Abstract
Introduction: The linkage between specific genetic variants and the susceptibility to dental caries remains a crucial area of investigation. This study aimed to examine the association of particular gene variants within MPPED2 (rs12797813), MTR (rs61739582), ACTN2 (rs6656267), and LPO (rs7209537) genes with the likelihood of developing dental caries.
Methods: The study recruited a cohort of 600 participants, divided equally between 300 individuals diagnosed with dental caries and 300 healthy controls devoid of the condition. The focus was on the genotyping of the mentioned gene variants using the Tetra-Primer Amplification Refractory Mutation System PCR (Tetra-ARMS-PCR) technique. This method is noted for its efficacy in detecting genetic variations.
Results: Analysis revealed significant associations between the occurrence of dental caries and the SNPs rs6656267 and rs7209537, with p-values less than 0.05 indicating statistical relevance. Notably, the presence of the C allele of rs6656267 and the A allele of rs7209537 was disproportionately higher amongst individuals with dental caries compared to the healthy control group. These associations were evident across both co-dominant and dominant genetic models, suggesting that these variants may increase the risk of developing dental caries. The discrepancy with the allele reference suggests a potential error in the citation of rs8178275, which likely should be rs7209537 based on the context provided.
Conclusion: The findings of this study suggest that the rs6656267 and rs7209537 variants in the ACTN2 and LPO genes, respectively, may serve as potential genetic markers for increased susceptibility to dental caries. However, the complex nature of genetic influences on dental caries risk necessitates further research to confirm these initial findings and to explore the mechanisms by which these SNPs influence caries development.
Introduction
Dental caries (DC), commonly known as tooth decay, is a chronic multifactorial condition that affects a large proportion of the population worldwide1. This condition arises from the interplay between oral bacteria, dietary carbohydrates, and various host factors, including saliva composition, tooth structure, and immune responses1. While behavioral and environmental factors play a crucial role in the development of DC, genetic influences have also been recognized as contributing to individual susceptibility2. Numerous genetic variations have been identified as potential contributors to a person's predisposition to developing DC. Among the genes studied in this context are metallophosphoesterase domain-containing 2 (MPPED2), methionine synthetase (MTR), alpha-actinin-2 (ACTN2), and lactoperoxidase (LPO). The MPPED2 gene, located on chromosome 11 (11p14.1), codes for a metallophosphoesterase. Although the precise function of this gene is not yet fully understood, it appears to be involved in the development of the nervous system3 and has been associated with aniridia 1, a congenital eye disorder4. Interestingly, while the MPPED2 gene does not have a known direct role in DC, a study has shown that its expression was significantly reduced (by a factor of 5) in oral epithelial cell lines exposed to bacterial pathogens. This finding suggests a potential indirect link between the MPPED2 gene and susceptibility to tooth decay5.
The enzymes methionine synthase reductase (MTRR) and MTR play a crucial role in the metabolic pathways involving folic acid, homocysteine, and vitamin B126. Previous studies have reported a relationship between vitamin B12 levels and the onset of DC7. Furthermore, cases with reduced concentrations of folic acid have been found to be more prone to caries development8. These findings collectively illustrate the prominent role of MTRR and MTR in the context of DC. It is known that gene polymorphisms can mediate the expression of MTRR and MTR, suggesting the potential involvement of MTRR and MTR gene polymorphisms in susceptibility to DC9.
The ACTN2 gene is responsible for the production of the alpha-actinin-2 protein, a cytoskeletal protein involved in the organization and stabilization of actin filaments in muscle cells10. Interestingly, ACTN2 is also expressed in odontoblasts, the cells that synthesize and secrete dentin, which constitutes the majority of the tooth structure beneath the enamel11. ACTN2 polymorphisms have been linked to various muscle-related disorders, such as hypertrophic cardiomyopathy and skeletal muscle myopathies12, 13. However, the role of ACTN2 in susceptibility to DC is not well-established, and the mechanisms by which ACTN2 polymorphisms might affect tooth structure or function are not fully understood14. It has been hypothesized that variants within ACTN2 may affect the binding affinity of ACTN2 to actin filaments or other proteins involved in dentin formation, thereby altering tooth structure and increasing the risk of DC14. Nevertheless, the available evidence on the association between ACTN2 gene polymorphisms and DC susceptibility is limited and conflicting, with some studies reporting positive associations and others finding no significant effects15, 16.
The LPO gene codes for a bactericidal salivary enzyme that plays a crucial role in protecting the lactating mammary gland and the intestinal tract of newborn infants against pathogenic microorganisms17, 18. In addition to its antimicrobial function, LPO has been reported to have other functions, such as growth-promotion activity and anti-tumor activity19, 20. Interestingly, a genome-wide association study (GWAS) focused on DC in children aged 3 to 12 years, examining their primary dentition, identified several novel genes, including LPO21. However, it is important to note that GWAS is a hypothesis-generating method, and the results require careful scrutiny and replication in independent samples to distinguish chance results from true associations.
To further elucidate the potential role of the MPPED2, MTR, ACTN2, and LPO genes in susceptibility to DC, additional studies are needed to investigate their functional effects on tooth development and to replicate the associations of their polymorphisms in different populations22. Such investigations could provide valuable insights into the genetic and molecular mechanisms underlying DC, which could inform the development of targeted prevention and treatment strategies for this common oral disease. In the present study, we aimed to investigate the potential association of specific single nucleotide polymorphisms (SNPs) within the MPPED2 (rs12797813), MTR (rs61739582), ACTN2 (rs6656267), and LPO (rs7209537) genes with susceptibility to DC in a population-based sample. Our findings could offer important insights into the genetic basis of DC and contribute to the development of personalized prevention and treatment approaches for this widespread oral health condition.
