Abstract
Introduction: Leptospirosis, an infectious disease that can spread from animals to humans, requires the development of a safe and effective vaccine. The immunogenic characteristics of LipL41, a conserved outer membrane protein of Leptospira, have been identified as a promising vaccine candidate. In this study, a recombinant DNA construct, pTR-EGFP-LipL41, incorporating the LipL41 gene and hGMCSF adjuvant in the pTR600 vector with a cytomegalovirus (CMV) promoter, was designed and evaluated.
Methods: The Chinese hamster ovary (CHO) cell line was transfected with pTR-EGFP-LipL41 and pTR-EGFP-hGMCSF-LipL41 using Lipofectamine 2000, and fluorescence microscopy analyzed their expression.
Results: The expression analysis demonstrated successful expression of pTR-EGFP-LipL41 and pTR-EGFP-hGMCSF-LipL41 in CHO cells. In vitro analysis of cell lines further assessed the expression of chemokines and cytokines, and molecular docking analyses were conducted to investigate interactions between various adjuvants (hGMCSF, hIgGFc, and hC3d) and LipL41. Docking studies uncovered key interactions between LipL41 and other adjuvants. The constructed recombinant DNA and molecular adjuvants exhibited a robust immunogenic response.
Conclusion: Further evaluation in suitable animal models may establish its effectiveness as a productive and safe immunogenic molecule against leptospiral infection.
Introduction
Leptospirosis is a significant zoonotic disease caused by obligate pathogenic organisms known as gram-negative spirillates belonging to Leptospira 1, 2. The disease is an important worldwide health issue, particularly in tropical and subtropical regions, and is expected to result in over 59,000 deaths annually3. The Leptospira bacteria have numerous species, with over 300 serovars within 64 species4. This complexity has been identified as a significant challenge in combating this pathogen5. This disease is typically spread through direct contact with the tissues, urine, or other bodily fluids of infected hosts6. It can also be transmitted indirectly through contact with objects that have come into contact with infected rodents, which are the primary carriers of the bacterium. The clinical symptoms of this condition can range from mild, such as headaches, muscle pain, and fever, to severe, such as jaundice, kidney dysfunction, lung bleeding, and failure of several organs7. Therefore, it is crucial to diagnose and prevent the disease early.
Nevertheless, leptospirosis continues to be a widespread disease, and the existing vaccines are limited in terms of their accessibility. Additional doses are typically required, and the vaccines only target a small number of specific serovars to offer cross-protective protection8. The current Leptospira vaccines usually consist of whole-killed bacteria or outer membrane proteins (OMPs)9. The proliferation of numerous and varied Leptospira serovars presents difficulty in developing a vaccine that effectively covers all the serovars. The outer membrane proteins (OMPs), including LipL32, LipL41, and OmpL1, play a crucial role in the bacteria's capacity to attach to host factors and regulate immune responses10, 11, 12. These lipoproteins are significant antigens that stimulate immunological responses and can thus be utilized to develop potent vaccinations13. Among these, LipL41 holds particular significance due to its homogeneous expression throughout pathogenic Leptospira species and absence in avirulent Leptospira14. Furthermore, it has been utilized in serodiagnosis and is believed to have the potential to be integrated into vaccines15.
The utilization of DNA vaccines has ushered in a new age in vaccine development and has significantly transformed conventional practices16. DNA vaccines are referred to as third-generation vaccines due to their advantageous characteristics, including stability, simplicity, and speed in vaccine design, cost-effectiveness in production, and safety17. The vaccines mentioned above function through the direct administration of plasmid DNA that contains the genetic code for the desired antigen18. This genetic material is subsequently produced within cells, triggering immunological responses. Mammalian expression systems are frequently utilized for DNA vaccines due to their ability to undergo post-translational modifications and efficiently process proteins, mimicking the conditions of the host organism19. DNA vaccines for leptospirosis have developed extensively, notably pTarget/LipL32, which has demonstrated the ability to elicit humoral immune responses and recognize native L. interrogans membrane proteins15. Lately, there has been a discussion on how molecular adjuvants can enhance the effectiveness of vaccines. Studies have shown that adjuvants, including hGMCSF (human granulocyte-macrophage colony-stimulating factor), stimulate the innate immune system, guide cell mobility, and enhance the collaboration of immune cells in presenting antigens20. DNA vaccines contain elements of these adjuvants. Multiple experiments have proven that molecular adjuvants can improve DNA vaccines' immunogenicity and stimulate cytokine production and antigen processing under specific conditions21.
Therefore, in this investigation, we chose to utilize a mammalian expression system to express the LipL41 ORF of the Leptospira and the EGFP and hGMCSF. The recombinant DNA was introduced into Chinese Hamster Ovary (CHO) cells because of their widespread use in protein manufacturing, as they reliably replicate the desired quantities22. The cells were transfected using Lipofectamine 2000 and calcium phosphate techniques, and the expression of the constructs containing the EGFP was verified using fluorescence microscopy. The immunological response to the recombinant DNA constructs was assessed by analyzing the production of cytokines and chemokines following transfection. Furthermore, protein-protein docking was conducted to determine the interaction between LipL41 and molecular adjuvants (hGMCSF, hIgGFC, and hC3d), demonstrating that hGMCSF is the most effective adjuvant for enhancing the vaccination potential of the peptide. Considering the data collected from the docking experiments and the expression data of hGMCSF in CHO cells, LipL41, when used as a heterologous DNA vaccine with hGMCSF, shows potential as a candidate for a leptospirosis vaccine. This work marks the beginning of developing second-generation leptospira vaccines that target many Leptospira serovars. This study further emphasizes the importance of investigating molecular adjuvants and DNA vaccine technologies and the integral role of the scientific community in creating necessary preventive methods against leptospirosis.
