Abstract
Introduction: The aim of this study was to examine the antioxidant effects of one of the natural plant sources against oxidative stress caused by aging. Moringa oleifera seeds (MOS), the less utilized part, were chosen to investigate their role against oxidative stress in aging male albino rats.
Methods: DPPH (2,2-diphenyl-1-picryl-hydrazyl-hydrate), ABTS (2,2'-azino-bis (3-ethylbenzothiazoline-6-sulfonic acid)), polyphenols, some vitamins, and amino acids were estimated in the moringa seeds. The animals were mainly divided into two groups: adult and elderly rats. Each group was further subdivided equally into normal (not treated) and treated rats (which were orally administered an aqueous ground suspension of the MOS seeds) at a dose of 500 mg/kg body weight for four weeks (five days/week). Serum levels of free testosterone, free triiodothyronine (FT3), free thyroxine (FT4), and liver and kidney function were assessed. Additionally, histopathological investigations of brain and testicular tissue samples were conducted. Glutathione S-transferase (GST), malondialdehyde (MDA), and acetylcholinesterase (AChE) were measured in the homogenates of brain and testicular tissues.
Results: The results reveal a powerful antioxidant effect of MOS, indicated by a significant reduction in MDA levels along with a significant increase in GST and AChE concentrations. MOS treatment significantly increased serum testosterone levels and thyroid hormone levels in the male rats' serum.
Conclusion: MOS could improve most oxidative stress disorders associated with aging in male rats. Finally, the inadequacy of research on brain and testicular aging has been identified, and new research options have been proposed to aid in the treatment of brain and testicular aging. Further study in this area may uncover the underlying mechanisms, paving the way for the development of new treatments for age-related issues.
Introduction
The aging process is defined as a gradual, unavoidable loss of several body functions1. As age progresses, the brain undergoes biological, psychosocial, neuroanatomical, and neurophysiological changes that are all associated with a decline in cognitive function2. There is accumulating evidence that increasing male age has a considerable detrimental influence on spermatogenesis and fertilization3. The spermatogenesis microenvironment reveals major anomalies with age, such as reduced number, organelle aging, aberrant hormone production, and deficiencies of the blood–testicular barrier4. Aging is characterized by a gradual decrease in tissue and organ function. According to the oxidative stress theory of aging, the accumulation of harmful reactive oxygen and nitrogen free radicals leads to age-related functional impairments. In addition, oxidative stress contributes to a number of age-related illnesses, such as sarcopenia and frailty (e.g., cardiovascular diseases, obstructive pulmonary disease, diabetes, renal disease, neurodegenerative diseases, and cancer). Antioxidant defenses mitigate the detrimental effects of free radicals, which are produced by a range of internal and environmental processes. Oxidative stress is brought on by an imbalance between the production of free radicals and antioxidant defenses5. Phytochemical prevention of serious health conditions has gained global recognition, with studies on the anti-aging properties of plant extracts garnering substantial attention.
Moringa oleifera (MO) is a medicinal plant belonging to the family Moringaceae. The MOS has recently drawn a lot of interest because of its nutritional value and health advantages6. MOS (fresh, powdered, or cooked) includes a wide range of nutrients, such as significant sulfur amino acids, protein, and minerals7. Recently, bioactive peptides generated from natural plants have gained attention in the health and pharmaceutical industries8. According to Jain et al.9, MOS contains around 52% of all necessary amino acids, making it a possible source of functional protein isolate. Because of the harmonized amino acid content, MOS protein could be utilized instead of other sources of protein for human diets. MOS protein contains significant levels of cysteine and methionine (43.6 g/kg protein), which are comparable to chicken eggs, cow, and human milk10. MOS has displayed a plethora of bioactivities such as antioxidant11, anti-inflammatory12, antimicrobial13, antiviral14, liver-protective15, anti-diabetic16, antitumor17, and cardio-protective activities18.
Previous research has concentrated on the utilization of MOS and its protective mechanisms in various tissues rather than the brain. As a result, the current study employs MOS to assess its effect as an antioxidant against oxidative stress produced by aging, such as serum biochemical disturbance and brain and testicular tissue damage.
Methods
All materials were of analytical grade and were purchased from commercial stores.
Determination of DPPH
The free radical scavenging capacity of MOS was determined using the stable DPPH (2,2-diphenyl-1-picryl-hydrazyl-hydrate), according to Hwang and Do Thi19.
Radical ABTS Scavenging Activity Determination
ABTS (2,2'-azino-bis (3-ethylbenzothiazoline-6-sulfonic acid)) stock solutions were produced according to Hwang and Do Thi19.