Gene (SNP) | Primers | Sequence (3'-5') | Product size |
ACTN2 (rs114880747) | Forward inner primer (G allele) | AGGTTTGCTATTTGTAAAAAATTTCATGTG | For G allele: 191 |
Reverse inner primer (A allele) | GGGGCAATCACATAAGCATATTAGATAT | For A allele: 258 | |
Forward outer primer (5' - 3') | GCGCTTCATAAATAGGTTTATTTCTGAG | Two outer primers: 391 | |
Reverse outer primer (5' - 3') | CCAAAAATCTTTTGGGTAGTCTTTTTAA | ||
LPO (rs8178275) | Forward inner primer (G allele) | GCTATTGCATCAACCAATCCCTGACG | For G allele: 229 |
Reverse inner primer (A allele) | GGCAGATACACCAGGAAACTGCAGCAT | For A allele: 164 | |
Forward outer primer (5' - 3') | TTAAAAGGGACAAATGTGCTCAGGGCAT | Two outer primers: 340 | |
Reverse outer primer (5' - 3') | TCTTACCTGCCCAGTGCCTTGTCTTTTC |
Gene (SNP) | Genotype in DMFT Score n = 300 | Decayed Missing Filled Teeth Score (DMFT score) | p-value | ||||||
1 DMFT 190 (63.35%) | 2 DMFT 65 (21.6%) | 3 DMFT 19 (6.3%) | 4 DMFT 14 (4.6%) | 5 DMFT 7 (2.33%) | 6 DMFT 3 (1%) | 7 DMFT 2 (0.66%) | |||
ACTN2 (rs114880747) | AA | 12 | 10 | 2 | - | - | - | - | ˂ 0.05 |
AG | 65 | 40 | 15 | 10 | 4 | 2 | 1 | ||
GG | 113 | 15 | 2 | 4 | 3 | 1 | 1 | ||
LPO (rs8178275) | AA | 20 | 19 | 5 | 3 | 3 | 1 | 1 | ˂ 0.05 |
AG | 49 | 29 | 12 | 9 | 2 | 2 | 1 | ||
GG | 121 | 17 | 2 | 2 | 2 | - | - |
Methods
Subjects
We carefully calculated the necessary sample size for the investigation using the statistical formula: n = Z²p(1-p)/e², where the value of Z was set at 1.96, and the estimated frequency was 60%. Based on these parameters, the researchers determined that the study would need to include 300 participants. To ensure a robust comparison, the study design incorporated a control group consisting of age-matched individuals who did not have dental caries (DC). Individuals who did not consent to participate were excluded from the study. In total, the final study sample comprised 600 individuals - 300 participants diagnosed with DC and 300 healthy controls who shared similar demographic characteristics. A dentist conducted a comprehensive oral examination of all the study participants. The DC (cavities) experience in the participants' teeth was assessed using the dmfs index, which measures the number of tooth surfaces that are decayed, missing due to decay, or filled/restored. Importantly, the study was conducted in full compliance with the ethical principles outlined in the Declaration of Helsinki. Each participant provided written informed consent, and 5 ml of peripheral blood was collected in EDTA-containing tubes for further analysis as part of the research protocol.
SNPs Genotyping
The genetic samples used in this study were obtained by extracting genomic DNA from EDTA-treated blood samples through the standard salting-out method. The extracted DNA was then stored at -20°C until the genetic analysis was conducted. To genotype the specified SNPs (rs12797813, rs61739582, rs6656267, and rs7209537), the tetra-primer amplification refractory mutation system PCR (Tetra-ARMS-PCR) approach was employed. The primer design for this method was facilitated by the Primer 1 online tool available at http://primer1.soton.ac.uk/primer1.html. The accuracy of the genotyping process was ensured by including negative control samples without genomic DNA and positive controls with known genotypes, which were compared to the corresponding sequencing results. The thermal cycling conditions for the PCR amplification of the individual SNPs were as follows:
- MPPED2 SNP rs12797813: Initial denaturation at 95°C for 5 minutes, followed by 30 cycles of denaturation at 95°C for 30 seconds, annealing at 62.5°C for 30 seconds, and extension at 72°C for 2 minutes, with a final extension step at 72°C for 5 minutes.
- MTR SNP rs61739582: Initial denaturation at 95°C for 5 minutes, followed by 30 cycles of denaturation at 95°C for 30 seconds, annealing at 63°C for 30 seconds, and extension at 72°C for 2 minutes, with a final extension step at 72°C for 5 minutes.
- ACTN2 SNP rs6656267: Initial denaturation at 95°C for 5 minutes, followed by 30 cycles of denaturation at 95°C for 30 seconds, annealing at 64.5°C for 30 seconds, and extension at 72°C for 2 minutes, with a final extension at 72°C for 5 minutes.
- LPO SNP rs7209537: Initial denaturation at 95°C for 5 minutes, followed by 30 cycles of denaturation at 95°C for 30 seconds, annealing at 61.5°C for 30 seconds, and extension at 72°C for 2 minutes, with a final extension at 72°C for 5 minutes.
For each PCR reaction, the following components were used: 1 µl of each inner primer (10 PM), 1 µl of each outer primer (5 PM), 1 µl of Mastermix (Amplicon® Mastermix containing MgCl2, Taq PCR buffer, Taq DNA polymerase, and dNTPs), and 2 µl of DNA (50 ng/µl), adjusted to a total volume of 20 µl with ddH2O. The primer sequences are provided in Table 1.