Methods
E. coli XL10 was used to generate and transform all recombinant plasmids. The sequences of all cloned PCR-amplified products were confirmed by sequencing. Sambrook's protocols for cloning and isolating plasmid DNA were used23. Hi-Media Labs provided all the chemicals, reagents, and antibiotics (India). Thermo Fisher Scientific supplied molecular biology reagents, the pTZ57R/T vector, and restriction endonucleases (USA). E. coli XL10 were grown in LB buffer containing the requisite concentrations of antibiotics for selective growth (Sigma-Aldrich, USA). We utilized molecular-grade water (Hi-Media, India) and phosphate-buffered saline (PBS) for all cloning and cell culture procedures. The cell culture media, Roswell Park Memorial Institute (RPMI), along with 10% fetal bovine serum (FBS) and antibiotics, were procured from Hi-Media (India). Calcium phosphate was obtained from Sigma-Aldrich (USA) for experimental use. The National Centre for Cell Science (NCCS) provided the CHO cell line.
Molecular Modeling and Docking
The sequence of LipL41 was retrieved from UniProt (www.uniprot.org) with the UniProt ID: Q33BM7, comprising 355 residues. A sequence similarity search for the target sequence was conducted using the Protein Data Bank on the BLAST server24. The Modeller software (version 9.9) was utilized to generate the structural model of the LipL41 protein25. Following prediction, the generated structure was saved in PDB format, and scores were calculated based on Discrete Optimized Protein Energy (DOPE)26. Standard programs evaluated the quality of the optimized model27, 28, 29. The final optimized structural model and spiral model were used for further analysis. Three significant glycoproteins or molecular adjuvants (hC3d, GMCSF, and hIgGFC) were employed to evaluate the binding effectiveness of the LipL41 protein. ClusPro 2.0 was used to investigate the potency of LipL41's interactions with molecular adjuvants30.
Construction of pTR-EGFP-LipL41 and pTR-EGFP-hGMCSF-LipL41 Plasmid
The EGFP (Enhanced Green Fluorescent Protein) gene, generously provided by Dr. B. Ashok Kumar from Madurai Kamaraj University, India, was amplified from the pEGFP plasmid. Furthermore, the study utilized the hGMCSF (human Granulocyte-Macrophage Colony-Stimulating Factor) gene, which was graciously provided by Dr. Tracy Willson from The Walter and Eliza Hall Institute of Medical Research, Australia. The transcription of these two genes (EGFP and hGMCSF) was achieved by using pairs of gene-specific primers flanked by a recognition sequence for a restriction enzyme, together with normal PCR conditions. Following electrophoresis on a 0.8% agarose gel, the PCR products underwent purification before being ligated into the pTZ57R/T vector. The resulting ligation mixture was then introduced into E. coli XL10 competent cells. Subsequently, colony PCR was employed to verify the identity of the selected recombinant colonies.
The recombinant DNA was processed using restriction enzyme-bounded primers, and the 1% agarose gel electrophoresis revealed 1056 bp of LipL41, 717 bp of EGFP, and 453 bp of the hGMCSF gene release. The recombinant clones (pTZ-LipL41, pTZ-EGFP, and pTZ-hGMCSF) were sub-cloned into the pTR600 mammalian expression vector (a kind gift from Dr. Ted Ross, University of Pittsburgh, USA), which was constructed to carry the cytomegalovirus (CMV). The recombinant DNA constructs were named pTR-EGFP-LipL41 and pTR-EGFP-hGMCSF-LipL41. Finally, the recombinant plasmids were extracted using the alkaline lysis method and were purified with a 30 mM concentration of MgCl2 and different concentrations of polyethylene glycol (PEG) 40% and PEG 6000. Afterwards, the purified plasmids were dissolved using molecular-grade water. Finally, the recombinant plasmids were transfected into the CHO cell line for EGFP expression.
Cell Viability
The CHO cell line was cultured in RPMI medium and seeded into cell culture flasks. These flasks were then placed in an incubator set at 37°C with a 5% CO2 atmosphere for 12 hours. Following incubation, adherent cells were observed using an inverted microscope. Subsequently, the adherent cells were rinsed with 1% trypsin solution and centrifuged at 600 rpm for 5 minutes. The Trypan Blue dye exclusion test determines the number of viable cells in a cell suspension. The cells were counted using a hemocytometer under the light microscope (100X). The following equation determined the number of viable cells: percentage of viable cells (cells/ml) = (average number of cells in 4 quadrants) x dilution factor for the size of the quadrant (i.e., 10,000) x dilution factor for the addition of trypan blue. Finally, 5 x 105 cells per well were added into 6-well plates (35 mm2). The total number of cells within the flask was determined using the following equation: Total cells in the flask = cells/ml x mL.