High-Performance Liquid Chromatography (HPLC)
The phytochemical, amino acids, and vitamin B composition of MOS were analyzed using HPLC. Fifty milligrams of MOS was combined with 5 mL of H2O and 5 mL of HCl, heated at 100°C for 24 hours, and filtered. Finally, 1 mL of filtrate was dried, resuspended in 0.1 M HCl, and injected into the HPLC. HPLC analysis was performed using an Agilent 1260 series. The Eclipse C18 column (4.6 mm x 250 mm i.d., 5 μm) temperature was maintained at 40°C.
Preparation of MOS and Experimental Animal Groups
Dry Moringa oleifera seeds (MOS) were purchased from the laboratories of the National Research Centre, Giza, Egypt. MOS were washed and peeled. A mortar was used to grind and finely powder the seeds, which were then used as a suspension in distilled water. Twenty male Wistar albino rats were obtained from the animal house of the Nuclear Research Centre, Egyptian Atomic Energy Authority (EAEA). Before beginning the experiment, rats were kept in the Animal House of the Nuclear Research Centre's Radioisotopes Department for two weeks.
Rats were divided into four groups (five animals each): G1: five normal adult rats (2 months) without any treatment. G2: five normal adult rats (2 months) received orally 500 mg/Kg b.wt MOS (in a gradual increase of the dose from 100-500 mg/Kg b.wt during the first week) for 4 weeks (five days/week) according to El-Hak et al.20, with some modification. G3: five aged (14 months) male rats without any treatment. G4: five aged (14 months) male rats received orally 500 mg/Kg b.wt MOS as in G2.
Sample Collection
Twenty-four hours after the last administration of MOS, we anesthetized the rats and humanely decapitated them to collect samples. Blood samples were collected without anticoagulants and centrifuged for five minutes at 3000 rpm to separate the serum. The rats' brain and testicular tissues from each group were subsequently collected.
Liver and Kidney Function
Serum alanine aminotransferase (ALT), aspartate aminotransferase (AST) enzymes, albumin, urea, and serum creatinine were determined using assay kits obtained from Vitro Scient Co. (Cairo, Egypt).
Thyroid and Testosterone Determination by Radioimmunoassay
Serum FT3 (Cat. No. A13430), FT4 (Cat. No. 33880), and free testosterone (Cat. No. DSL4900) were determined using a commercial kit purchased from Beckman Coulter, Immunotech, Czech Republic.
Brain and Testicular Tissue Homogenate Preparation
The brain and testicular tissues of the rats in each group (10% wt/v) were promptly homogenized using a German IKA homogenizer. The tissue was cleansed with ice-cold PBS (0.01M, pH 7.4). Then, the homogenates were centrifuged at 5000 rpm for 15 minutes at 4°C with a Beckman Coulter Allegra 64R fixed-angle rotor (PN 392050).
Antioxidant Detection
Acetylcholine Esterase (AChE): AChE activity was measured according to Ellman et al.21.
Glutathione S-transferase (GST) Kinetic Assays: GST activities towards 1-chloro-2,4-dinitrobenzene (CDNB) were measured spectrophotometrically at 340 nm according to Habig et al.22.
Lipid Peroxidation: Lipid peroxidation (LPO) was assessed in terms of malondialdehyde (MDA) creation, according to the assay stated by Ohkawa et al.23. The MDA concentration was quantified as nmol MDA/mg protein.
Histopathological Examination
The brain and testicular tissue samples were collected in various groups and well-preserved in 10% formalin saline for one day. After washing with tap water, dehydration was accomplished using successive dilutions of alcohol (methyl, ethyl, and absolute ethyl). Specimens were cleaned in xylene and fixed in paraffin at 56°C in a hot air oven for one day according to Banchroft et al.24.
Statistical Analysis
Results are represented as mean values ± standard error. A parametric test was used to determine whether the data had a normal distribution, and thereafter, various assessments were statistically analyzed using one-way ANOVA tests, followed by Tukey's HSD multiple comparisons as a post-hoc test, to identify the significant differences between the various groups. A p-value of < 0.05 was considered statistically significant. The software SPSS statistical version 20 (SPSS® Inc., USA) was used for all statistical evaluations.