Statistical Analysis
We utilized the SNPStats web tool (https://www.snpstats.net/start.htm) to evaluate the selected SNP's conformity to Hardy-Weinberg equilibrium (HWE) and to investigate the associations between SNPs and DC using various models (co-dominant, dominant, recessive, and over-dominant). The impact of each variant was quantified using an odds ratio (OR) accompanied by its respective 95% confidence interval, while statistical significance was determined by a p-value of 0.05 or lower.
Patients | Healthy controls | P-value | ||
rs114880747 | A/A | 24 | 19 | ˃ 0.05 |
A/G | 137 | 120 | ||
G/G | 139 | 161 | ||
rs8178275 | A/A | 52 | 30 | ˃ 0.05 |
A/G | 104 | 124 | ||
G/G | 144 | 146 |
Model | DC patients Number (%) | Controls Number (%) | OR (95% CI) | P-value | |||
rs114880747 | Allele | A vs. G | 185 (30.8%) 415 (69.2%) | 158 (26.3%) 442 (73.7%) | 0.596 (0.432-0.811) | 0.005 | |
Co-dominant | A/A | 24 (8%) | 19 (6.3%) | 1.00 | 0.022 | ||
A/G | 137 (45.7%) | 120 (40%) | 0.58 (0.38-0.86) | ||||
G/G | 139 (46.3%) | 161 (53.7%) | 0.50 (0.23-1.08) | ||||
Dominant | A/A | 24 (8%) | 19 (6.3%) | 1.00 | 0.011 | ||
A/G-G/G | 276 (92%) | 281 (93.7%) | 0.55 (0.37-0.81) | ||||
Recessive | A/A-A/G | 161 (53.7%) | 139 (46.3%) | 1.00 | 0.16 | ||
G/G | 139 (46.3%) | 161 (53.7%) | 0.59 (0.28-1.26) | ||||
Overdominant | A/A-G/G | 163 (54.3%) | 180 (60%) | 1.00 | 0.059 | ||
A/G | 137 (45.7%) | 120 (40%) | 0.72 (0.51-1.01) | ||||
rs8178275 | Allele | A vs. G | 208 (34.7%) 392(65.3%) | 184 (30.7%) 416(69.3%) | 0.543 (0.411-0.809) | 0.013 | |
Co-dominant | A/A | 52 (17.3%) | 30 (10%) | 1.00 | 0.014 | ||
A/G | 104 (34.7%) | 124 (41.3%) | 1.71 (1.02-2.86) | ||||
G/G | 144 (48%) | 146 (48.7%) | 1.00 | 0.035 | |||
Dominant | A/A | 52 (17.3%) | 30 (10%) | 1.00 | |||
A/G-G/G | 248 (82.7%) | 270 (90%) | 2.06 (1.08-4.38) | ||||
Recessive | A/A-A/G | 156 (52) | 154 (51.3%) | 1.00 | 0.12 | ||
G/G | 144 (48%) | 146 (48.7%) | 1.87 (0.76-4.62) | ||||
Overdominant | A/A-G/G | 196 (65.3%) | 176 (58.7%) | 1.00 | 0.09 | ||
A/G | 104 (34.7%) | 124 (41.3%) | 1.45 (0.91-2.30) |
Results
Our study enrolled 300 patients with DC and 300 healthy individuals, with the patients having a mean age of 17 years and the controls having a mean age of 20.5 years. The case group consisted of 98 males (32.7%) and 202 females (67.3%), while the control group comprised 115 males (38.3%) and 185 females (61.7%). Table 2 presents the responses to questionnaires that children and their parents completed. The severity of DC was assessed by calculating the DMFT score, which quantified the presence of decayed, missing, and filled teeth for each individual participant. We found an association between the rs6656267 SNP in the ACTN2 gene and the DMFT score in DC cases (p = 0.025), as presented in Table 2. Additionally, our findings, shown in Table 3, demonstrate an association between the rs7209537 SNP in the LPO gene and the DMFT score in DC cases (p = 0.001).
In accordance with the Hardy-Weinberg equilibrium, the genotype distributions of the analyzed SNPs were consistent with expectations (p > 0.05). The results of the exact tests conducted for the various SNPs are as follows: for rs12797813, the p-values were 0.10 for cases and 0.17 for controls; for rs61739582, the p-values were 0.08 for cases and 0.13 for controls; for rs6656267, the p-values were 0.11 for cases and 0.18 for controls; and for rs7209537, the p-values were 0.08 for cases and 0.16 for controls, as presented in Table 4. The DNA band positions on the agarose gel are depicted in Figure 1.
A statistically significant increase in the frequency of the C allele (minor allele) for rs6656267 was observed in individuals with DC compared to healthy controls (OR (95% CI) = 0.596 (0.432-0.811), p = 0.013; as shown in Table 4). This SNP was associated with the risk of DC in both co-dominant and dominant models (OR (95% CI) = 0.58 (0.38-0.86), p-value = 0.03; OR (95% CI) = 0.55 (0.37-0.81), p-value = 0.029, respectively). Additionally, the frequency of the A allele (minor allele) for rs7209537 was found to be significantly higher in individuals with DC compared to healthy controls, with an odds ratio (95% confidence interval) of 0.543 (0.411-0.809) and a p-value of 0.045. These particular SNPs were linked to an increased risk of DC in both co-dominant and dominant genetic models, with odds ratios (95% confidence intervals) of 1.71 (1.02-2.86) and 2.06 (1.08-4.38) and p-values of 0.014 and 0.019, respectively. It is also worth mentioning that there was no association between rs12797813 and rs61739582 and susceptibility to DC in any of the models (p-value > 0.05).
