide are contraindicated in severe cases3. Hence, identifying substances that can protect and improve β cell functionality for more effective and safe insulin production and release is of paramount importance.
Plant-based sources are abundant in nutraceuticals and bioactive compounds with therapeutic potential. An estimated 80% of the world's population depends on herbal remedies for primary healthcare4. The application of medicinal plants and natural substances in diabetes treatment is garnering attention, supported by findings from preclinical and clinical research. For instance, a 2024 study demonstrated that a 70% ethanol extract of Ardisia elliptica Thunb., at a dosage of 250 mg/kg, was as effective in lowering serum glucose, creatinine, and urea levels as metformin, a leading antidiabetic drug5. A 2019 clinical trial found that consuming raspberries (Rubus idaeus) daily significantly decreased serum glucose levels after meals and reduced pro-inflammatory markers such as IL-6 and TNF-α four hours after consumption, compared to a control group6. Reports from 2023 have further underscored the importance of herbal medicines and plant secondary metabolites in diabetes management, particularly highlighting the elucidation of their action mechanisms7, 8, 9. Various plants, such as Momordica charantia L., Moringa oleifera Lam., Andrographis paniculata (Burm.f.) Nees, Clitoria ternatea L., and Gymnema sylvestre R.8, 10, 11, along with natural compounds like rutin, quercetin, hesperidin, kaempferol, cinnamic acid, resveratrol, paeoniflorin, and berberine7, 12, have been recognized for their antioxidant and hypoglycemic properties, as well as their role in enhancing the viability and insulin secretion of pancreatic β cells. The snow banana, scientifically designated as Ensete glaucum (Roxb.) Cheesman and a member of the Musaceae family13, is not just nutritionally rich in carbohydrates, fiber, vitamins, and minerals, but also in healing and health-promoting phytochemicals14. Traditionally, E. glaucum seeds have been extensively utilized by indigenous communities to treat a variety of ailments, including digestive disorders, diabetes, kidney stones, edema, stomach ulcers, and allergic reactions14, 15. Previous studies have indicated that E. glaucum seed extract can protect pancreatic cells in vivo and stimulate insulin secretion16; however, the responsible compounds and their mechanisms of action are yet to be identified.
Overall, this study aims to assess the hypoglycemic potential of E. glaucum seed extract and its isolated compounds in vivo, as well as their protective and stimulatory effects on insulin secretion from isolated mouse pancreatic islets in vitro, against streptozotocin toxicity, which is commonly used to induce diabetes by damaging pancreatic β cells. Moreover, this study anticipates determining the mechanism by which E. glaucum seed-derived compounds stimulate insulin, with a predictive in silico approach targeting the pancreatic ATP-sensitive potassium (K_ATP) channel.
Methods
Plant Material and Extraction Ensete glaucum bunches were harvested in Bac Ai District, Ninh Thuan Province, Viet Nam, in April 2020. An identification form (TNDL-CCD-2020) was kept at the Department of Natural Resources and Medicinal Materials Development, Research Center of Ginseng and Medicinal Materials in Ho Chi Minh City. The plant's scientific name has also been verified with the World Flora Online Plant List (https://wfoplantlist.org/plant-list). Seeds were separated from mature and ripe fruits and thoroughly rinsed with running water. After drying, the seeds were ground into a powder using a grinder. They were then extracted with 98% ethanol by hot extraction at 70°C for 60 minutes. The resultant liquid extract was filtered, and the extraction was repeated to achieve a weight/volume ratio of 1/20. The liquid extract was subsequently concentrated using a rotary evaporator at 70°C under reduced pressure to obtain the E. glaucum seed extract (EGE). The extract was preserved at 2-8°C and dissolved in an appropriate solvent to create a stock solution.Fractionation and Isolation The fractionation and isolation of compounds from E. glaucum seeds are summarized in Figure 1 and described in the Supplementary Information (SI).Animals The Institute of Vaccines and Medical Biologicals (IVAC) in Nha Trang City, Vietnam, supplied healthy Swiss albino mice (male, 4-5 weeks old, 18-22 g). The mice were cared for in an animal house with 50-60% relative humidity, a temperature of 25°C, and 12h light-dark cycles. They were acclimatized for 7 days before the experiment and housed in cages made of PP plastic. They had unlimited access to tap water and standard food supplied by IVAC. The administered volume (oral or injection) was 10 mL/kg body weight in the morning (8-9 a.m.).Experimental Design This study was a single-center, double-blind, controlled preclinical trial. The experiments were carried out at the Research Center of Ginseng and Medicinal Materials in Ho Chi Minh City. Streptozotocin (Sigma-Aldrich) (STZ, 170 mg/kg, i.p., single dose)17 was used after an overnight fast of 16 hours to induce a hyperglycemic mouse model. The streptozotocin was weighed and dissolved in 0.1 M sodium citrate pH 4.5 immediately before injection into mice. After 7 days, the fasting plasma glucose (FPG) was determined, and those with FPG ≥ 200 mg/dL18 were classified as hyperglycemic and chosen for the experiment.
The STZ-induced hyperglycemic mice were divided into 5 groups (n = 9). Group I received distilled water as the pathological control; Group II and III were administered EGE daily (25 and 50 mg/kg, respectively), and Group IV was administered glibenclamide daily (Medical Import Export Joint Stock Company DOMESCO, Vietnam) (GLI, 5 mg/kg). A group of normoglycemic mice injected with sodium citrate was also included in the study as a control. The animals were treated orally with/without EGE or glibenclamide for 7 days. The dosage and administration time of the extract and glibenclamide were selected based on previous reports16, 17. EGE or glibenclamide was mixed with distilled water for oral administration to the mice. The weight of the mice was measured daily.
Blood Biochemical Assessment
At baseline and at the end of day 7, mouse tail blood was collected to measure FPG concentration after one hour of treatment. Ten microliters of blood were combined with an equal volume of 2% EDTA (Ethylenediaminetetraacetic acid). The mixture was then centrifuged at 5,000 rpm for 5 minutes. Subsequently, 5 µL of plasma was gently mixed with 250 µL of glucose indicator agent at 37°C for 10 minutes. The glucose concentration was calculated according to the manufacturer’s guidelines for the GOD-PAP Kit (Glucose oxidase-phenol amino phenazone) from Erba, based on absorbance determined at 500 nm using a Biotek Microplate Reader (Synergy™ HTX Multi-Mode, USA)16.