MOS (1g/15 ml) | |||
Compounds | Area | Concentration (µg/ml) | Concentration (µg/g) |
Gallic acid | 30.83 | 2.43 | 36.46 |
Chlorogenic acid | 28.42 | 4.30 | 64.52 |
Catechin | 1.49 | 0.35 | 5.28 |
Coffeic acid | 2.66 | 0.20 | 2.93 |
Syringic acid | 2.17 | 0.19 | 2.78 |
Pyro catechol | 16.38 | 2.38 | 35.69 |
Vanillin | 41.11 | 1.42 | 21.32 |
Cinnamic acid | 42.52 | 0.85 | 12.71 |
Kaempferol | 18.02 | 2.82 | 42.36 |
MOS (1g/15 ml) | ||
Area | Concentration (µg/g) | |
Vitamin B1 | 11.65 | 437.14 |
Vitamin B2 | 5.37 | 76.50 |
Vitamin B6 | 35.15 | 2693.42 |
Vitamin B9 | 3.40 | 244.52 |
Vitamin B12 | 12.60 | 1577.69 |
Compound | Area (Sample) | Concentration S (ng/g) | Concentration S (mg/g) |
ASP | 1426.98 | 10711189.79 | 10.71 |
GLU | 6742.52 | 60270978.95 | 60.27 |
Serine | 1632.96 | 8117751.79 | 8.12 |
Histidine | 441.34 | 5915776.01 | 5.92 |
Glycine | 3518.41 | 12872315.78 | 12.87 |
Threonine | 1051.08 | 5883521.42 | 5.88 |
Arginine | 5301.38 | 38192078.24 | 38.19 |
Alanine | 2513.66 | 10227841.74 | 10.23 |
Tyrosine | 461.20 | 3846887.14 | 3.85 |
Cystine | 4.24064 | 597574.11 | 0.60 |
Valine | 1817.97 | 9686963.72 | 9.69 |
Methionine | 1072.21 | 5130091.14 | 5.13 |
Phenylalanine | 1399.57 | 11036623.03 | 11.04 |
Isoleucine | 1719.54 | 8218485.13 | 8.22 |
Leucine | 2687.61 | 14500137.30 | 14.50 |
Lysine | 221.63 | 3599818.29 | 3.60 |
Proline | 454.56 | 10849480.01 | 10.85 |
Parameters | Groups | |||
G1: Adult control (N = 5) | G2: Adult+MOS (N = 5) | G3: Old control (N = 5) | G4: Old+MOS (N = 5) | |
AST (U/L) | 75.6 ± 1.02 | 72.8 ± 1.01 | 87.8 ± 2.8 | 78.2± 8 . 3 |
Tukey test | a | a | a | a |
ALT (U/L) | 25.2 ± 2.05 | 20.0 ± 0.54 | 40.4 ± 5.19 | 34.0±2.5 |
Tukey test | ab | a | c | bc |
Albumin (g/dL) | 3.0 ± 0.25 | 3.86 ± 0.13 | 2.54 ± 0.17 | 3.68±0. 20 |
Tukey test | ab | c | a | bc |
Total protein (g/dL) | 5.98 ± 0.18 | 6.50±0.266 | 4.72±0.33 | 6.90±0.25 |
Tukey test | a | |||
Urea (mg/dL) | 54.8 ± 2.13 | 46.5±1.6 | 60.2±1.8 | 48.96±1.92 |
Tukey test | bc | a | c | ab |
Creatinine (mg/dL) | 0.46 ± 0.02 | 0.34±0.02 | 0.74±0.05 | 0.50±0.044 |
Tukey test | ab | a | c | |
Free T3 (pg/mL) | 5.62 ± 0.36 | 8.07±0.7 | 3.66±0.1 | 6.38±0.47 |
Tukey test | c | a | bc | |
Free T4 (ng/dl) | 2.10 ± 0.02 | 3.6±0.31 | 2.0±0.03 | 3.1±0.2 |
Tukey test | a | ab | ||
Free testosterone (pg/ml) | 14.3 ± 0.6 | 19.6±1.2 | 13.2±0.6 | 18.7±0.7 |
Tukey test | a | a | ab |
Testicular | Brain | |||||
Oxidative marker Groups | GST (nmol/min/ mg protein) | AChE (µmol/min/ µg protein) | MDA (nmol/mg protein) | GST (nmol/ min/mg protein) | AChE (µmol/min/µg protein) | MDA (nmol/mg protein) |
G1 : Adult control | 176.4 ± 2.83 | 4.98 ± 0.31 | 158.6 ± 2.2 | 235 ± 8.94 | 5.92 ± 0.24 | 149.4 ± 1.63 |
Tukey test | ||||||
G2 : Adult+MOS | 251.2 ± 5.49 | 9.24 ± 0.12 | 147 ± 1.87 | 336 ± 9.28 | 10.82 ± 0.32 | 134 ± 1.78 |
Tukey test | d | d | a | c | d | a |
G3 : Old control | 97.0 ± 4.63 | 2.96 ± 0.166 | 209.4 ± 2.8 | 100 ± 20.7 | 4.12 ± 0.21 | 187.4 ± 1.63 |
Tukey test | a | a | d | a | a | d |
G4 : Old+MOS | 215.8 ± 3.89 | 6.84 ± 0.172 | 183.8 ± 2.3 | 288.2 ± 4.8 | 7.88 ± 0.32 | 170.2 ± 2.57 |
Tukey test | c | c | c | c | c | c |
Organ | Histopathological alterations | G1: Adult control | G2: Adult+MOS | G3: Old control | G4: Old+MOS |
Testes | Degenerated seminiferous tubules | + | - | +++ | - |
Brain | nuclear pyknosis and neuronal degeneration | + | - | + | - |
Results
DPPH and ABTS
The in vitro results indicated that DPPH scavenging activity had a value of 0.395 mg trolox/g, and the radical scavenging activities of ABTS were 0.802 mg trolox/g.