Lipofectamine-Mediated Transient EGFP Expression
The recombinant DNA constructs pTR-EGFP-LipL41 and pTR-EGFP-hGMCSF-LipL41 were introduced into the CHO cell line through transfection. The CHO cell lines were cultured in RPMI media, both complete and incomplete, supplemented with 10% FBS and a penicillin-streptomycin antibiotic solution (Hi-Media, India). The cell cultures were maintained at 37°C in a humidified atmosphere with 5% CO2 concentration.
In Vitro DNA Transfection in CHO Cell Line
The CHO cells were cultured by seeding 5 x 105 cells into 6-well plates (35 mm2), and the plates were incubated at 37°C and 5% CO2 overnight. The CHO cells were transfected with DNA constructs pTR600, pTR-EGFP-LipL41, and pTR-EGFP-hGMCSF-LipL41 using Lipofectamine™ 2000. Briefly, the supernatant 10-15 µL with five µg of plasmid DNA was mixed with RPMI media (Tube A). Tube marked as B has 5μL of Lipofectamine™ 2000 reagent. The contents of tubes A & B were mixed and kept at 37°C for 30 minutes. Subsequently, 1.5 mL of reduced serum media was added to each well, and the master mix (~400μL) was added to each well of 6-well plates (35 mm2). The plates were incubated for 72 hours, performed in triplicate, and repeated twice.
Cytotoxicity Assay
The cytotoxicity assay was employed on pTR-EGFP-LipL41 and pTR-EGFP-hGMCSF-LipL41. In summary, CHO cells (5 x 105) were subjected to treatment with Lipofectamine 2000 at concentrations of 2.5, 5, 7.5, and 10, and then incubated in the dark for 12 hours. Tests were run in triplicates, and sample sizes (positive and negative controls) were developed. The positive control wells contained Lipofectamine transfect plasmid DNA (pTR-EGFP-LipL41 and pTR-EGFP-hGMCSF-LipL41), while the negative control wells contained the same number of CHO cells and the pTR600 vector without EGFP. The mixture was incubated for hours at 37°C in a CO2 incubator with a CO2 concentration of 5%. The RPMI growth medium was withdrawn after incubation, and 100 mL of growth medium was added with various doses of plasmid DNA pTR-EGFP-LipL41 and pTR-EGFP-hGMCSF-LipL41 (1-10 mg/mL) and maintained for 12 hours at 37°C in a humidified incubator with 5% CO2. After incubation, the culture media was withdrawn correctly, and 150 μL of MTT was added to each well. MTT cultures were maintained for 3 hours at 37°C in a humidified incubator with a CO2 concentration of 5%. The proportion of viable cells was calculated using the formula: % viability of cells = (OD of test / OD of control) x 100.
Fluorescent Microscopic Analysis
Transfected CHO cells were washed with phosphate-buffered saline (PBS). Washed cells were carefully placed on a glass slide, and the periphery was sealed with nail paint. Mounted slides were observed under the fluorescence microscope. Protein expression (GFP tagged) was checked by fluorescent and confocal microscopy.
Real-Time PCR
The pTR-EGFP-LipL41 and pTR-EGFP-hGMCSF-LipL41 were transfected into the CHO cell line using Lipofectamine™ 2000. The cells that received the genetic material were cultured for 24 hours at a temperature of 37°C in a CO2 incubator with a CO2 concentration of 5%. After incubation, RNA isolation was carried out using the Hi-PurA™ Total RNA Miniprep Purification Kit, and cDNA was synthesized using the RevertAid First Strand cDNA Synthesis Kit (Thermo Scientific, USA). A Nanodrop analyzer checked the concentration of cDNA. The 100 ng of cDNA was used to study cytokine and chemokine expression using specific primers. The samples underwent amplification using 20 µL of cDNA, including forward and reverse primers, along with 1X SYBR Green I Master mix (Roche) and molecular-grade water. The PCR cycle consisted of denaturation at 25°C for 10 minutes, annealing at 42°C for 30 minutes, and extension at 85°C for 5 minutes. Details of the RT-PCR primers are provided in Table 1.