Isolation and Culturing of Pancreatic Islets
Pancreatic islet isolation was performed in accordance with the previously described methods with some slight alterations19, 20. Mice were euthanized with carbon dioxide inhalation, and the pancreas was collected and treated with 3 mL of collagenase II (Gibco, Thermo Fisher Scientific, US) at 200 U/mL in sterile Hank's Balanced Salt Solution (HBSS, Gibco, Thermo Fisher Scientific, US) at 37°C for 30 minutes to achieve complete digestion. The islets were placed in a 50 mL falcon tube, rapidly cooled on ice, and then 25 mL of HBSS/CaCl2 at 3 mM was added with vigorous shaking for 10 seconds 40 times. The samples were centrifuged at 290 g for 30 seconds at 4°C. The precipitate was resuspended in 20 mL of HBSS/CaCl2 at 3 mM and filtered through a 0.419 mm sieve. Continuous centrifugation at 290 g for 30 seconds at 4°C was performed. The precipitate was then suspended in 10 mL of Ficoll 1077 and 10 mL of RPMI 1640 and centrifuged at 900 g for 20 minutes at 20°C. Pancreatic islets from the middle layer of Ficoll/medium were collected in RPMI 1640 containing 10% FBS, penicillin-streptomycin (100 U/mL-100 µg/mL), and centrifuged at 290 g for 5 minutes at 4°C. The pancreatic islets were filtered through a 70 nm filter into the culture medium. Individual islets, which had smooth perimeters and lacked a dark core, were selected using a 200-µL micropipette under a microscope and grown in a 6-well plate with fresh RPMI 1640 medium. After 24 hours, they were incubated at 37°C with 5% CO2. These islets were checked for their specificity and functionality.
Assessment of Islet Specificity and Functionality
Islet specificity was tested by Dithizone staining. Briefly, 10 µL of Dithizone (Sigma-Aldrich) solution (10 mg/mL) was added to 1 mL of Krebs-Ringer bicarbonate buffer (KRB, pH 7.4) containing suspended islets and incubated for 5-10 minutes at 37°C. Islets that stained bright red were observed under an inverted microscope (Nikon Eclipse Ts2R-FL, China), whereas non-islet tissues remained unstained19.
Islet functionality was assessed by measuring the level of insulin secreted. Isolated islets were washed and placed in KRB buffer. Then, 10 islets were exposed to 2.8 mM glucose in the buffer at 37°C for 60 minutes. Following this, the islets were stimulated with 16.8 mM glucose for another 60 minutes at 37°C. The insulin released into the medium under both conditions was determined using an ELISA insulin kit (Abcam) according to the manufacturer’s instructions21. The total cell protein content was also estimated using the Bradford reagent with BSA (Bovine Serum Albumin) as the standard for calculating insulin levels.
Assessment of Islet Mitochondrial Metabolism
An MTT (3-(4,5-Dimethylthiazol-2-yl)-2,5-diphenyltetrazolium bromide) assay (Sigma-Aldrich) was used to evaluate the effect of test samples on cell viability. Ten islets were treated with test samples at various concentrations at 37 °C in 5% CO2 for 24 hours. These islets were then collected and exposed to 100 μL of MTT (0.5 mg/mL) at 37 °C for 4 hours. Subsequently, 100 μL of dimethyl sulfoxide (Sigma-Aldrich) was added and the mixture was incubated for 15 minutes to solubilize the formazan crystals. Absorbance was recorded at 570 and 630 nm using a BioTek Microplate Reader. The viability of islet cells treated with and without test samples was presented as the percentage of cell viability (%) by comparing the mean absorbance of cells from different groups using the formula: Cell viability (%) = (Mean absorbance of treated cells – Mean absorbance of blank) / (Mean absorbance of untreated cells – Mean absorbance of blank) × 10022.
Experimental Design for Assessing Islet Protective Effects
Glucose-Stimulated Insulin Secretion (GSIS) Assay
Ten islets per group were treated for 1 hour at 37 °C in 500 μL KRB buffer containing either 2.8 or 16.8 mM glucose, along with either extracts or compounds from E. glaucum seeds at various concentrations23. Glimepiride (Stellapharm J.V. Co., Ltd.) was used as a positive control. Aliquots of supernatant were collected for insulin assays using an ELISA insulin kit (Abcam), following the manufacturer’s guidelines, and islets were gathered for protein assays using the Bradford reagent.
Effect of Extract and Compounds on Insulin Secretion in STZ-Treated Cells
The insulin-secreting effect of the test samples was evaluated in STZ-treated cells. Ten islets were treated with or without the extract (50 and 100 μg/mL) or compounds (50 and 100 μM) in the presence of STZ (5 mM)24. After 24 hours, the treated islets were incubated in KRB solution containing 16.8 mM glucose for 1 hour at 37 °C. Subsequently, the supernatant was collected, and the insulin content was estimated by an ELISA insulin kit (Mercodia), according to the manufacturer’s guidelines. The islets were then collected for protein assays using the Bradford reagent. Glimepiride served as a positive control. These experiments were repeated to confirm the protective effects of the extract and compounds from E. glaucum seeds on islet cells using the MTT assay.
Molecular Docking Simulation
The interaction between the compounds and the protein was evaluated through molecular docking. The 3D structures of the compounds were retrieved from PubChem25. The crystal structure of the recombinant pancreatic ATP-sensitive potassium channel (KATP channel), accession no. 6JB1, was obtained from the RCSB Protein Data Bank. The channel is a fusion construct of four inward-rectifier potassium channel 6 (Kir6.2) subunits from Mus musculus, surrounded by four sulfonylurea receptors 1 (SUR1) from Mesocricetus auratus (Figure S1-A in the Supplementary Information). The structure was processed with PyMOL 2.5 (https://pymol.org), to remove heteromolecules and reduce the size of the structure. Due to its symmetric nature, the KATP structure was trimmed to one SUR1 and two adjacent KIR6 subunits (Figure S1-B in the Supplementary Information), to lower the computational cost for docking. The trimmed structure and the compounds were processed as macromolecules and ligands using PyRx 1.9.2 with the default settings26. Docking was performed using the AutoDock Vina 1.2.0 protocol27 with the Vinardo scoring function, covering the entire protein with the grid, an exhaustiveness of 1000, and 15 output poses. The docking poses were evaluated based on the minimized affinity as calculated by the scoring function. The pose with the best affinity (lowest score) for each compound was visualized in PyMOL 2.5, and the protein-ligand interactions were analyzed using BIOVIA Discovery Studio Visualizer.
Statistical Analysis
Data were statistically analyzed using GraphPad Prism software (version 8.0.2, Inc., La Jolla, CA, USA) and reported as Mean ± SD. The data were initially tested for normality using the Anderson-Darling test and the Shapiro-Wilk test. One-way analysis of variance (ANOVA) was employed for statistical analysis, followed by Tukey’s test for homogeneity of variance and Dunnett’s test for heterogeneity of variance, assuming a normal distribution. Alternatively, the Kruskal-Wallis test was used in the case of a non-normal distribution, followed by Dunn’s post hoc test. Results were considered statistically significant when p < 0.05.