HPLC
The phytochemical, amino acids, and vitamin B of MOS were estimated using HPLC. The individual phenolic and flavonoid compounds results showed that chlorogenic acid and kaempferol had a strong response and more intense peaks (Table 1 and Figure 1). The vitamin B content in MOS was estimated using HPLC. The results showed that vitamins B6 (2693.42 µg/g) and B12 (1577.69 µg/g) had the highest concentration, as shown in Table 2 and Figure 2. The amino acid content in MOS is shown in Table 3 and Figure 3.
In vivo results
Toxicity test of MOS
Acute oral administration of MOS up to 500 mg/kg resulted in no adverse effects in the experimental animals, including food intake, atypical body growth, decreased activity, diarrhea, bleeding, or mortality. As a result, no fatal dosage was established in this study.
Liver and kidney function
The results indicated that aging induced moderate changes in the biochemical parameters and an imbalance of oxidants and antioxidants in the male rats. There was a significant increase in serum urea, ALT, and AST levels in the aged rats compared to the control group. Treatment of rats with MOS significantly (P < 0.05) reduced urea levels in the serum of aged rats (Table 4). Furthermore, data showed that ALT, creatinine, and albumin levels were lower in MOS-treated rats than in control adult rats.
Effect of the MOS on testosterone and thyroid hormones
Aging showed a significant decrease in FT3, FT4, and free testosterone (Table 4). MOS treatment significantly increased serum testosterone levels and thyroid hormones in male rats.
Effect of the MOS on oxidative status
The results indicated that aging induced an imbalance of oxidants and antioxidants in male rats. Aging showed a significant increase in lipid peroxidation in testicular and brain tissues (Table 5). Treatment of rats with MOS significantly (P < 0.05) diminished the raised values of MDA in the aged group (Table 5). The abnormally declined activities of GST and AChE enzymes significantly increased in the old group administered MOS when compared with control. The administration of MOS dramatically increased GST and AChE levels compared to untreated rats.
Histopathological findings
The histopathological investigation of the testicular and brain tissue of different groups is provided in Figure 4 and Figure 5, respectively. Table 6 illustrates the histopathological changes in the testes and brains of rats administered moringa or not, based on the scoring severity of injury.
Discussion
The worldwide issue of population aging is becoming increasingly problematic as the global economy and medical care expand. Age-related disorders are affecting an increasing number of senior individuals25. Consequently, the demand for natural plant-based treatments that prevent and treat age-related illnesses is growing. This study employs MOS to assess its effects as an antioxidant and anti-aging agent against oxidative stress caused by aging, such as brain and testicular damage.
The phytochemical composition, vitamin B content, and amino acids of MOS used in this study are presented in Table 1, Table 2 and Table 3. MOS has been shown to contain bioactive secondary metabolites such as flavonoids (catechin, kaempferol, vanillin), with the most abundant being kaempferol (42.36 µg/g), and phenolic acids (ellagic acid, gallic acid, ferulic acid, chlorogenic acid), with the most abundant being chlorogenic acid (64.52 µg/g). Manisha et al.26 demonstrated that MO leaves contained flavonoids, mainly quercetin and kaempferol, with concentrations of up to 137.81 and 106.75 mg/g, respectively, and phenolic acids, which include ferulic acid, ellagic acid, and chlorogenic acid.