Discussion
Dental caries (DC) is influenced by both external environmental factors and genetic predisposition23. High-sugar diets, particularly those rich in candies and chocolates, are a common cause of DC24. Additionally, inadequate oral hygiene practices, insufficient consumption of fluoride, and prolonged bottle-feeding are other contributing factors to the onset of DC25. Implementing preventive measures early on can help mitigate the occurrence of DC at an early stage. Numerous studies have explored the genetic predisposition to tooth decay, and various genes have been identified that may contribute to the development of this condition26, 27, 28, 29. The genetic susceptibility to tooth decay involves multiple genes and environmental factors. Identifying the genes involved in the development of tooth decay could provide valuable insights into the etiology of this condition and pave the way for the creation of novel preventive and therapeutic approaches. The current study aimed to enhance the understanding of the genetic foundation of caries susceptibility. To achieve this, samples were collected from a population in Iran, both with and without DC, and genotyped for specific polymorphisms (rs12797813 SNP of the MPPED2 gene, SNP rs61739582 of the MTR gene, rs6656267 SNP of the ACTN2 gene, and rs7209537 SNP of the LPO gene). These genes were identified as candidate genes in previous genome-wide association studies (GWAS) for DC15, 16. The current study found a positive association between the rs6656267 and rs7209537 polymorphisms and susceptibility to DC. This observation aligns with the findings from a previous study conducted on a cohort of 65 Greek children aged 5 to 12 years, which showed a positive association between the ACTN2 (rs6656267) gene polymorphism and DC16. Furthermore, another GWAS has provided significant evidence of an association between ACTN2 single nucleotide polymorphisms (SNPs) and DC15. While the precise mechanism linking ACTN2 to DC remains incompletely understood, one possible explanation is that the ACTN2 gene appears to be involved in regulating and organizing ameloblasts, the cells responsible for tooth enamel formation30, 31. The ACTN2 gene is located on chromosome 1 (1q43) and encodes a protein that is a member of the spectrin gene superfamily and is involved in the formation of the cytoskeleton. ACTN2 has been associated with several disorders, including cardiomyopathy30, 31. The LPO protein plays a crucial role in defending against DC in the context of oral health32. It also helps regulate the microbial composition in the oral cavity, preventing the growth of pathogenic microorganisms associated with periodontitis33. The physiological properties of LPO have been exploited in the development of oral hygiene products enriched with the LPO protein to prevent these oral diseases34. Salivary LPO has the ability to convert thiocyanate ions (SCN-) and hydrogen peroxide into hypothiocyanite ions (OSCN-). These OSCN- ions possess the capacity to kill bacteria by binding to their proteins. As a result, the presence of LPO in saliva promotes oral health and helps prevent the development of periodontitis35. The available research indicates that the concentration of LPO in the saliva is higher in patients with periodontitis compared to healthy individuals36, 37, 38. As early as 1995, the LPO concentration was proposed as a potential marker to evaluate the degree of periodontal tissue destruction39. Subsequently, later studies have shifted their focus to evaluating the product of LPO activity, malondialdehyde (MDA), which is a lipid peroxidation biomarker40. Dakovic's study found a relationship between the concentration of MDA in the saliva and the degree of inflammation in the periodontium, concluding that the levels of this marker in saliva indicate the presence of inflammatory activity at that particular time41. Other researchers have corroborated these findings42, 43, and the most recent study by Veljovic et al. in 2022 obtained statistically significant results demonstrating a positive association between periodontitis and the levels of MDA and LPO in both blood and saliva44. In contrast, the current study has found that the rs7209537 SNP of the LPO gene is significantly associated with susceptibility to DC. This observation is not in alignment with the findings of Stanley et al., who, through a meta-analysis, reported that SNPs within the LPO gene are not associated with DC susceptibility15. These discrepancies in results may be attributable to genetic differences between the study populations. Given the diverse functions of ACTN2 and LPO genes, it is plausible that complex interactions between them could modulate an individual's risk of developing DC. Exploring these potential gene-gene interactions may provide valuable insights into the multifactorial nature of DC susceptibility.
It is also worth mentioning that our results have shown that there is no association of polymorphisms within the MTR (rs61739582) and MPPED2 (rs12797813) genes with DC in studied participants. The MTR gene, responsible for methionine and homocysteine production, has not been previously implicated in DC, although maternal MTR may be associated with non-syndromic cleft lip and palate45, which in turn is associated with DC46, 47, 48. The findings from the current study are notable in the context of previous research. Unlike our study population, Weilian Du et al.49 have shown that the risk of DC is associated with the MTR gene rs1805087 polymorphism in Chinese children. Notably, Antunes et al.50 reported that the MTRR gene rs1801394 polymorphism was identified as a genetic risk factor for early childhood caries (ECC) susceptibility in Rio de Janeiro, while the MTR gene rs1805087 polymorphism was not associated with ECC. This is consistent with the results of the present study. The reason for this discrepancy is not entirely clear, but the researchers believe that the MTR gene rs1805087 polymorphism is worthy of further examination. Additionally, in line with the current study, Katifelis et al.16 have shown that polymorphisms within the MPPED2 gene are not associated with the risk of DC. However, another study has revealed that MPPED2 polymorphisms are significantly associated with childhood caries. These mixed findings highlight the complexity of the genetic factors underlying susceptibility to oral diseases, such as DC. The discrepancies between studies may be attributable to factors like differences in study populations, methodologies, and genetic backgrounds.