Position C/H | DEPT | 1H-NMR ( δ H ppm) (No. H, mult., J Hz) | 13 C-NMR ( δ C ppm) | HMBC correlations ( 1 H → 13 C) |
---|---|---|---|---|
2 | CH | 4.80 (1H, s ) | 78.0 | C-7, C-1’ |
3 | CH | 4.02 (1H, dd , 4.8, 9.6) | 64.8 | C-10, C-1’ |
4 | CH 2 | H a : 2.48 (1H, dd , 3.0, 16.2) H b : 2.68 (1H, dd , 4.8, 16.2) | 28.1 | C-2, C-3, C-5, C-6, C-8, C-9, C-10 |
5 | C | 156.5 | ||
6 | CH | 5.71 (1H, d , 2.4) | 94.1 | C-5, C-7, C-8, C-10 |
7 | C | 155.7 | ||
8 | CH | 5.89 (1H, d , 2.4) | 95.1 | C-6, C-7, C-9, C-10 |
9 | C | 156.2 | ||
10 | C | 98.4 | ||
1’ | C | 129.9 | ||
2’, 6’ | CH | 6.71 (2H, dd , 1.8, 6.6) | 114.4 | C-2, C-1’, C-3’, C-5’, C-4’ |
3’, 5’ | CH | 7.21 (2H, dd , 1.8, 7.2) | 128.2 | C-2, C-2’, C-6’, C-4’ |
4’ | C | 156.5 |
Position C/H | EG2 (acetone-d6) | Coniferaldehyde (acetone-d6s) | ||
1 H-NMR ( δ H ppm) (No. H, mult., J Hz) | 13 C-NMR ( δ C ppm) | 1 H-NMR ( δ H ppm) (No. H, mult., J Hz) | 13 C-NMR ( δ C ppm) | |
1 | 148.9 | 148.97 | ||
2 | 150.9 | 146.98 | ||
3 | 6.91 (1H, d , J=6.5 Hz) | 116.2 | 6.96 (1H, d , J=8.24 Hz) | 114.94 |
4 | 7.19 (1H, dd , J=1.5; 7.0 Hz) | 124.7 | 7.12 (1H, dd , J=2.14; 8.24 Hz) | 124.01 |
5 | 127.3 | 126.54 | ||
6 | 7.35 (1H, d , J=2.5 Hz) | 111.6 | 7.07 (1H, d , J=2.14 Hz) | 109.52 |
7 | 7.56 (1H, d , J=13.5 Hz) | 154.2 | 7.71 (1H, d , J=15.87 Hz) | 153.24 |
8 | 6.65 (1H, dd , J=1.5; 13.0 Hz) | 126.8 | 6.60 (1H, dd , J=7.62; 15.87 Hz) | 126.26 |
9 | 9.63 (1H, d , J=6.5 Hz) | 194.1 | 9.64 (1H, d , J=7.62 Hz) | 193.69 |
1-OH | 5.37 (H, s ) | 5.98 (H, s ) | ||
2-OCH3 | 3.91 (3H, s ) | 56.3 | 3.94 (3H, s ) | 55.93 |
Group | Plasma glucose level (mg/dL) | Body weight (g) | ||||
Basal | Pre-treatment | Post-treatment | Basal | Pre-treatment | Post-treatment | |
NC | 103.06 ± 14.27 | 106.83 ± 17.36*** | 116.69 ± 17.00 | 25.83 ± 0.95 | 28.57 ± 2.45 | 32.20 ± 3.36$, $$$$ |
HC | 96.08 ± 17.71 | 309.76 ± 93.62$$$ (↑189.96%) | 251.94 ± 97.47****, $$ (↑115.91%) | 25.10 ± 1.87 | 22.66 ± 5.03* | 21.90 ± 2.46#### |
H-EGE2 | 91.17 ± 15.44 | 306.32 ± 75.32@@@@ (↑186.74%) | 109.97 ± 14.72 (↓56.35%) | 25.77 ± 1.38 | 23.88 ± 4.54* | 26.26 ± 5.45 |
H-EGE5 | 97.14 ± 14.77 | 309.06 ± 79.08@@@@ (↑189.30%) | 92.63 ± 17.56 (↓63.23%) | 25.36 ± 1.63 | 23.66 ± 4.94* | 26.58 ± 5.48 |
H-GLI5 | 98.39 ± 13.11 | 300.96 ± 92.96@@@@ (↑181.72%) | 88.25 ± 22.12 (↓64.97%) | 25.63 ± 1.69 | 22.64 ± 3.83* | 23.19 ± 4.25 |
Results
Isolated compounds from E. glaucum seeds
The NMR (Nuclear Magnetic Resonance) data for compounds EG1 and EG2 were provided in Table 1 and Table 2, respectively, as well as in the Supplementary Information (SI). From these data, EG1 and EG2 were identified as afzelechin and coniferaldehyde, respectively, according to references28 for EG1 and29 for EG2. The structures of the compounds EG1 and EG2 are shown in the SI (Figure S2).
E. glaucum seed extract exhibited hypoglycemic potential in hyperglycemia
The hypoglycemic effect of E. glaucum seed extract on STZ-induced hyperglycemic mice after 7 days of treatment is shown in Table 3. The mean plasma glucose concentrations of mouse groups defined as pathological were higher than those of the physiological group (> 180%)). After 7 days of treatment, hyperglycemic mice treated with the extract (25 and 50 mg/kg) and glibenclamide (5 mg/kg) had notably reduced plasma glucose levels, while untreated hyperglycemic mice still had high plasma glucose levels (↑115.91% compared to the physiological group). Additionally, STZ not only increased plasma glucose levels but also negatively affected the weight of the mice. The extract at both doses showed a slight improvement in mouse weight after 7 days of treatment.
Extract and compounds of E. glaucum seeds stimulated insulin release from pancreatic islets
To determine whether the hypoglycemic effect of the ethanol extract of E. glaucum seeds was related to its ability to stimulate insulin secretion from β-cells, and whether compounds isolated from E. glaucum seeds contributed to this activity, pancreatic islets from mice were isolated. Islet specificity was assessed by dithizone staining, and β-cell insulin secretory function was examined by GSIS assay.
Dithizone staining is the standard method for islet characterization. Dithizone is a chemical compound that easily forms complexes with transition metals, and it can be used to identify cells that are abundant in zinc, such as islets, since zinc ions play a crucial role in preserving the structure and integrity of insulin molecules within β-cells and are released with insulin. Thus, dithizone is capable of differentiating islets from exocrine and ductal cells30. Under a microscope, cultured islets had smooth borders, uniform boundaries, and no darkening at the center (Figure 2 A, 2B, and 2C). Islets stained with dithizone showed that they were all crimson, with no presence of other cell types within the islets (Figure 2 D and 2E). Furthermore, insulin secretion slightly increased when islets were stimulated by a high glucose level (Figure 2 F), which was consistent with previously reported results4, 31.
To examine the effect of the ethanol extract and identified compounds from E. glaucum seeds on the enhancement of insulin release from islet β-cells with confidence, their effect on islet cell survival was evaluated and insulin levels were calculated based on the total cellular protein content. The findings indicated that there was no significant variance in the viability of islet cells when they were treated with the extract at concentrations from 12.5 to 50 µg/mL. However, the extract significantly increased the metabolic activity of the islets when the treatment dose rose to 100 and 500 µg/mL (Figure 3 A). Regarding the ability to stimulate insulin secretion, there was no difference in insulin levels between the extract-treated groups and the control in the basal state (2.8 mM glucose). Conversely, in the stimulated state (16.8 mM glucose), the extract at a concentration of 50 µg/mL significantly increased insulin secretion compared to the control (Figure 3 B).