Infertility affects 50-80 million people worldwide, with males accounting for 20-50% of cases27. In the present study, the effect of MOS in enhancing male reproduction is clearly observed in the aged group compared to the control. The antioxidants in MOS worked in tandem with the epididymis's antioxidant system to further protect and improve the process of spermatogenesis. Aging may be associated with a rise in endogenous ROS, which reduces antioxidant enzyme activity, with Leydig cells being particularly vulnerable to this impact. High ROS levels can alter the hormonal balance that governs male reproductive processes by acting on the hypothalamic-pituitary-gonadotropic (HPG) axis28. Reduced LH secretion causes Leydig cells to generate insufficient testosterone29, resulting in uncontrolled spermatogenesis and suppression of sexual behavior30. In spermatozoa, MDA molecules enter the cell membrane structure, disrupting the symmetric distribution of lipid membrane components. Lipid peroxidation destroys the central region of the sperm cell, resulting in a loss of acrosome ability for fertilization31. Moringa leaves might have beneficial effects on improving male sexual performance in stress-induced sexual dysfunction in rats32. Zade et al.33 investigated the effects of the aqueous seed extract on the frequency of mounting and intromission in male rats. The investigators concluded that MOS may improve male sexual behavior because it also significantly boosts desire and sperm count. MO has been used to treat male sexual functions such as desire, erectile dysfunction, and testicular damage34. After oral administration of MO, aged rats (18-19 months old) showed improvements in sperm count and morphology, indicating its potential utility in the treatment of sperm abnormalities35. Furthermore, MO is a preventative approach for a variety of ailments and disorders, such as testosterone-induced benign prostatic hyperplasia36.
This study provides findings on the role of MOS on brain cholinergic enzyme (AChE) and MDA. The G2 and G4 groups (MOS administration) presented a significant drop in MDA level and an increase in GST and AChE activities when compared to control groups. MOS contains high phenol and flavonoid content, which are crucial oxidative components in plants. Their antioxidant activity is attributed to their redox characteristics, which help scavenge and neutralize free radicals. MO is utilized to treat neurodegenerative illnesses, including Alzheimer's, ischemic stroke, and epilepsy37. Aging of the brain can cause memory and cognitive impairment, which is commonly associated with changes in the structural flexibility of dendritic spines. In elderly animals and humans, spine number and maturity are reduced, along with alterations in synaptic transmission, which may represent abnormal neuronal plasticity intimately related to reduced brain function. In the most severe cases, a neurodegenerative illness that entirely destroys the basic processes of brain development is possible38.
This study concluded that MOS improves the role of the thyroid by raising thyroid hormones (FT3 and FT4) and reducing oxidative stress in aged male rats when compared to adults. Tahiliani and Kar39 revealed that MO leaf extract has therapeutic efficacy in managing hyperthyroidism, an autoimmune condition, by suppressing T3 production and release.
Our results showed that MOS treatment led to improvements in AST and ALT levels, suggesting a hepatoprotective effect. A modest dose of M. seeds (500 mg/kg B.W.) was more effective at lowering ALT, although larger doses (1000 & 2000 mg/kg B.W.) produced the lowest level when compared to the control group20.
This study concluded that MOS treatment led to improvements in urea and creatinine levels, suggesting a renal protective effect. MO reduces inflammation and oxidative stress, which are common causes of kidney disease40.
Conclusions
From the current study, it is clear that MOS can increase sexual behavior in male rats and could potentially have an impact depending on particular circumstances. MOS exhibited a significant decrease in the MDA level and an increase in GST and AChE activities in brain tissue. Additionally, MOS improves the role of the thyroid by raising the thyroid hormones FT3 and FT4. These findings highlight the crucial role of wild MOS bioactive components in brain, thyroid, and testicular function. Further study in this area may uncover the underlying mechanisms, paving the way for the development of new treatments for age-related issues.
Abbreviations
ABTS: 2,2'-azino-bis (3-ethylbenzothiazoline-6-sulfonic acid), AChE: Acetylcholinesterase, ALT: Alanine aminotransferase, AST: Aspartate aminotransferase, DPPH: 2,2-diphenyl-1-picryl-hydrazyl-hydrate, EAEA: Egyptian Atomic Energy Authority, FT3: Free triiodothyronine, FT4: Free thyroxine, GST: Glutathione S-transferase, HPG Axis: Hypothalamic-pituitary-gonadotropic axis, HPLC: High-Performance Liquid Chromatography, LH: Luteinizing hormone, LPO: Lipid peroxidation, MDA: Malondialdehyde, MO: Moringa oleifera, MOS: Moringa oleifera seeds, ROS: Reactive oxygen species
Acknowledgments
The authors wish to extend their heartfelt appreciation to Prof. Dr. Adel Bakeer, an esteemed Professor of Pathology at the Faculty of Veterinary Medicine, University of Cairo, for his invaluable support throughout the histopathological examination.