DC is a multifactorial disease, and the interplay between various genetic factors may significantly influence an individual's risk of developing the condition. By focusing solely on the selected SNPs, our study may have overlooked the potential synergistic or antagonistic effects of genetic variants across different genes. The findings from our study, which identified significant associations between the rs6656267 and rs7209537 variants and susceptibility to DC, have the potential to contribute to the evolving field of personalized dentistry and public health interventions. The identification of genetic risk factors for DC can have significant public health implications. DC is a highly prevalent and chronic oral health condition, presenting a substantial burden on healthcare systems globally. By understanding the genetic underpinnings of DC susceptibility, we can explore strategies for early identification, targeted prevention, and personalized management of this condition. In addition, the incorporation of genetic screening for DC risk factors into routine dental care practices could provide valuable insights for both clinicians and patients. By assessing an individual's genetic profile, healthcare providers can gain a better understanding of their predisposition to developing DC. This information can then be used to tailor preventive strategies, optimize oral hygiene regimens, and monitor high-risk individuals more closely. Building on the genetic risk profiles obtained through screening, healthcare providers can develop personalized preventive measures and treatment approaches for patients.
Genetic variations can differ significantly among populations due to factors like ancestry, geographical location, and ethnic diversity. These population-specific genetic differences can lead to discrepancies in the results of association studies conducted across diverse populations. It is crucial to account for these population-specific genetic factors when interpreting the findings of such studies. Furthermore, the extensive review of the relevant literature conducted as part of this study makes a significant contribution to the field of DC research. This comprehensive review of existing knowledge provides a valuable foundation for understanding the complex genetic underpinnings of this widespread oral health condition. Continued research is necessary to fully elucidate the genetic determinants of oral health conditions and their potential utility as diagnostic or prognostic markers.
Conclusions
The present study investigated the potential association between specific genetic variants within the MPPED2 (rs12797813), MTR (rs61739582), ACTN2 (rs6656267), and LPO (rs7209537) genes and susceptibility to DC. Using the reliable T-ARMS-PCR method for genotyping, the researchers found a significant association between the rs6656267 single nucleotide polymorphism (SNP) in the ACTN2 gene and the rs7209537 SNP in the LPO gene with an increased risk of developing DC. These findings suggest that the rs6656267 variant of the ACTN2 gene and the rs7209537 variant of the LPO gene may serve as potential genetic risk factors for DC. The observed associations were noted in both the co-dominant and dominant genetic models, further strengthening the relevance of these gene variants in DC susceptibility. While the study provides valuable insights into the potential role of the rs6656267 and rs7209537 variants in DC, the researchers acknowledge the need for additional investigations to confirm and expand upon these findings. Further studies with larger sample sizes, diverse population cohorts, and functional analyses are warranted to validate the associations and elucidate the underlying mechanisms by which these gene variants influence DC susceptibility. Unraveling the genetic basis of DC can have significant implications for disease prevention, early detection, and personalized treatment approaches. By understanding the genetic determinants of DC, researchers and clinicians can potentially develop targeted interventions and strategies to mitigate the impact of this prevalent oral health condition. In conclusion, the present study presents evidence supporting the association of the rs6656267 variant within the ACTN2 gene and the rs7209537 variant within the LPO gene with increased susceptibility to DC. These findings contribute to the growing body of knowledge regarding the genetic factors underlying this widespread oral disease and highlight the importance of continued research in this field. In future studies, it will be crucial to investigate the interactions between the ACTN2, LPO, MPPED2, and MTR genes, as well as other relevant genes, while performing functional studies to better understand the complex genetic landscape underlying DC susceptibility.
Abbreviations
ACTN2 - Alpha-actinin-2, CI - Confidence Interval, DC - Dental Caries, DMFT - Decayed, Missing, and Filled Teeth, ECC - Early Childhood Caries, EDTA - Ethylenediaminetetraacetic acid, GWAS - Genome-Wide Association Study, HWE - Hardy-Weinberg Equilibrium, LPO - Lactoperoxidase, MDA - Malondialdehyde, MPPED2 - Metallophosphoesterase domain-containing 2, MTR - Methionine synthase, MTRR - Methionine Synthase Reductase, OR - Odds Ratio, PCR - Polymerase Chain Reaction, SNPs - Single Nucleotide Polymorphisms, Tetra-ARMS-PCR - Tetra-Primer Amplification Refractory Mutation System PCR
Acknowledgments
The authors would like to announce their appreciation to all patients who have made the achievement of current investigation. This research was conducted with the support of Mashhad University of Medical Sciences.
Author’s contributions
SA: designed the research study and performed the research, SD: wrote the manuscript and performed the research, YN: wrote the manuscript and performed the research, SK: wrote the manuscript and performed the data analysis, AF: wrote the manuscript and collaboration in data analysis, MRA: performed the research, BB: performed the research, FA: designed the research study and performed the research and data analysis. All authors read and approved the final manuscript.
Funding
This research was conducted with the support of Mashhad University of Medical Sciences.
Availability of data and materials
Data and materials used and/or analyzed during the current study are available from the corresponding author on reasonable request.
Ethics approval and consent to participate
The study was conducted in adherence to the ethical principles outlined in the Declaration of Helsinki and approved by ethical committee of Mashhad University of Medical Sciences.
Consent for publication
Not applicable.
Competing interests
The authors declare that they have no competing interests.
References
-
Pitts
N.B.,
Twetman
S.,
Fisher
J.,
Marsh
P.D.,
Understanding dental caries as a non-communicable disease. British Dental Journal.
2021;
231
(12)
:
749-53
.
View Article PubMed Google Scholar -
Cavallari
T.,
Arima
L.Y.,
Ferrasa
A.,
Moysés
S.J.,
Tetu Moysés
S.,
Hirochi Herai
R.,
Dental caries: genetic and protein interactions. Archives of Oral Biology.