Afzelechin, at the investigated concentrations (12.5-500 µM), did not exhibit a significant effect on the viability of islet cells compared with the control, although a 500 µM concentration slightly reduced islet cell survival compared to the control (Figure 3 C). The results showed no difference in insulin levels secreted from pancreatic islets treated with afzelechin (12.5-100 µM) compared to the control under basal conditions. However, there was a trend towards increased insulin secretion as the concentration gradually increased. Under stimulated conditions, 100 µM afzelechin significantly stimulated insulin-secreting islet β-cells compared to the control (Figure 3 D).
Similar to afzelechin, coniferaldehyde at concentrations between 12.5 and 100 µM did not affect islet cell survival; however, islet cell survival was significantly reduced when treated with this compound at a concentration of 500 µM (Figure 3 E). Coniferaldehyde (12.5-50 µM) also did not increase insulin release from isolated pancreatic islets under basal glucose conditions, while 100 µM coniferaldehyde stimulated insulin secretion under high glucose conditions (Figure 3 F).
Glimepiride, an insulin-stimulating sulfonylurea drug used to control glycemia in patients with type 2 diabetes, was used as the reference drug in this study. The results showed that glimepiride at a concentration of 10 µM did not affect islet cell survival. Simultaneously, it was able to stimulate insulin secretion by islet β-cells under both basal and stimulated states (Figure 3 G and 3H).
Extract and compounds of E. glaucum seeds protected isolated mouse islets against STZ toxicity
Based on the results of the GSIS test, to investigate the potential protective role of the extract, specifically afzelechin and coniferaldehyde, against STZ-induced decline in the insulinotropic action of glucose, isolated pancreatic islets were co-incubated with STZ (5 mM) and test sample at concentrations of 50 and 100 (µg/mL or µM), and then stimulated by a high dose of glucose (16.8 mM). STZ significantly reduced islet β-cell survival and insulin release compared to the control. Meanwhile, the extract at 50 and 100 µg/mL, and glimepiride at 10 µM, demonstrated islet cell protection with significantly higher cell survival compared to the STZ-treated group (Figure 4 A). Insulin release levels in the islet groups treated with the extract and glimepiride were also higher than in the STZ group, with a statistically significant difference found in the 100 µg/mL extract and 10 µM glimepiride-treated groups. Moreover, the potency of the extract appeared to be slightly lower than that of glimepiride (10 µM) (Figure 4 C).
To demonstrate whether afzelechin and coniferaldehyde had an effect on insulin release, playing a role in the activity of the extract, the experiment was repeated, showing that the effects of STZ were similar to those of the previous test, with a decrease in survival and secreted insulin levels compared to the control. Both compounds had the same islet protection against STZ toxicity as glimepiride, with a significant difference observed only in islets treated with the two compounds at a concentration of 100 µM (Figure 4 B). Similar results were found for the ability to stimulate insulin secretion from mouse isolated pancreatic islets. Afzelechin (100 µM), coniferaldehyde (100 µM), and glimepiride (10 µM) markedly improved insulin release compared with the STZ group. Notably, these compounds at a concentration of 100 µM appeared to be non-inferior to glimepiride at a concentration of 10 µM (Figure 4 D).
Afzelechin and coniferaldehyde stimulated insulin secretion from isolated islets by targeting the KATP channel
(+)-Afzelechin and coniferaldehyde, along with the control glimepiride, were docked to the trimmed structure of the KATP channel. The minimized affinity distribution of the resulting postures was represented in Figure 5 A. Overall, glimepiride exhibited the highest affinity to KATP (from -10.9 to -8.6 kcal/mol), followed by afzelechin (-8.3 to -7.2 kcal/mol) and coniferaldehyde (-7.3 to -5.8 kcal/mol). The majority of the poses of glimepiride bound to the space between the transmembrane domain 0 (TMD0) and TMD1 of the SUR1 subunit, while most poses of afzelechin and coniferaldehyde bound to the space between TMD1 and TMD2 of the SUR1 subunit (Figure S3 in the SI). However, the best pose of all three compounds bound to the same area between TMD1 and TMD2 of the SUR1 subunit, which is close to the bound position of the inhibitor repaglinide (Figure 5 B). Afzelechin had two alkyl interactions (W1297 and P551), a π-π stacked (W1297), a π-sigma (T548) interaction, and a hydrogen bond (R1145) (Figure 5 C). Coniferaldehyde interacted with R1300 and N547 through hydrogen bonds and with W1297 through a π-π stacked interaction (Figure 5 D). Meanwhile, glimepiride had more intensive interactions through hydrogen bonds (R1300, W1297, and Y1294) and alkyl interactions (V596, L434, L592, V587, L1149, P551, H584, and L1027).
Discussion
Medicinal plants are used around the world to support disease treatment and are potential sources of active medicinal ingredients7. Many medicinal plants and natural compounds derived from plants show promise in the treatment of diabetes; hence, they are also recommended to support diabetes treatment8, 11. In the present study, the antidiabetic effect of Ensete glaucum seeds was investigated.
In research, numerous diabetes models are employed, each with its own advantages and limitations, from in vitro models on β-cell lines and pancreatic islets to in vivo models with various animals such as fruit flies, roundworms, zebrafish, rodents (like mice), and large animals. Among these, the mouse model is the most commonly used32. Some agents that cause diabetic conditions can be chemicals (notably streptozotocin and alloxan), viruses, genetics, and surgery. Streptozotocin is the most frequently used chemical agent, with diverse doses and potential combinations with other agents such as nicotinamide and a high-fat diet to induce type 1 or type 2 diabetes33. Streptozotocin induces pancreatic β-cell toxicity, elevates blood glucose, reduces insulin secretion, and may trigger β-cell apoptosis through the induction of reactive oxygen species (ROS) both in vitro and in vivo34, 35. Experimental evidence has shown that STZ at a dose of 170 mg/kg increased mouse blood glucose17, a concentration of 5 mM could inhibit islet insulin secretion24, concentrations of 10-20 mM could induce β-cell apoptosis, while a dose of 30 mM induced β-cell necrosis36. In this study, STZ at 170 mg/kg and 5 mM was used to induce adverse effects on β cells in vivo and in vitro models, respectively. The current results provide evidence of a protective effect of the extract and compounds from E. glaucum seeds, including afzelechin and coniferaldehyde, against the deleterious effects of STZ on both the survival and glucose-stimulated insulin secretion from isolated pancreatic islets and glucose homeostasis in mice injected with a β-cytotoxic agent.