Cytokines | Forward Primer (5’ – 3’) | Reverse Primer (5’ – 3’) |
IL-2 | AATTCGGTACATCCTCACGG | GGTTGTTTTCTGCCAGTGCC |
IL-6 | AATTCGGTACATCCTCGACGG | GGTTGTTTTCTGCCAGTGCC |
IL-8 | GACCACACTGCGCCAACAC | CTTCTCCACAACCCTCTGCAC |
IL-10 | GGTTGCCAAGCCTTGTCTGA | AGGGAGTTCACATGCGCCT |
TNF α | GGAGAAGGGTGACCGACTCA | CTGCCCAGACTCGGCAA |
IFN γ | AGCTCTGCATCGTTTTGGGT | CGCTTCCCTGTTTTAGCTGC |
CXCL11 | CCTTGGCTGTGATATTGTGTGCTA | CCTATGCAAAGACTGCGTCCTC |
CCL17 | TGAGGACGCTCCAGGGATG | AACGGTGGACGTCCCAGGTA |
β- ACTIN | TCACCCACACTGTGCCCATCTACG | CAGCGGAACCGCTCATTGCCAATG |
Protein 1 | Protein 2 | PDB ID of Protein 2 | Resolution (Å) | Interaction Energy (Kcal/mol) | Number of Hydrogen Bonds |
LipL42 (Theoretical model) | hGMCSF | 2GMF_A | 2.4 | -594.9 | 246 |
hC3D | 1C3D_A | 1.8 | -555.9 | 198 | |
MCO | Model | Nil | -428.7 | 150 |
Results
Molecular Modelling and Molecular Docking
The intricate configuration was produced by employing ClusPro software to dock LipL41 with three cytokines30. The output generated by ClusPro resulted in the identification of the ten most highly ranked docked structures, which were sorted based on their respective binding energies. The conformation with the least binding energy was selected for further analysis through docking, as indicated in Table 2. The docking outcomes indicate that LipL41 exhibits superior interaction with hGMCSF, as evidenced by the interaction energy of -594.9 Kcal/mol and the formation of 246 hydrogen bonds. The hC3d-LipL41 complex demonstrates an interaction energy of -555.9 Kcal/mol and is distinguished by the creation of 198 hydrogen bonds. In contrast, the hIgGFC-LipL41 complex (MCO) displays an interaction energy of -428.7 Kcal/mol and involves the formation of 150 hydrogen bonds. Upon assessment of the binding energies of the three complexes, it was observed that the GMCSF-LipL41 complex exhibited the lowest binding energy. This finding suggests that the complex in question possesses greater binding efficacy.
Construction of Mammalian Expression Plasmid pTR-EGFP-LipL41
The pTZ-LipL41 and pTR-EGFP DNA samples were subjected to restriction digestion using BamHI and XhoI enzymes. The resulting DNA fragments were then examined using 0.8% agarose gel electrophoresis. The digested fragments of pTZ-LipL41 (1065-bp), EGFP (717-bp), and pTR (3.8-kb) were purified by extracting them from the agarose gel, and then a ligation reaction was carried out for the fragments overnight. The ligation mixture was introduced into competent E. coli XL10 cells through transformation, and the resulting transformed colonies were examined for positive clones using colony PCR. The plasmid was extracted from the colony of the positive patch using the alkaline lysis technique, and the clones were verified using restriction digestion analysis with HindIII and XhoI enzymes. The LipL41 and EGFP (1.8-kb) genes were released (Figure 1). The pTR-EGFP-LipL41 plasmids were sequenced and associated with the original LipL41 EGFP sequences. Basic Local Sequence Alignment (BLAST) analysis showed the recombinant clones were 100% identical to the original sequences.
Construction of Mammalian Expression Plasmid pTR-EGFP-hGMCSF-LipL41
The pTR-EGFP-LipL41 and hGMCSF DNA samples were subjected to restriction digestion using BamHI and XhoI enzymes. The resulting digested DNA fragments were then examined using 0.8% agarose gel electrophoresis. The product resulting from the digestion of pTR-EGFP-LipL41 (1.8-kb) and hGMCSF (453-bp) using restriction enzymes was purified by extracting it from the agarose gel. Subsequently, an overnight ligation reaction was carried out. The ligation mixture was introduced into competent E. coli XL10 cells, and the resulting transformed cells were examined for positive clones by colony PCR. The plasmid was extracted from the colony of the positive patch, and the clones were verified by restriction digestion analysis using NheI and StuI enzymes. A release of EGFP-hGMCSF-LipL41 (2-kb) was observed (Figure 2). The pTR-EGFP-hGMCSF-LipL41 plasmids were sequenced and associated with the original LipL41, EGFP, and hGMCSF sequences. The BLAST analysis showed the recombinant clones were 100% identical to the original sequences.
Estimation of Cytotoxic Effects of pTR-EGFP-LipL41 and pTR-EGFP-hGMCSF-LipL41 of Recombinant DNA Constructs
In this study, we examined the cytotoxic effects of pTR-EGFP-LipL41, pTR-EGFP-hGMCSF-LipL41, and recombinant DNA constructs on the CHO cell line. CHO cells were seeded into 96-well plates and exposed to varying concentrations (ranging from 2.5 to 10 μg/mL) of Lipofectamine. Analysis using the MTT cell viability assay unveiled a dose-dependent trend of cytotoxicity, as illustrated in Figure 3. Additionally, cell viability was assessed. With the 5 μg/mL concentration of Lipofectamine 2000, approximately 90% of cell viability was observed (Figure 3).
In Vitro Transfection of Plasmid DNA into CHO Cell Line
The effectiveness of the engineered recombinant DNA complexes in expressing EGFP in the eukaryotic (CHO cell line) system was evaluated by introducing plasmid DNA of pTR600, pTR-EGFP-LipL41, and pTR-EGFP-hGMCSF-LipL41 through transfection. The results were compared with the negative control, pTR600. Fluorescent microscope analysis was conducted 24 and 48 hours after transfection of pTR600, pTR-EGFP-LipL41, and pTR-EGFP-hGMCSF-LipL41 to observe EGFP expression. The results may be seen in Figure 4.