Author’s contributions
Noha Sayed Hamed: Writing – review & editing, Writing – original draft, Visualization, Validation, Methodology, Formal analysis, Data curation, conducted the experiments, Conceptualization. Hoda Badr Hammad: Editing, Validation, Data curation. Mona Ibrahim Abdou: Writing – review & editing, Formal analysis, Data curation, Conceptualization. All authors read and approved the final manuscript.
Funding
None.
Availability of data and materials
Data and materials used and/or analyzed during the current study are available from the corresponding author on reasonable request.
Ethics approval
Ethical approval was sought and obtained from the Ethical Committee at the National Center for Radiation Research and Technology, EAEA (NCRRT- EAEA-12A/20).
Consent for publication
Not applicable.
Competing interests
The authors declare that they have no competing interests.
References
-
Leal
S.L.,
Yassa
M.A.,
Neurocognitive aging and the hippocampus across species. Trends in Neurosciences.
2015;
38
(12)
:
800-12
.
View Article PubMed Google Scholar -
Glisky
E.L.,
Changes in Cognitive Function in Human Aging. In: Riddle DR, editor. Brain Aging: Models, Methods, and Mechanisms. Boca Raton (FL): CRC Press/Taylor & Francis, Chapter 1(2007). https://www.ncbi.nlm.nih.gov/books/NBK3885/. 2007
.
-
Khandwala
Y.S.,
Baker
V.L.,
Shaw
G.M.,
Stevenson
D.K.,
Lu
Y.,
Eisenberg
M.L.,
Association of paternal age with perinatal outcomes between 2007 and 2016 in the United States: population based cohort study. BMJ (Clinical Research Ed.).
2018;
363
:
k4372
.
View Article PubMed Google Scholar -
Santiago
J.,
Silva
J.V.,
Alves
M.G.,
Oliveira
P.F.,
Fardilha
M.,
Testicular aging: an overview of ultrastructural, cellular, and molecular alterations. The Journals of Gerontology. Series A, Biological Sciences and Medical Sciences.
2019;
74
(6)
:
860-71
.
View Article PubMed Google Scholar -
Hajam
Y.A.,
Rani
R.,
Ganie
S.Y.,
Sheikh
T.A.,
Javaid
D.,
Qadri
S.S.,
Oxidative Stress in Human Pathology and Aging: Molecular Mechanisms and Perspectives. Cells.
2022;
11
(3)
:
552
.
View Article PubMed Google Scholar -
Liao
M.,
Sun
C.,
Li
R.,
Li
W.,
Ge
Z.,
Adu-Frimpong
M.,
Xu
X.,
Yu
J.,
Amelioration action of gastrodigenin rhamno-pyranoside from Moringa seeds on non-alcoholic fatty liver disease. Food Chemistry.
2022;
379
:
132087
.
View Article Google Scholar -
Maryann
C.U.,
Lord
A.,
Uchechukwu
N.,
Chibuike
C.U.,
Potential of Moringa oleifera seeds and leaves as functional food ingredients for human health promotion. Journal of Food and Nutrition Research.
2018;
57
(1)
:
1-14
.
-
Görgüç
A.,
Gençdağ
E.,
Y\ilmaz
F.M.,
Bioactive peptides derived from plant origin by-products: biological activities and techno-functional utilizations in food developments - A review. Food Research International.
2020;
136
:
109504
.
View Article PubMed Google Scholar -
Jain
A.,
Subramanian
R.,
Manohar
B.,
Radha
C.,
Preparation, characterization and functional properties of Moringa oleifera seed protein isolate. Journal of Food Science and Technology.
2019;
56
(4)
:
2093-104
.
View Article PubMed Google Scholar -
Owon
M.,
Osman
M.,
Ibrahim
A.,
Salama
M.A.,
Matthäus
B.,
Characterisation of different parts from Moringa oleifera regarding protein, lipid composition and extractable phenolic compounds. Oilseeds and Fats, Crops and Lipids.
2021;
28
:
45
.
View Article Google Scholar -
Gu
X.,
Yang
Y.,
Wang
Z.,
Nutritional, phytochemical, antioxidant, α-glucosidase and α-amylase inhibitory properties of Moringa oleifera seeds. South African Journal of Botany.
2020;
133
:
151-60
.
View Article Google Scholar -
Ziani
B.E.,
Rached
W.,
Bachari
K.,
Alves
M.J.,
Calhelha
R.C.,
Barros
L.,
Detailed chemical composition and functional properties of Ammodaucus leucotrichus Cross. & Dur. and Moringa oleifera Lamarck. Journal of Functional Foods.