The existence of active chemical components in E. glaucum seeds contributed to the hypoglycemic effect and the enhancement of insulin release from islet β cells of the extract. Our previous screening investigation illustrated that seeds of E. glaucum contain polyphenols, flavonoids, tannins, and saponins15. Previous studies have shown that these phytochemicals have antioxidant, hypoglycemic, anti-inflammatory, β-cell preservation, and insulin-stimulating effects through various mechanisms12, 37, 38. In this study, the mouse isolated islet cell protective ability and insulin release stimulation from β cells of E. glaucum seed isolated compounds were further investigated. Afzelechin (a type of flavonoid) and coniferaldehyde (a type of phenolic) might be important components contributing to the effects of E. glaucum seeds detected in this study. Although some previous studies have shown the antioxidant39, glucose-metabolising40, anti-inflammatory41, and memory-improving42 activities of coniferaldehyde and the α-glucosidase inhibitory43, antioxidant44 and neuroprotective45 properties of afzelechin from plants, and Min6 β-cell insulin secreting potentiating activity of biosynthesized afzelechin from Escherichia coli bacteria46, this is the first study to show an effect of these compounds from E. glaucum seeds on islet protection and insulin secretion stimulation from pancreatic islets. Studying isolated pancreatic islets is more meaningful than studying a pancreatic β-cell line because pancreatic islets are a system of cell types with responsive interactions for homeostasis. From our potential findings, the active mechanism of these two compounds is on the K_ATP channel, an important channel in the regulation of insulin secretion from pancreatic β cells, which is also the target of sulfonylurea drugs commonly used in the treatment of type 2 diabetes.
The K_ATP channel is an octameric complex formed by four inward-rectifier K+ channels 6 (Kir6) as the pore-forming unit, surrounded by four sulfonylurea receptors (SUR) as the regulatory unit. There are several types of K_ATP channels assembled from different combinations of Kir6.2 and SUR, including the pancreatic type (SUR1/Kir6.2), cardiac type (SUR2A/Kir6.2), and the vascular smooth muscle type (SUR2B/Kir6.1). The K_ATP channel of pancreatic β-cells is a well-known target for medications for type 2 diabetes, including repaglinide and sulfonylureas. Several structures of pancreatic K_ATP are available, including from humans47, 48 and mice/rats49, 50, some of which are drug-bound conformations. These structures contribute insight into the inhibitive mechanisms of antidiabetic drugs. The crystal structure of the drug-bound K_ATP channel, including repaglinide and glibenclamide, has been resolved49, 50, revealing the inhibition mechanism. The drugs bind to the groove between TMD1 and TMD2 of SUR1, which prevents the dimerization of its two nucleotide binding domains (NBDs) and modulates the interaction between SUR1 and the N-terminus of KIR6, both events can inhibit channel opening (Figure 6). Residues in SUR1, including R1246 and R1300, are essential for the interaction and inhibitory effects of repaglinide and glibenclamide on K_ATP49, 50.
Docking analysis indicates that the three compounds afzelechin, coniferaldehyde, and glimepiride also bind to the same groove as repaglinide and glibenclamide. This result is unsurprising for glimepiride since it is also a sulfonylurea like glibenclamide. Glimepiride also interacts with R1300, W1297, and L592, similarly to glibenclamide. Together with the highest binding affinity, these results can reasonably explain its high activity in insulin release tests. Afzelechin and coniferaldehyde might have similar or partial inhibitory mechanisms to those of repaglinide and glibenclamide due to the similarity in their binding sites. Coniferaldehyde has a lower affinity compared to afzelechin; however, it was shown to also interact with R1300, one of the key residues. These results could expound on the similar activity of afzelechin and coniferaldehyde in the tests.
In this study, the hypoglycemic effect of E. glaucum seed extract was demonstrated in STZ-induced hyperglycemic mice. From the results of this study and our previous research16, the active mechanism behind this activity may be the effect of stimulating pancreatic β cells to secrete insulin in response to hyperglycemic states. Additionally, other effects, such as protecting pancreatic cells against apoptosis and inhibiting glucose absorption, were observed. Simultaneously, one of the compounds that may contribute to the effects of the extract is afzelechin and coniferaldehyde with the predicted mechanism of inhibiting the K_ATP channel, thereby stimulating insulin secretion from β cells.
The physicochemical and ADME (Absorption-Distribution-Metabolism-Excretion) properties of coniferaldehyde and afzelechin were analyzed in our previous study using computational assays16. These properties are within an acceptable range for oral medications. Afzelechin has a poor predicted blood-brain barrier penetration, which did not affect its effectiveness in this case since the targeted K_ATP channel is located on pancreatic β-cells. Our other data suggested that afzelechin and coniferaldehyde exhibit an inhibitory effect against protein tyrosine phosphatase 1B (PTP1B) and α-glucosidase16, which are also potential targets for the treatment of type 2 diabetes. These properties highlight the potential of these two compounds as medications for type 2 diabetes.
The potential biological effects of afzelechin and coniferaldehyde have been mentioned in previous studies and this study. However, their safety and adverse effects are unclear. A prediction of the toxicity of these two compounds in previous research indicated that the oral LD_50 of afzelechin and coniferaldehyde were 2500 mg/kg (Category 5 by GHS—Globally Harmonized System of Classification and Labeling of Chemicals) and 1560 mg/kg (Category 4 by GHS)16, respectively. In comparison, metformin and glimepiride, drugs used to treat diabetes in clinical practice, have an LD_50 value of 1000 mg/kg (Category 4 by GHS) and >10000 mg/kg (Category 5 by GHS), respectively. Therefore, the LD_50 of afzelechin and coniferaldehyde are within the acceptable range. Afzelechin was not predicted to trigger any of the five toxicities examined (hepatotoxicity, carcinogenicity, immunotoxicity, mutagenicity, cytotoxicity), whereas coniferaldehyde was predicted to potentially have hepatotoxicity and immunotoxicity16. The toxicity risk of coniferaldehyde was also shown in in vitro testing, which, at a concentration of 500 µg/mL, could be toxic to pancreatic islet cell survival, with a significantly lower percentage of viable cells compared to the control; this may be due to the presence of its aldehyde functional group. Thus, in vivo safety studies of these compounds should be performed.
Although the E. glaucum seed extract was demonstrated to have an antidiabetic effect, several isolated compounds, including afzelechin and coniferaldehyde, could potentially contribute to the effect of the extract by stimulating pancreatic β-cells to secrete insulin. Nevertheless, there are still some limitations that exist in this study. Firstly, the mechanism by which the extract and compounds stimulate pancreatic β-cells to secrete insulin has not been clarified; it is necessary to further study this effect through in vitro and in vivo experiments at the molecular level. Secondly, afzelechin and coniferaldehyde from E. glaucum seeds are potential compounds that inhibit the K_ATP channel according to in silico testing, but their inhibitory effects on this channel have not been demonstrated by in vitro or in vivo testing, which is beyond the scope of our report. Thirdly, the hypoglycemic effect of afzelechin and coniferaldehyde from E. glaucum seeds has not been proven in an STZ-induced hyperglycemic mouse model due to the limited number of isolated samples. It is encouraged to perform such testing in the future to demonstrate the in vivo effects of these compounds. Fourthly, the safety of the extract (sub-acute toxicity) and the compounds (acute and sub-acute toxicity) in animals was not evaluated in this study. In conclusion, our results also showed the potential effect of E. glaucum seeds, as well as some of its components, in supporting the treatment of diabetes by reducing blood glucose, possibly by stimulating pancreatic β-cells to secrete insulin.