2019;
108
.
View Article PubMed Google Scholar -
GeneCards. Human Gene Database, MPPED2 gene. . https://wwwgenecardsorg/cgi-bin/carddisppl?gene=MPPED2 Accessed May 25, 2024..
.
-
MalaCards. Human Disease Database. Aniridia 1 (AN1). . https://wwwmalacardsorg/card/aniridia_1 Accessed May 25, 2024..
.
-
Milward
M.R.,
Chapple
I.L.,
Wright
H.J.,
Millard
J.L.,
Matthews
J.B.,
Cooper
P.R.,
Differential activation of NF-kappaB and gene expression in oral epithelial cells by periodontal pathogens. Clinical and Experimental Immunology.
2007;
148
(2)
:
307-24
.
View Article PubMed Google Scholar -
Elizabeth
K.E.,
Praveen
S.L.,
Preethi
N.R.,
Jissa
V.T.,
Pillai
M.R.,
Folate, vitamin B12, homocysteine and polymorphisms in folate metabolizing genes in children with congenital heart disease and their mothers. European Journal of Clinical Nutrition.
2017;
71
(12)
:
1437-41
.
View Article PubMed Google Scholar -
M Hugar
S.,
S Dhariwal
N.,
Majeed
A.,
Badakar
C.,
Gokhale
N.,
Mistry
L.,
Assessment of vitamin B12 and its correlation with dental caries and gingival diseases in 10-to 14-year-old children: a cross-sectional study. International Journal of Clinical Pediatric Dentistry.
2017;
10
(2)
:
142-6
.
View Article PubMed Google Scholar -
MacKeown
J.M.,
Cleaton-Jones
P.E.,
Fatti
P.,
Caries and micronutrient intake among urban South African children: a cohort study. Community Dentistry and Oral Epidemiology.
2003;
31
(3)
:
213-20
.
View Article PubMed Google Scholar -
He
L.,
Van Roie
E.,
Bogaerts
A.,
Verschueren
S.,
Delecluse
C.,
Morse
C.I.,
The genetic effect on muscular changes in an older population: a follow-up study after one-year cessation of structured training. Genes.
2020;
11
(9)
:
968
.
View Article PubMed Google Scholar -
Ranta-Aho
J.,
Olive
M.,
Vandroux
M.,
Roticiani
G.,
Dominguez
C.,
Johari
M.,
Mutation update for the ACTN2 gene. Human Mutation.
2022;
43
(12)
:
1745-56
.
View Article PubMed Google Scholar -
Khan
Q.E.,
Sehic
A.,
Khuu
C.,
Risnes
S.,
Osmundsen
H.,
Expression of Clu and Tgfb1 during murine tooth development: effects of in-vivo transfection with anti-miR-214. European Journal of Oral Sciences.
2013;
121
(4)
:
303-12
.
View Article PubMed Google Scholar -
Chiu
C.,
Bagnall
R.D.,
Ingles
J.,
Yeates
L.,
Kennerson
M.,
Donald
J.A.,
Mutations in alpha-actinin-2 cause hypertrophic cardiomyopathy: a genome-wide analysis. Journal of the American College of Cardiology.
2010;
55
(11)
:
1127-35
.
View Article PubMed Google Scholar -
Savarese
M.,
Palmio
J.,
Poza
J.J.,
Weinberg
J.,
Olive
M.,
Cobo
A.M.,
Actininopathy: A new muscular dystrophy caused by ACTN2 dominant mutations. Annals of Neurology.
2019;
85
(6)
:
899-906
.
View Article PubMed Google Scholar -
https://www.ncbi.nlm.nih.gov/snp/rs114880747. .
.
-
Stanley
B.O.,
Feingold
E.,
Cooper
M.,
Vanyukov
M.M.,
Maher
B.S.,
Slayton
R.L.,
Genetic association of MPPED2 and ACTN2 with dental caries. Journal of Dental Research.
2014;
93
(7)
:
626-32
.
View Article PubMed Google Scholar -
Katifelis
H.,
Sioziou
A.,
Gazouli
M.,
Emmanouil
D.,
ACTN2 (rs6656267) and MPPED2 (rs11031093 and rs536007) polymorphisms in primary dentition caries: A case-control study. International Journal of Paediatric Dentistry.
2020;
30
(4)
:
478-82
.
View Article PubMed Google Scholar -
Gothefors
L.,
Marklund
S.,
Lactoperoxidase activity in human milk and in saliva of newborn infants. Infection and Immunity.
1975;
11
(6)
:
1210-5
.
View Article PubMed Google Scholar -
Leigh
J.A.,
Field
T.R.,
Williams
M.R.,
Two strains of Streptococcus uberis, of differing ability to cause clinical mastitis, differ in their ability to resist some host defence factors. Research in Veterinary Science.
1990;
49
(1)
:
85-7
.
View Article PubMed Google Scholar -
Reiter
B.,
Fulford
R.J.,
Marshall
V.M.,
Yarrow
N.,
Ducker
M.,
Knutsson
M.,
An evaluation of the growth promoting effect of the lactoperoxidase system in newborn calves. Animal Science (Penicuik, Scotland).
1981;
32
(3)
:
297-306
.
View Article Google Scholar -
Stanislawski
M.,
Rousseau
V.,
Goavec
M.,
Ito
H.,
Immunotoxins containing glucose oxidase and lactoperoxidase with tumoricidal properties: in vitro killing effectiveness in a mouse plasmacytoma cell model. Cancer Research.
1989;
49
(20)
:
5497-504
.