2019;
53
:
237-47
.
View Article Google Scholar -
Mehwish
H.M.,
Rajoka
M.S.,
Xiong
Y.,
Cai
H.,
Aadil
R.M.,
Mahmood
Q.,
Green synthesis of a silver nanoparticle using Moringa oleifera seed and its applications for antimicrobial and sun-light mediated photocatalytic water detoxification. Journal of Environmental Chemical Engineering.
2021;
9
(4)
:
105290
.
View Article Google Scholar -
Xiong
Y.,
Rajoka
M.S.,
Mehwish
H.M.,
Zhang
M.,
Liang
N.,
Li
C.,
Virucidal activity of Moringa A from Moringa oleifera seeds against Influenza A Viruses by regulating TFEB. International Immunopharmacology.
2021;
95
:
107561
.
View Article PubMed Google Scholar -
Liang
L.L.,
Cai
S.Y.,
Gao
M.,
Chu
X.M.,
Pan
X.Y.,
Gong
K.K.,
Purification of antioxidant peptides of Moringa oleifera seeds and their protective effects on H2O2 oxidative damaged Chang liver cells. Journal of Functional Foods.
2020;
64
:
64
.
View Article Google Scholar -
Olusola
A. O.,
Ekun
E. O.,
Moringa oleifera seed protein hydrolysates inhibit haemoglobin glycosylation and α-glucosidase activity in-vitro. Global Journal of Medical Research.
2019;
19
:
3–B
.
-
Abou-Hashem
M.M.,
Abo-Elmatty
D.M.,
Mesbah
N.M.,
Abd El-Mawgoud
A.M.,
Induction of sub-G0 arrest and apoptosis by seed extract of Moringa peregrina (Forssk.) Fiori in cervical and prostate cancer cell lines. Journal of Integrative Medicine.
2019;
17
(6)
:
410-22
.
View Article PubMed Google Scholar -
Aderinola
T.A.,
Fagbemi
T.N.,
Enujiugha
V.N.,
Alashi
A.M.,
Aluko
R.E.,
Amino acid composition and antioxidant properties of Moringa oleifera seed protein isolate and enzymatic hydrolysates. Heliyon.
2018;
4
(10)
:
e00877
.
View Article PubMed Google Scholar -
Hwang
E.S.,
Thi
N.D.,
Effects of Extraction and Processing Methods on Antioxidant Compound Contents and Radical Scavenging Activities of Laver (Porphyra tenera). Preventive Nutrition and Food Science.
2014;
19
(1)
:
40-8
.
View Article PubMed Google Scholar -
El-Hak
H.N.,
Moustafa
A.R.,
Mansour
S.R.,
Toxic effect of Moringa peregrina seeds on histological and biochemical analyses of adult male Albino rats. Toxicology Reports.
2017;
5
:
38-45
.
View Article PubMed Google Scholar -
Ellman
G.L.,
Courtney
K.D.,
Andres
V.,
Feather-Stone
R.M.,
A new and rapid colorimetric determination of acetylcholinesterase activity. Biochemical Pharmacology.
1961;
7
(2)
:
88-95
.
View Article PubMed Google Scholar -
Habig
W.H.,
Pabst
M.J.,
Jakoby
W.B.,
Glutathione S-transferases. The first enzymatic step in mercapturic acid formation. The Journal of Biological Chemistry.
1974;
249
(22)
:
7130-9
.
View Article PubMed Google Scholar -
Ohkawa
H.,
Ohishi
N.,
Yagi
K.,
Assay for lipid peroxides in animal tissues by thiobarbituric acid reaction. Analytical Biochemistry.
1979;
95
(2)
:
351-8
.
View Article PubMed Google Scholar -
Banchroft
J.D.,
Stevens
A.,
Turner
D.R.,
Theory and practice of histological techniques. Fourth Ed. Churchil Livingstone, New York, London, San Francisco, Tokyo (1996)..
.
-
Ismail
Z.,
Ahmad
W.I.,
Hamjah
S.H.,
Astina
I.K.,
The Impact of Population Ageing: A Review. Iranian Journal of Public Health.
2021;
50
(12)
:
2451-60
.
View Article PubMed Google Scholar -
Manisha
N.,
Rajak
R.,
Jat
D.,
Oxidative stress and antioxidants: an overview. International Journal of Advanced Research and Review..
2017;
2
:
110-9
.
-
Mohlala
K.,
Offor
U.,
Monageng
E.,
Takalani
N.B.,
Opuwari
C.S.,
Overview of the Effects of Moringa oleifera Leaf Extract on Oxidative Stress and Male Infertility: A Review. Applied Sciences (Basel, Switzerland).