Conclusions
E. glaucum seed extract exhibited a hypoglycemic effect in an STZ-induced hyperglycemic mouse model. The extract stimulated pancreatic β-cells to secrete insulin and protected pancreatic islet cells from the damaging toxicity of STZ. Afzelechin and coniferaldehyde, isolated from E. glaucum seeds, also displayed protective activity and stimulated insulin release from mouse pancreatic islet β-cells through the predicted mechanism of K_ATP channel inhibition. The findings of this study have partially confirmed the potential of E. glaucum seeds for further future studies aimed at supporting diabetes management.
Abbreviations
ADME - Absorption, Distribution, Metabolism, and Excretion, DEPT - Distortionless Enhancement by Polarization Transfer, EDTA - Ethylenediaminetetraacetic Acid, ELISA - Enzyme-Linked Immunosorbent Assay, FBS - Fetal Bovine Serum, FPG - Fasting Plasma Glucose, GHS - Globally Harmonized System of Classification and Labeling of Chemicals, GOD-PAP - Glucose Oxidase-Phenol Amino Phenazone, GSIS - Glucose-Stimulated Insulin Secretion, HBSS - Hank's Balanced Salt Solution, K_ATP - ATP-sensitive Potassium Channel, KRB - Krebs-Ringer Bicarbonate Buffer, LD_50 - Lethal Dose, 50%, MTT - 3-(4,5-Dimethylthiazol-2-yl)-2,5-diphenyltetrazolium Bromide, NBD - Nucleotide Binding Domain, NMR - Nuclear Magnetic Resonance, PTP1B - Protein Tyrosine Phosphatase 1BSTZ - Streptozotocin, SUR - Sulfonylurea Receptor, TMD - Transmembrane Domain, µg/mL - Micrograms per Milliliter, µM - Micromolar, Kir6 - Inward-rectifier K+ Channels 6
Acknowledgments
We express our sincere gratitude to MSc. Le Duc Thanh, the Research Center of Ginseng and Medicinal Materials Ho Chi Minh City, for timely authentication of herbs under study.
Author’s contributions
LHT conceptualized, designed, conducted the experiments, analyzed the data, and wrote the paper; LPH performed the in silico experiments and collaborated in the preparation of the manuscript; LTKA contributed to the in vitro experiments; LBT performed chemical composition isolation; NMK, NTTH contributed ideas, edited and reviewed the manuscript; LVM supervised the work, edited and reviewed the manuscript. All the authors have read the final manuscript and approved the submission.
Funding
This work received partially financial support from the Department of Science and Technology of Ninh Thuan Province, Viet Nam (Grant number 11/2020/HĐ-SKHCN).
Availability of data and materials
All the data generated during this study were statistically analyzed and are presented in the figures and tables. The dataset could be available upon reasonable request from the corresponding author.
Ethics approval
All the animal studies were carried out according to the guidelines of the “Guidelines for the Care and Use of Laboratory Animals” (NIH publication #85-23, revised in 1985) and this research work and protocols were approved by the institutional animal ethical committee of University of Medicine and Pharmacy at Ho Chi Minh City, Ho Chi Minh City, Viet Nam (Ref: 591/GCN-HĐĐĐNCTĐV).
Consent for publication
Not applicable.
Competing interests
The authors declare that they have no competing interests.
References
-
ElSayed
N.A.,
Aleppo
G.,
Aroda
V.R.,
Bannuru
R.R.,
Brown
F.M.,
Bruemmer
D.,
on behalf of the American Diabetes Association
2. Classification and Diagnosis of Diabetes: Standards of Care in Diabetes-2023. Diabetes Care.
2023;
46
:
19-40
.
View Article PubMed Google Scholar -
Thota
S.,
Akbar
A.,
Insulin. In: StatPearls [Internet]. Treasure Island (FL): StatPearls Publishing. 2023. Available from: https://www.ncbi.nlm.nih.gov/books/NBK560688/. .
.
-
Costello
R.A.,
Nicolas
S.,
Shivkumar
A.,
Sulfonylureas. In: StatPearls [Internet]. Treasure Island (FL): StatPearls Publishing. 2023. Available from: https://www.ncbi.nlm.nih.gov/books/NBK513225/. .
.
-
Rahimifard
M.,
Navaei-Nigjeh
M.,
Mahroui
N.,
Mirzaei
S.,
Siahpoosh
Z.,
D
P.,
Improvement in The Function of Isolated Rat Pancreatic Islets through Reduction of Oxidative Stress Using Traditional Iranian Medicine. Cell Journal.
2014;
16
(2)
:
147-63
.
PubMed Google Scholar -
Wong
P.L.,
Zolkeflee
N.K.,
Ramli
N.S.,
Tan
C.P.,
Azlan
A.,
Tham
C.L.,
Antidiabetic effect of Ardisia elliptica extract and its mechanisms of action in STZ-NA-induced diabetic rat model via 1H-NMR-based metabolomics. Journal of Ethnopharmacology.
2024;
318
.
View Article PubMed Google Scholar -
Schell
J.,
Betts
N.M.,
Lyons
T.J.,
Basu
A.,
Raspberries Improve Postprandial Glucose and Acute and Chronic Inflammation in Adults with Type 2 Diabetes. Annals of Nutrition & Metabolism.
2019;
74
(2)
:
165-74
.
View Article PubMed Google Scholar -
Wang
T.,
Wang
Y.Y.,
Shi
M.Y.,
Liu
L.,
Mechanisms of action of natural products on type 2 diabetes. World Journal of Diabetes.
2023;
14
(11)
:
1603-20
.
View Article PubMed Google Scholar -
Ahda
M.,
Jaswir
I.,
Khatib
A.,
Ahmed
Q.U.,
Mahfudh
N.,
Ardini
Y.D.,
A review on selected herbal plants as alternative anti-diabetes drugs: chemical compositions, mechanisms of action, and clinical study. International Journal of Food Properties.
2023;
26
(1)
:
1414-25
.
View Article Google Scholar -
Yedjou
C.G.,
Grigsby
J.,
Mbemi
A.,
Nelson
D.,
Mildort
B.,
Latinwo
L.,
The Management of Diabetes Mellitus Using Medicinal Plants and Vitamins. International Journal of Molecular Sciences.
2023;
24
(10)
:
9085
.
View Article PubMed Google Scholar -
Wickramasinghe
A.S.,
Kalansuriya
P.,
Attanayake
A.P.,
Herbal Medicines Targeting the Improved β-Cell Functions and β-Cell Regeneration for the Management of Diabetes Mellitus. Evidence-Based Complementary and Alternative Medicine.
2021;
2021
.
View Article PubMed Google Scholar -
Rahman
M.M.,
Uddin
M.J.,
Reza
A.S.,
Tareq
A.M.,
Emran
T.B.,
Simal-Gandara
J.,
Ethnomedicinal Value of Antidiabetic Plants in Bangladesh: A Comprehensive Review. Plants.