Quantification of Cytokine and Chemokine
The CHO cells were genetically modified with pTR-EGFP-LipL41 and pTR-EGFP-hGMCSF-LipL41 and then induced with Phorbol 12-myristate 13-acetate (PMA) to produce cytokines and chemokines. Cells were collected following 24 hours of incubation, RNA was isolated, and cDNA was generated using a reverse transcriptase enzyme. An equivalent concentration of the cDNA template was employed to examine the differential cytokine and chemokine production. The cytokines IL-2, IL-6, IL-8, IL-10, IFNγ, TNF-α, and the chemokines CXCL 11 and CCL17 were all subjected to divergent cytokine profiling. Real-time quantitative PCR was conducted utilizing the ABI 7000 Real-Time PCR instrument from Conquer Scientific. β-actin served as an internal control for normalization of the values. The expression of IL-2, IL-6, IL-8, IL-10, IFNγ, CXCL 11, and CCL17 was assessed for both EGFP-LipL41 and EGFP-hGMCSF-LipL41 constructs. Notably, no significant fold change in the expression of TNF-α was observed, as depicted in Figure 5.
Discussion
This study showcases the successful transfection of CHO cell lines with pTR-EGFP-LipL41 and pTR-EGFP-hGMCSF-LipL41 recombinant plasmids. This achievement underscores the potential of recombinant DNA vaccines in the fight against Leptospira infections. LipL41, an outer membrane protein of Leptospira, is a well-studied target antigen for vaccine development due to its strong immunological characteristics15. The addition of the hGMCSF gene, a potent immunomodulator, further enhances the immune response by stimulating cytokine production and activating the immune defense to a highly protective level31.
As previously explained, the successful transfer of genes to the CHO cell line was verified by observing the expression of EGFP using recombinant DNA constructs. Fluorescence microscopy revealed a high percentage of transfection effectiveness, with EGFP localized in the nucleus of the transfected CHO cells. This finding aligns with the conclusions of other researchers who have previously utilized EGFP as a marker to assess the effectiveness of transfection and gene expression in mammalian cells32, 33.
Our work also attempted to estimate the mRNA levels of cytokines after introducing pTR-EGFP-LipL41 and pTR-EGFP-hGMCSF-LipL41 into CHO cell lines through transfection, facilitating comprehension of the connections between cytokines and the immune response, specifically in the context of resolving infections, particularly microbial disorders such as leptospirosis. The current study has identified an elevation in cytokines, specifically IL-2, IL-6, IL-8, IL-10, and IFNγ. Additionally, chemokines such as CXCL11 and CCL17 have also shown an increase. This study suggests that the observed recombinant DNA constructions induce both an inflammatory response and a slowing of inflammation, resulting in a balanced immune response. Nevertheless, the potential rise in cholesteryl ester content caused by ADCs may lead to decreased TNF-α levels. This decrease in TNF-α levels may indicate an anti-inflammatory effect, which might help mitigate the down-regulation and tissue damage often due to this process34, 35, 36.
IL-2 and IFNγ, two cytokines, exhibit the most significant increases. These cytokines are highly effective in enhancing the body's protective immune response against microbial diseases37, 38. IL-2 promotes the survival, development, and proliferation of lymphoid progenitor cells, while IFNγ boosts the activity of macrophages and improves antigen presentation by increasing the expression of MHC class I and II molecules39. The upregulation of these cytokines suggests that the use of recombinant DNA constructions could be highly beneficial in inducing a robust immune response, particularly in the context of vaccinations. Moreover, the elevated level of IL-8, a type of chemokine known for its ability to attract neutrophils, can enhance the recruitment of immune cells to the site of infection and bolster the body's ability to eradicate the pathogen40. The increase in the IL-10/IL-6 ratio and the potent anti-inflammatory cytokine IL-10 production support the idea of a shifting immune response towards a balanced state. This is because IL-10 can help control inflammation and prevent tissue damage during the infection41. Remarkably, the outcome demonstrates a reduction in TNF-α levels, suggesting the presence of an anti-inflammatory impact. While TNF-α is a potent pro-inflammatory cytokine that is involved in initiating immune responses and is known for its diverse functions, excessive release of TNF-α can lead to severe inflammation and tissue damage3. The reduction in TNF-α levels may also indicate that the recombinant DNA constructs can mitigate excessive inflammation without affecting the immune response.
CONCLUSION
In conclusion, the findings of this study provide valuable insights into the potential of LipL41 and hGMCSF as promising targets for DNA vaccine development against leptospirosis. The transfection of CHO cell lines with recombinant DNA constructs demonstrated efficient gene expression and cytokine production, which are critical for eliciting a strong and balanced immune response. Further research is needed to evaluate the in vivo efficacy of these constructs and their potential for use in vaccine development. Additionally, optimizing the delivery methods and exploring alternative adjuvants may further enhance these DNA vaccines' immunogenicity and protective efficacy.