PubMed Google Scholar -
Shaffer
J.R.,
Wang
X.,
Feingold
E.,
Lee
M.,
Begum
F.,
Weeks
D.E.,
Genome-wide association scan for childhood caries implicates novel genes. Journal of Dental Research.
2011;
90
(12)
:
1457-62
.
View Article PubMed Google Scholar -
Cordell
H.J.,
Clayton
D.G.,
Genetic association studies. Lancet.
2005;
366
(9491)
:
1121-31
.
View Article PubMed Google Scholar -
Elamin
A.,
Garemo
M.,
Gardner
A.,
Dental caries and their association with socioeconomic characteristics, oral hygiene practices and eating habits among preschool children in Abu Dhabi, United Arab Emirates - the NOPLAS project. BMC Oral Health.
2018;
18
(1)
:
104
.
View Article PubMed Google Scholar -
Skafida
V.,
Chambers
S.,
Positive association between sugar consumption and dental decay prevalence independent of oral hygiene in pre-school children: a longitudinal prospective study. Journal of Public Health (Oxford, England).
2018;
40
(3)
:
e275-83
.
View Article PubMed Google Scholar -
Selwitz
R.H.,
Ismail
A.I.,
Pitts
N.B.,
Dental caries. Lancet.
2007;
369
(9555)
:
51-9
.
View Article PubMed Google Scholar -
Opal
S.,
Garg
S.,
Jain
J.,
Walia
I.,
Genetic factors affecting dental caries risk. Australian Dental Journal.
2015;
60
(1)
:
2-11
.
View Article PubMed Google Scholar -
Werneck
R.I.,
Mira
M.T.,
Trevilatto
P.C.,
A critical review: an overview of genetic influence on dental caries. Oral Diseases.
2010;
16
(7)
:
613-23
.
View Article PubMed Google Scholar -
Wang
X.,
Willing
M.C.,
Marazita
M.L.,
Wendell
S.,
Warren
J.J.,
Broffitt
B.,
Genetic and environmental factors associated with dental caries in children: the Iowa Fluoride Study. Caries Research.
2012;
46
(3)
:
177-84
.
View Article PubMed Google Scholar -
Li
Z.Q.,
Hu
X.P.,
Zhou
J.Y.,
Xie
X.D.,
Zhang
J.M.,
Genetic polymorphisms in the carbonic anhydrase VI gene and dental caries susceptibility. Genetics and Molecular Research.
2015;
14
(2)
:
5986-93
.
View Article PubMed Google Scholar -
GeneCards. Human Gene Database Aghwgaoc-bcipgAAO, 2019.. 2019
.
-
Sehic
A.,
Risnes
S.,
Khan
Q.E.,
Khuu
C.,
Osmundsen
H.,
Gene expression and dental enamel structure in developing mouse incisor. European Journal of Oral Sciences.
2010;
118
(2)
:
118-30
.
View Article PubMed Google Scholar -
Gudipaneni
R.K.,
Kumar R
V.,
G
J.,
Peddengatagari
S.,
Duddu
Y.,
Short term comparative evaluation of antimicrobial efficacy of tooth paste containing lactoferrin, lysozyme, lactoperoxidase in children with severe early childhood caries: a clinical study. Journal of Clinical and Diagnostic Research : JCDR.
2014;
8
(4)
:
18-20
.
View Article PubMed Google Scholar -
Shimizu
E.,
Kobayashi
T.,
Wakabayashi
H.,
Yamauchi
K.,
Iwatsuki
K.,
Yoshie
H.,
Effects of orally administered lactoferrin and lactoperoxidase-containing tablets on clinical and bacteriological profiles in chronic periodontitis patients. International journal of dentistry.
2011;
2011
:
405139
.
View Article PubMed Google Scholar -
Borzouee
F.,
Mofid
M.R.,
Varshosaz
J.,
Samsam Shariat
S.Z.,
Purification of lactoperoxidase from bovine whey and investigation of kinetic parameters. Advanced Biomedical Research.
2016;
5
(1)
:
189
.
View Article PubMed Google Scholar -
Magacz
M.,
K\kedziora
K.,
Sapa
J.,
Krzyściak
W.,
The significance of lactoperoxidase system in oral health: application and efficacy in oral hygiene products. International Journal of Molecular Sciences.
2019;
20
(6)
:
1443
.
View Article PubMed Google Scholar -
Cherian
D.A.,
Peter
T.,
Narayanan
A.,
Madhavan
S.S.,
Achammada
S.,
Vynat
G.P.,
Malondialdehyde as a marker of oxidative stress in periodontitis patients. Journal of Pharmacy {&}amp; Bioallied Sciences.
2019;
11
(6)
:
297-300
.
View Article PubMed Google Scholar -
Önder
C.,
Kurgan
S.,
Altingöz
S.M.,
Bagis
N.,
Uyanik
M.,
Serdar
M.A.,
Impact of non-surgical periodontal therapy on saliva and serum levels of markers of oxidative stress. Clinical Oral Investigations.
2017;
21
(6)
:
1961-9
.
View Article PubMed Google Scholar -
Warad
S.B.,
Pattanashetti
J.,
Kalburgi
N.,
Koregol
A.,
Rao
S.,
Estimation of salivary malondialdehyde Levels in smokeless tobacco chewers with chronic periodontitis-A cross sectional clinico biochemical study. Odovtos-International Journal of Dental Sciences..
2022;
23
(3)
:
137-46
.
-
Gutteridge
J.M.,
Lipid peroxidation and antioxidants as biomarkers of tissue damage. Clinical Chemistry.