2021;
10
(4)
:
729
.
View Article PubMed Google Scholar -
Shehadeh
M.B.,
Suaifan
G.A.,
Abu-Odeh
A.M.,
Plants Secondary Metabolites as Blood Glucose-Lowering Molecules. Molecules (Basel, Switzerland).
2021;
26
(14)
:
4333
.
View Article PubMed Google Scholar -
Cheesman
E.E.,
Classification of the Bananas: The Genus Ensete Horan. Kew Bulletin.
1947;
2
(2)
:
97-106
.
View Article Google Scholar -
Joga
R.,
Sangma
E.,
Karmakar
B.,
Lyngdoh
V.,
Aochen
C.,
Phytochemical investigations on the therapeutic properties of Ensete glaucum (Roxb.) Cheesman. Indian Journal of Traditional Knowledge.
2020;
20
:
68-73
.
-
Ly
H.T.,
Khuong
H.,
Do
T.,
Nguyen
T.,
Phan
T.,
Extraction and Phytochemical Composition of Ensete glaucum (Roxb.) Cheesman Fruits in Bu Gia Map National Park, Binh Phuoc Province. Journal of Technical Education Science..
2022;
116-20
:
116-20
.
View Article Google Scholar -
Ly
H.T.,
Pham
K.D.,
Le
P.H.,
Do
T.H.,
Nguyen
T.T.,
Le
V.M.,
Pharmacological properties of Ensete glaucum seed extract: novel insights for antidiabetic effects via modulation of oxidative stress, inflammation, apoptosis and MAPK signaling pathways. Journal of Ethnopharmacology.
2024;
320
.
View Article PubMed Google Scholar -
Ly
H.T.,
Nguyen
T.T.,
Tran
T.T.,
Lam
B.T.,
Phung
T.T.,
Le
V.M.,
Hypoglycemic and antioxidant activities of Clerodendrum inerme leaf extract on streptozotocin-induced diabetic models in mice. Chinese Herbal Medicines.
2019;
11
(4)
:
387-93
.
View Article Google Scholar -
Hammeso
W.W.,
Emiru
Y.K.,
Ayalew Getahun
K.,
Kahaliw
W.,
Antidiabetic and Antihyperlipidemic Activities of the Leaf Latex Extract of Aloe megalacantha Baker (Aloaceae) in Streptozotocin-Induced Diabetic Model. Evidence-Based Complementary and Alternative Medicine.
2019;
2019
.
View Article PubMed Google Scholar -
Saliba
Y.,
Bakhos
J.J.,
Itani
T.,
Farès
N.,
An optimized protocol for purification of functional islets of Langerhans. Laboratory Investigation.
2017;
97
(1)
:
70-83
.
View Article PubMed Google Scholar -
Zmuda
E.J.,
Powell
C.A.,
Hai
T.,
A method for murine islet isolation and subcapsular kidney transplantation. Journal of Visualized Experiments.
2011;
2096
(50)
.
View Article PubMed Google Scholar -
Banerjee
M.,
Bhonde
R.R.,
Islet generation from intra islet precursor cells of diabetic pancreas: in vitro studies depicting in vivo differentiation. JOP.
2003;
4
(4)
:
137-45
.
PubMed Google Scholar -
Kumar
P.,
Nagarajan
A.,
Uchil
P.D.,
Analysis of Cell Viability by the MTT Assay. Cold Spring Harb Protoc. 2018;2018(6):pdb.prot095505. Available from: https://doi.org/10.1101/pdb.prot095505..
.
-
Rahimifard
M.,
Baeeri
M.,
Bahadar
H.,
Moini-Nodeh
S.,
Khalid
M.,
Haghi-Aminjan
H.,
Therapeutic Effects of Gallic Acid in Regulating Senescence and Diabetes; an In Vitro Study. Molecules (Basel, Switzerland).
2020;
25
(24)
:
5875
.
View Article PubMed Google Scholar -
Samaddar
S.,
Jha
D.,
Koneri
R.,
Optimization of pancreatic islet isolation from rat and evaluation of islet protective potential of a saponin isolated from fruits of Momordica dioica. Journal of Applied Pharmaceutical Science.
2020;
10
:
89-099
.
View Article Google Scholar -
Kim
S.,
Chen
J.,
Cheng
T.,
Gindulyte
A.,
He
J.,
He
S.,
PubChem 2023 update. Nucleic Acids Research.
2023;
51
:
1373-80
.
View Article PubMed Google Scholar -
Dallakyan
S.,
Olson
A.J.,
Small-Molecule Library Screening by Docking with PyRx. 2015;
:
243-50
.
View Article Google Scholar -
Eberhardt
J.,
Santos-Martins
D.,
Tillack
A.F.,
Forli
S.,
AutoDock Vina 1.2.0: New Docking Methods, Expanded Force Field, and Python Bindings. Journal of Chemical Information and Modeling.
2021;
61
(8)
:
3891-8
.
View Article PubMed Google Scholar -
Wan
S.B.,
Chan
T.H.,
Enantioselective synthesis of afzelechin and epiafzelechin. Tetrahedron.
2004;
60
(37)
:
8207-11
.
View Article Google Scholar -
Sy
L.K.,
Brown
G.D.,
Coniferaldehyde derivatives from tissue culture of Artemisia annua and Tanacetum parthenium. Phytochemistry.
1999;
50
(5)
:
781-5
.
View Article Google Scholar -
Khiatah
B.,
Qi
M.,
Wu
Y.,
Chen
K.T.,
Perez
R.,
Valiente
L.,
Pancreatic human islets and insulin-producing cells derived from embryonic stem cells are rapidly identified by a newly developed Dithizone. Scientific Reports.
2019;
9
(1)
:
9295
.
View Article PubMed Google Scholar -
Karaoz
E.,
Genç
Z.S.,
Demircan
P.Ç.,
Aksoy
A.,
Duruksu
G.,
Protection of rat pancreatic islet function and viability by coculture with rat bone marrow-derived mesenchymal stem cells. Cell Death & Disease.
2010;
1
(4)
.
View Article PubMed Google Scholar -
Kleinert
M.,
Clemmensen
C.,
Hofmann
S.M.,
Moore
M.C.,
Renner
S.,
Woods
S.C.,
Animal models of obesity and diabetes mellitus. Nature Reviews. Endocrinology.
2018;
14
(3)
:
140-62
.
View Article PubMed Google Scholar -
Arivazhahan
A.,
Screening Methods for the Evaluation of Antidiabetic Drugs. In: Lakshmanan M, Shewade DG, Raj GM, editors. Introduction to Basics of Pharmacology and Toxicology: Volume 3: Experimental Pharmacology: Research Methodology and Biostatistics. Singapore: Springer Nature Singapore; 2022. p. 453-63..
.
-
Furman
B.L.,
Streptozotocin-Induced Diabetic Models in Mice and Rats. Current Protocols.
2021;
1
(4)
.