Abbreviations
CHO: Chinese Hamster Ovary, CMV: Cytomegalovirus, DNA: Deoxyribonucleic Acid, DOPE: Discrete Optimized Protein Energy, E. coli: Escherichia coli, EGFP: Enhanced Green Fluorescent Protein, FBS: Fetal Bovine Serum, GFP: Green Fluorescent Protein, GMCSF: Granulocyte-Macrophage Colony-Stimulating Factorh, C3d: Human Complement Component 3d, hGMCSF: Human Granulocyte-Macrophage Colony-Stimulating Factor, hIgGFc: Human Immunoglobulin G Fc, IFNγ: Interferon Gamma, IL: Interleukin, LB: Lysogeny Broth, MHC: Major Histocompatibility Complex, MTT: 3-(4,5-Dimethylthiazol-2-yl)-2,5-Diphenyltetrazolium Bromide, NCCS: National Centre for Cell Science, OMPs: Outer Membrane Proteins, ORF: Open Reading Frame, PBS: Phosphate-Buffered Saline, PCR: Polymerase Chain Reaction, PEG: Polyethylene Glycol, PDB: Protein Data Bank, PMA: Phorbol 12-Myristate 13-Acetate, RNA: Ribonucleic Acid, RPMI: Roswell Park Memorial Institute, RT-PCR: Real-Time Polymerase Chain Reaction, TNFα: Tumor Necrosis Factor Alpha
Acknowledgments
The author would like to thank Madurai Kamaraj University's DST-SAP facility for availing of fluorescence microscopy. The author thanked the DST- PURSE facility at Madurai Kamaraj University's School of Biotechnology for offering RT-PCR and RUSA for the reagents and kits.
Author’s contributions
Conceptualization: GP, SS, MM. Data curation: GP, SP, DS. Methodology: GP, SP, DS. Writing – original draft: KMS, HS. Writing – review & editing: GP, KMS, HS. All authors read and approved the final manuscript.
Funding
The first author gratefully thanks for the financial assistance received from UGC-BSR-F.No.25-1/2014-15(BSR)/7-120/2007(BSR).
Availability of data and materials
Data and materials used and/or analyzed during the current study are available from the corresponding author on reasonable request.
Ethics approval and consent to participate
Not applicable.
Consent for publication
Not applicable.
Competing interests
The authors declare that they have no competing interests.
References
-
Leptospirosis
N.L.,
Leptospirosis. Clinical Microbiology Reviews.
2001;
14
:
296-326
.
View Article PubMed Google Scholar -
Bradley
E.A.,
Lockaby
G.,
Leptospirosis and the Environment: A Review and Future Directions. Pathogens (Basel, Switzerland).
2023;
12
(9)
:
1167
.
View Article PubMed Google Scholar -
Barazzone
G.C.,
Teixeira
A.F.,
Azevedo
B.O.,
Damiano
D.K.,
Oliveira
M.P.,
Nascimento
A.L.,
Revisiting the Development of Vaccines Against Pathogenic Leptospira: Innovative Approaches, Present Challenges, and Future Perspectives. Frontiers in Immunology.
2022;
12
:
760291
.
View Article PubMed Google Scholar -
Azevedo
I.R.,
Amamura
T.A.,
Isaac
L.,
Human leptospirosis: in search for a better vaccine. Scandinavian Journal of Immunology.
2023;
98
(5)
:
e13316
.
View Article PubMed Google Scholar -
Fraga
T.R.,
Carvalho
E.,
Isaac
L.,
Barbosa
A.S.,
Leptospira and leptospirosis. In book: Molecular Medical Microbiology.
2024;
:
1849-71
.
-
Vincent
A.T.,
Schiettekatte
O.,
Goarant
C.,
Neela
V.K.,
Bernet
E.,
Thibeaux
R.,
Revisiting the taxonomy and evolution of pathogenicity of the genus Leptospira through the prism of genomics. PLoS neglected tropical diseases.
2019;
13
(5)
:
e0007270
.
View Article PubMed Google Scholar -
Nagraik
R.,
Kaushal
A.,
Gupta
S.,
Sharma
A.,
Kumar
D.,
Leptospirosis: a systematic review. Journal of Microbiology, Biotechnology and Food Sciences.
2021;
9
(6)
:
1099-109
.
View Article Google Scholar -
Palaniappan
R.U.,
Ramanujam
S.,
Chang
Y.F.,
Leptospirosis: pathogenesis, immunity, and diagnosis. Current Opinion in Infectious Diseases.
2007;
20
(3)
:
284-92
.
View Article PubMed Google Scholar -
Gupta
S.,
Sharma
N.,
Naorem
L.D.,
Jain
S.,
Raghava
G.P.,
Collection, compilation and analysis of bacterial vaccines. Computers in Biology and Medicine.
2022;
149
.
View Article PubMed Google Scholar -
Barnett
J.K.,
Barnett
D.,
Bolin
C.A.,
Summers
T.A.,
Wagar
E.A.,
Cheville
N.F.,
Expression and distribution of leptospiral outer membrane components during renal infection of hamsters. Infection and immunity.
1999;
67
(2)
:
853-61
.
View Article PubMed Google Scholar -
Zuerner
R.L.,
Host Response to Leptospira Infection BT - Leptospira and Leptospirosis. 2015;
:
223-50
.
View Article Google Scholar -
Guerreiro
H.,
Croda
J.,
Flannery
B.,
Mazel
M.,
Matsunaga
J.,
Reis
M. Galvão,
Leptospiral proteins recognized during the humoral immune response to leptospirosis in humans. Infection and immunity.
2001;
69
(8)
:
4958-68
.
View Article PubMed Google Scholar -
Zhao
T.,
Cai
Y.,
Jiang
Y.,
He
X.,
Wei
Y.,
Yu
Y.,
Vaccine adjuvants: mechanisms and platforms. Signal Transduction and Targeted Therapy.
2023;
8
(1)
:
283
.