1995;
41
(12 Pt 2)
:
1819-28
.
View Article PubMed Google Scholar -
Ayala
A.,
Muñoz
M.F.,
Argüelles
S.,
Lipid peroxidation: production, metabolism, and signaling mechanisms of malondialdehyde and 4-hydroxy-2-nonenal. Oxidative Medicine and Cellular Longevity.
2014;
2014
(1)
:
360438
.
View Article PubMed Google Scholar -
Dakovic
D.,
Malondialdehyde as an Indicator of Local Oxidative Cell Damage in Periodontitis Patients (Doctoral dissertation, Military Medical Academy).
.
-
Trivedi
S.,
Lal
N.,
Mahdi
A.A.,
Mittal
M.,
Singh
B.,
Pandey
S.,
Evaluation of antioxidant enzymes activity and malondialdehyde levels in patients with chronic periodontitis and diabetes mellitus. Journal of Periodontology.
2014;
85
(5)
:
713-20
.
View Article PubMed Google Scholar -
Aziz
A.S.,
Kalekar
M.G.,
Benjamin
T.,
Suryakar
A.N.,
Prakashan
M.M.,
Bijle
M.N.,
Effect of nonsurgical periodontal therapy on some oxidative stress markers in patients with chronic periodontitis: A biochemical study. World Journal of Dentistry.
2013;
4
(1)
:
17-23
.
View Article Google Scholar -
Veljovic
T.,
Djuric
M.,
Mirnic
J.,
Gusic
I.,
Maletin
A.,
Ramic
B.,
Lipid peroxidation levels in saliva and plasma of patients suffering from periodontitis. Journal of Clinical Medicine.
2022;
11
(13)
:
3617
.
View Article PubMed Google Scholar -
Mostowska
A.,
Hozyasz
K.K.,
Jagodzinski
P.P.,
Maternal MTR genotype contributes to the risk of non-syndromic cleft lip and palate in the Polish population. Clinical Genetics.
2006;
69
(6)
:
512-7
.
View Article PubMed Google Scholar -
Al-Dajani
M.,
Comparison of dental caries prevalence in patients with cleft lip and/or palate and their sibling controls. The Cleft Palate-Craniofacial Journal.
2009;
46
(5)
:
529-31
.
View Article PubMed Google Scholar -
Britton
K.F.,
Welbury
R.R.,
Dental caries prevalence in children with cleft lip/palate aged between 6 months and 6 years in the West of Scotland. European Archives of Paediatric Dentistry ; Official Journal of the European Academy of Paediatric Dentistry.
2010;
11
(5)
:
236-41
.
View Article PubMed Google Scholar -
Parapanisiou
V.,
Gizani
S.,
Makou
M.,
Papagiannoulis
L.,
Oral health status and behaviour of Greek patients with cleft lip and palate. European Archives of Paediatric Dentistry ; Official Journal of the European Academy of Paediatric Dentistry.
2009;
10
(2)
:
85-9
.
View Article PubMed Google Scholar -
Du
W.,
Liu
L.,
Li
F.,
Xu
S.,
Genetic Association between the Risk of Dental Caries and MTR Gene Polymorphism in Chinese Children. The Tohoku Journal of Experimental Medicine.
2024;
263
(1)
:
63-8
.
View Article PubMed Google Scholar -
Antunes
L.A.,
Machado
C.M.,
Couto
A.C.,
Lopes
L.B.,
Sena
F.C.,
Abreu
F.V.,
A polymorphism in the MTRR gene is associated with early childhood caries and underweight. Caries Research.
2017;
51
(2)
:
102-8
.
View Article PubMed Google Scholar
Comments
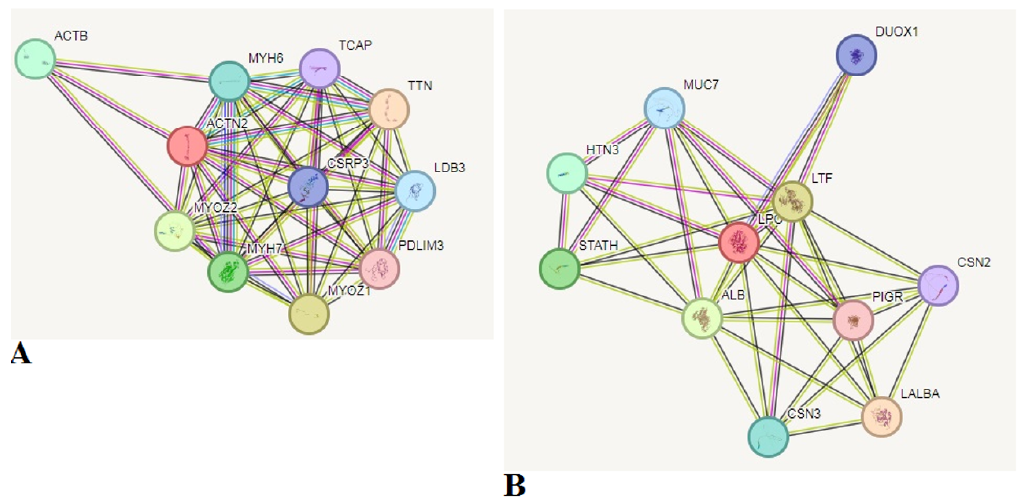
Article Details
Volume & Issue : Vol 11 No 7 (2024)
Page No.: 6592-6602
Published on: 2024-07-31
Citations
Copyrights & License

This work is licensed under a Creative Commons Attribution 4.0 International License.
Search Panel
- HTML viewed - 1297 times
- PDF downloaded - 407 times
- XML downloaded - 36 times