View Article PubMed Google Scholar -
Yuan
J.,
Li
S.,
Peng
H.,
Ma
Y.,
Li
L.,
Fu
L.,
Artesunate protects pancreatic β-cells from streptozotocin-induced diabetes via inhibition of the NLRP3/caspase-1/GSDMD pathway. General and Comparative Endocrinology.
2022;
326
.
View Article PubMed Google Scholar -
Saini
K.S.,
Thompson
C.,
Winterford
C.M.,
Walker
N.I.,
Cameron
D.P.,
Streptozotocin at low doses induces apoptosis and at high doses causes necrosis in a murine pancreatic beta cell line, INS-1. Biochemistry and Molecular Biology International.
1996;
39
(6)
:
1229-36
.
View Article PubMed Google Scholar -
Ghorbani
A.,
Rashidi
R.,
Shafiee-Nick
R.,
Flavonoids for preserving pancreatic beta cell survival and function: A mechanistic review. Biomedicine and Pharmacotherapy.
2019;
111
:
947-57
.
View Article PubMed Google Scholar -
Sun
C.,
Zhao
C.,
Guven
E.C.,
Paoli
P.,
Simal-Gandara
J.,
Ramkumar
K.M.,
Dietary polyphenols as antidiabetic agents. Food Frontiers.
2020;
1
(1)
:
18-44
.
View Article Google Scholar -
Cai
D.,
Wang
J.,
Chen
S.,
Jiang
L.,
Chen
J.,
Wu
J.,
Coniferaldehyde prevents articular cartilage destruction in a murine model via Nrf2/HO% UNKNOWN UNICODE CHARACTER 02011 (NON-BREAKING HYPHEN) 1 pathway. Molecular Medicine Reports.
2021;
23
(3)
:
224
.
View Article PubMed Google Scholar -
Gai
H.,
Zhou
F.,
Zhang
Y.,
Ai
J.,
Zhan
J.,
You
Y.,
Coniferaldehyde ameliorates the lipid and glucose metabolism in palmitic acid-induced HepG2 cells via the LKB1/AMPK signaling pathway. Journal of Food Science.
2020;
85
(11)
:
4050-60
.
View Article PubMed Google Scholar -
Itoh
T.,
Ando
M.,
Tsukamasa
Y.,
Wakimoto
T.,
Nukaya
H.,
Whiskey congeners suppress LPS/IFNγ-induced NO production in murine macrophage RAW 264 cells by inducing heme oxygenase-1 expression. Journal of Agricultural and Food Chemistry.
2012;
60
(51)
:
12491-500
.
View Article PubMed Google Scholar -
Dong
Y.,
Stewart
T.,
Bai
L.,
Li
X.,
Xu
T.,
Iliff
J.,
Coniferaldehyde attenuates Alzheimer's pathology via activation of Nrf2 and its targets. Theranostics.
2020;
10
(1)
:
179-200
.
View Article PubMed Google Scholar -
Saijyo
J.,
Suzuki
Y.,
Okuno
Y.,
Yamaki
H.,
Suzuki
T.,
Miyazawa
M.,
Alpha-glucosidase inhibitor from Bergenia ligulata. Journal of Oleo Science.
2008;
57
(8)
:
431-5
.
View Article PubMed Google Scholar -
Li
D.L.,
Li
X.M.,
Peng
Z.Y.,
Wang
B.G.,
Flavanol derivatives from Rhizophora stylosa and their DPPH radical scavenging activity. Molecules (Basel, Switzerland).
2007;
12
(5)
:
1163-9
.
View Article PubMed Google Scholar -
Li
G.,
Min
B.S.,
Zheng
C.,
Lee
J.,
Oh
S.R.,
Ahn
K.S.,
Neuroprotective and free radical scavenging activities of phenolic compounds from Hovenia dulcis. Archives of Pharmacal Research.
2005;
28
(7)
:
804-9
.
View Article PubMed Google Scholar -
Chemler
J.A.,
Lock
L.T.,
Koffas
M.A.,
Tzanakakis
E.S.,
Standardized biosynthesis of flavan-3-ols with effects on pancreatic beta-cell insulin secretion. Applied Microbiology and Biotechnology.
2007;
77
(4)
:
797-807
.
View Article PubMed Google Scholar -
Zhao
C.,
MacKinnon
R.,
Molecular structure of an open human KATP channel. Proceedings of the National Academy of Sciences of the United States of America.
2021;
118
(48)
.
View Article PubMed Google Scholar -
Lee
K.P.,
Chen
J.,
MacKinnon
R.,
Molecular structure of human KATP in complex with ATP and ADP. eLife.
2017;
6
.
View Article PubMed Google Scholar -
Martin
G.M.,
Kandasamy
B.,
DiMaio
F.,
Yoshioka
C.,
Shyng
S.L.,
Anti-diabetic drug binding site in a mammalian KATP channel revealed by Cryo-EM. eLife.
2017;
6
.
View Article PubMed Google Scholar -
Ding
D.,
Wang
M.,
Wu
J.X.,
Kang
Y.,
Chen
L.,
The Structural Basis for the Binding of Repaglinide to the Pancreatic KATP Channel. Cell Reports.
2019;
27
(6)
.
View Article PubMed Google Scholar
Comments
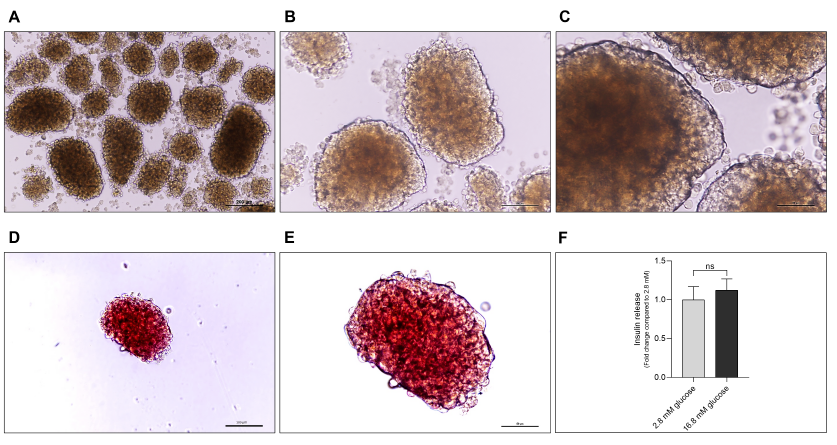
Downloads
Article Details
Volume & Issue : Vol 11 No 4 (2024)
Page No.: 6363-6378
Published on: 2024-04-30
Citations
Copyrights & License

This work is licensed under a Creative Commons Attribution 4.0 International License.
Search Panel
Pubmed
Google Scholar
Pubmed
Google Scholar
Pubmed
Google Scholar
Pubmed
Google Scholar
Pubmed
Google Scholar
Pubmed
Google Scholar
Pubmed
Search for this article in:
Google Scholar
Researchgate
- HTML viewed - 1811 times
- XML downloaded - 79 times
- PDF downloaded - 496 times
- Supplement downloaded - 419 times