View Article PubMed Google Scholar -
Fernandes
L.G.,
Teixeira
A.F.,
Nascimento
A.L.,
Nascimento ALTO. Evaluation of Leptospira interrogans knockdown mutants for LipL32, LipL41, LipL21, and OmpL1 proteins. Frontiers in Microbiology.
2023;
14
:
1199660
.
View Article Google Scholar -
Takahashi
Teixeira
Nascimento
T
MB,
The leptospiral LipL21 and LipL41 proteins exhibit a broad spectrum of interactions with host cell components. Virulence.
2021;
12
(1)
:
2798-813
.
View Article PubMed Google Scholar -
Koff
W.C.,
Rappuoli
R.,
Plotkin
S.A.,
Historical Advances in Structural and Molecular Biology and How They Impacted Vaccine Development. Journal of Molecular Biology.
2023;
435
(13)
:
168113
.
View Article PubMed Google Scholar -
Melo
A.R. S.,
L.S. de Macêdo,
M.D. Invenção,
I.A. de Moura,
M.A. da Gama,
C.M. de Melo,
A.J. Silva,
M.V. Batista,
A.C. Freitas,
hird-Generation Vaccines: Features of Nucleic Acid Vaccines and Strategies to Improve Their Efficiency. Infection and immunity.
2022;
69
(8)
:
4958-68
.
View Article PubMed Google Scholar -
Montero
D.A.,
Vidal
R.M.,
Velasco
J.,
Carreño
L.J.,
Torres
J.P.,
Benachi
O.M.,
Two centuries of vaccination: historical and conceptual approach and future perspectives. Frontiers in public health.
2024;
11
:
1326154
.
View Article PubMed Google Scholar -
Lu
B.,
Lim
J.M.,
Yu
B.,
Song
S.,
Neeli
P.,
Sobhani
N.,
The next-generation DNA vaccine platforms and delivery systems: advances, challenges and prospects. Frontiers in Immunology.
2024;
15
:
1332939
.
View Article PubMed Google Scholar -
Fan
J.,
Jin
S.,
Gilmartin
L.,
Toth
I.,
Hussein
W.M.,
Stephenson
R.J.,
Advances in Infectious Disease Vaccine Adjuvants. Vaccines.
2022;
10
(7)
:
1120
.
View Article PubMed Google Scholar -
Pagliari
S.,
Dema
B.,
Sanchez-Martinez
A.,
Zurbia-Flores
G. Montalvo,
Rollier
C.S.,
DNA Vaccines: History, Molecular Mechanisms and Future Perspectives. Journal of Molecular Biology.
2023;
435
(23)
:
168297
.
View Article PubMed Google Scholar -
Glinšek
K.,
Bozovi\vcar
K.,
Bratkovi\vc
T.,
CRISPR Technologies in Chinese Hamster Ovary Cell Line Engineering. International Journal of Molecular Sciences.
2023;
24
(9)
:
8144
.
View Article PubMed Google Scholar -
Sambrook
J.,
Fritsch
E.F.,
Maniatis
T.,
Molecular Cloning: A Laboratory ManualCold Spring Harbor Laboratory Press 1989.
Google Scholar -
Berman
H.M.,
Westbrook
J.,
Feng
Z.,
Gilliland
G.,
Bhat
T.N.,
Weissig
H.,
The Protein Data Bank. Nucleic Acids Research.
2000;
28
(1)
:
235-42
.
View Article PubMed Google Scholar -
Fiser
A.,
Sali
A.,
Modeller: generation and refinement of homology-based protein structure models. Modeller: generation and refinement of homology-based protein structure models.
2003;
374
:
461-91
.
View Article PubMed Google Scholar -
Eramian
D.,
Shen
M.Y.,
Devos
D.,
Melo
F.,
Sali
A.,
Marti-Renom
M.A.,
A composite score for predicting errors in protein structure models. Protein Science.
2006;
15
(7)
:
1653-66
.
View Article PubMed Google Scholar -
Benkert
P.,
Tosatto
S.C.,
Schomburg
D.,
QMEAN: A comprehensive scoring function for model quality assessment. Proteins.
2008;
71
(1)
:
261-77
.
View Article PubMed Google Scholar -
Colovos
C.,
Yeates
T.O.,
Verification of protein structures: patterns of nonbonded atomic interactions. Protein Science.
1993;
2
(9)
:
1511-9
.
View Article PubMed Google Scholar -
Shah
P.M.,
Idiopathic hypertrophic subaortic stenosis (hypertrophic obstructive cardiomyopathy) changing concepts-1975. Chest.
1975;
68
(6)
:
814-7
.
View Article PubMed Google Scholar -
Comeau
S.R.,
Gatchell
D.W.,
Vajda
S.,
Camacho
C.J.,
ClusPro: a fully automated algorithm for protein-protein docking. Nucleic acids research.
2004;
32
(Web Server issue)
:
W96-9
.
View Article PubMed Google Scholar -
Bhattacharya
P.,
Thiruppathi
M.,
Elshabrawy
H.A.,
Alharshawi
K.,
Kumar
P.,
Prabhakar
B.S.,
GM-CSF: an immune modulatory cytokine that can suppress autoimmunity. Cytokine.
2015;
75
(2)
:
261-71
.
View Article PubMed Google Scholar -
Rizzuto
R.,
Brini
M.,
Pizzo
P.,
Murgia
M.,
Pozzan
T.,
Chimeric green fluorescent protein as a tool for visualizing subcellular organelles in living cells. Current Biology.
1995;
5
(6)
:
635-42
.
View Article PubMed Google Scholar -
Ansari
A.M.,
Ahmed
A.K.,
Matsangos
A.E.,
Lay
F.,
Born
L.J.,
Marti
G.,
Cellular GFP Toxicity and Immunogenicity: Potential Confounders in in Vivo Cell Tracking Experiments. Stem Cell Reviews and Reports.
2016;
12
(5)
:
553-9
.
View Article PubMed Google Scholar -
Bachmann
M.F.,
Kopf
M.,
Balancing protective immunity and immunopathology. Current Opinion in Immunology.
2002;
14
(4)
:
413-9
.
View Article PubMed Google Scholar -
Laurence
A.,
Tato
C.M.,
Davidson
T.S.,
Kanno
Y.,
Chen
Z.,
Yao
Z.,
Interleukin-2 signaling via STAT5 constrains T helper 17 cell generation. Immunity.
2007;
26
(3)
:
371-81
.
View Article PubMed Google Scholar -
Dinarello
C.A.,
An IL-1 family member requires caspase-1 processing and signals through the ST2 receptor. Immunity.
2005;
23
(5)
:
461-2
.
View Article PubMed Google Scholar -
Chirathaworn
C.,
Supputtamongkol
Y.,
Lertmaharit
S.,
Poovorawan
Y.,
Cytokine levels as biomarkers for leptospirosis patients. Cytokine.
2016;
85
:
80-2
.
View Article PubMed Google Scholar -
Kany
S.,
Vollrath
J.T.,
Relja
B.,
Cytokines in Inflammatory Disease. International Journal of Molecular Sciences.
2019;
20
(23)
:
6008
.
View Article PubMed Google Scholar -
Senavirathna
I.,
Rathish
D.,
Agampodi
S.,
Cytokine response in human leptospirosis with different clinical outcomes: a systematic review. BMC Infectious Diseases.
2020;
20
(1)
:
268
.
View Article PubMed Google Scholar -
Cagliero
J.,
Villanueva
S.Y.,
Matsui
M.,
Leptospirosis Pathophysiology: Into the Storm of Cytokines. Frontiers in Cellular and Infection Microbiology.
2018;
8
:
204
.
View Article PubMed Google Scholar -
Li
S.,
Wang
M.,
Ojcius
D.M.,
Zhou
B.,
Hu
W.,
Liu
Y.,
Leptospira interrogans infection leads to IL-1β and IL-18 secretion from a human macrophage cell line through reactive oxygen species and cathepsin B mediated-NLRP3 inflammasome activation. Microbes and Infection.
2018;
20
(4)
:
254-60
.
View Article PubMed Google Scholar
Comments
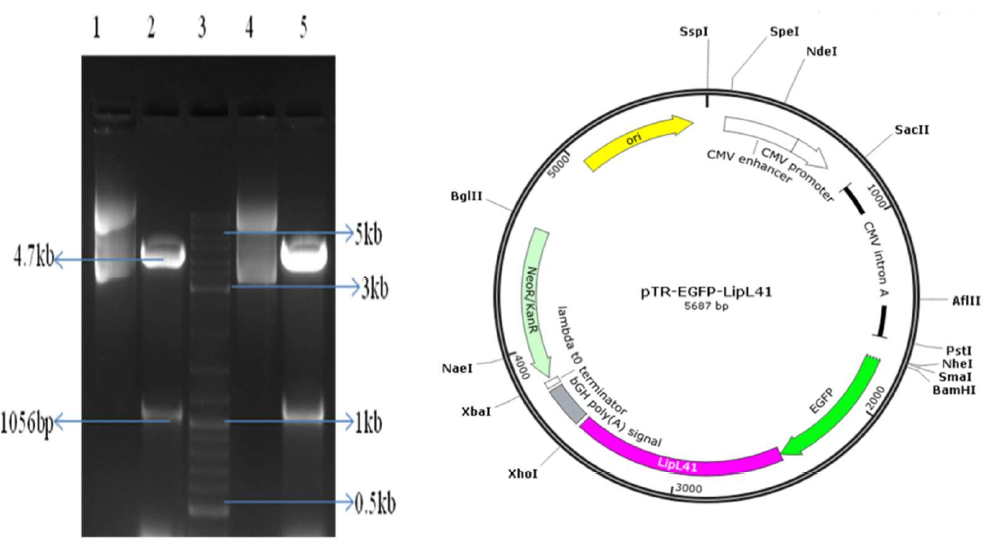
Article Details
Volume & Issue : Vol 11 No 9 (2024)
Page No.: 6775-6785
Published on: 2024-09-30
Citations
Copyrights & License

This work is licensed under a Creative Commons Attribution 4.0 International License.
Search Panel
Pubmed
Google Scholar
Pubmed
Google Scholar
Pubmed
Google Scholar
Pubmed
Google Scholar
Pubmed
Google Scholar
Pubmed
Search for this article in:
Google Scholar
Researchgate
- HTML viewed - 1814 times
- PDF downloaded - 691 times
- XML downloaded - 95 times