2023;
13
(7)
:
4387
.
View Article Google Scholar -
Spiers
J.G.,
Chen
H.J.,
Sernia
C.,
Lavidis
N.A.,
Activation of the hypothalamic-pituitary-adrenal stress axis induces cellular oxidative stress. Frontiers in Neuroscience.
2015;
8
:
456
.
View Article PubMed Google Scholar -
Greifová
H.,
Jambor
T.,
Tokárová
K.,
Speváková
I.,
Kní\vzatová
N.,
Luká\vc
N.,
Resveratrol attenuates hydrogen peroxide-induced oxidative stress in TM3 Leydig cells in vitro. Journal of Environmental Science and Health. Part A, Toxic/Hazardous Substances {&}amp; Environmental Engineering.
2020;
55
(5)
:
585-95
.
View Article PubMed Google Scholar -
Darbandi
M.,
Darbandi
S.,
Agarwal
A.,
Sengupta
P.,
Durairajanayagam
D.,
Henkel
R.,
Reactive oxygen species and male reproductive hormones. Reproductive Biology and Endocrinology.
2018;
16
(1)
:
87
.
View Article PubMed Google Scholar -
Asadi
N.,
Bahmani
M.,
Kheradmand
A.,
Rafieian-Kopaei
M.,
The Impact of Oxidative Stress on Testicular Function and the Role of Antioxidants in Improving it: A Review. Journal of Clinical and Diagnostic Research : JCDR.
2017;
11
(5)
:
01-05
.
View Article PubMed Google Scholar -
Prabsattroo
T.,
Wattanathorn
J.,
Iamsaard
S.,
Somsapt
P.,
Sritragool
O.,
Thukhummee
W.,
Moringa oleifera extract enhances sexual performance in stressed rats. Journal of Zhejiang University. Science. B..
2015;
16
(3)
:
179-90
.
View Article PubMed Google Scholar -
Zade
S.V.,
Dabhadkar
K.D.,
Thakare
G.V.,
Pare
R.S.,
Effect of Aqueous Extract of Moringa oleifera Seed on Sexual Activity of Male Albino Rats. Biological Forum : An International Journal.
2013;
5
(1)
:
129-40
.
-
Syarifuddin
N.,
Toleng
A.,
Rahardja
D.,
Ismartoyo
I.,
Yusuf
M.,
Improving Libido and Sperm Quality of Bali Bulls by Supplementation of Moringa oleifera Leaves. Media Peternakan.
2017;
40
(2)
:
88-93
.
View Article Google Scholar -
Widiastini
L.P.,
Karuniadi
I.G.,
Tangkas
M.,
Ethanol Extract of Moringa oleifera Increased the Number of Spermatozoa and Improved Sperm Morphology of Old Rattus norvegicus. Jurnal Bioteknologi & Biosains Indonesia..
2022;
9
:
11-9
.
-
Mohamed
M.A.,
Ahmed
M.A.,
El Sayed
R.A.,
Molecular effects of Moringa leaf extract on insulin resistance and reproductive function in hyperinsulinemic male rats. Journal of Diabetes and Metabolic Disorders.
2019;
18
(2)
:
487-94
.
View Article PubMed Google Scholar -
Azlan
U.K.,
Annuar
N.A. Khairul,
Mediani
A.,
Aizat
W.M.,
Damanhuri
H.A.,
Tong
X.,
An insight into the neuroprotective and anti-neuroinflammatory effects and mechanisms of Moringa oleifera. Frontiers in Pharmacology.
2023;
13
:
1035220
.
View Article PubMed Google Scholar -
Sikora
E.,
Bielak-Zmijewska
A.,
Dudkowska
M.,
Krzystyniak
A.,
Mosieniak
G.,
Wesierska
M.,
Cellular Senescence in Brain Aging. Frontiers in Aging Neuroscience.
2021;
13
:
646924
.
View Article PubMed Google Scholar -
Tahiliani
P.,
Kar
A.,
Role of Moringa oleifera leaf extract in the regulation of thyroid hormone status in adult male and female rats. Pharmacological Research.
2000;
41
(3)
:
319-23
.
View Article PubMed Google Scholar -
Akter
T.,
Rahman
M.A.,
Moni
A.,
Apu
M.A.,
Fariha
A.,
Hannan
M.A.,
Prospects for Protective Potential of Moringa oleifera against Kidney Diseases. Plants.
2021;
10
(12)
:
2818
.
View Article PubMed Google Scholar
Comments
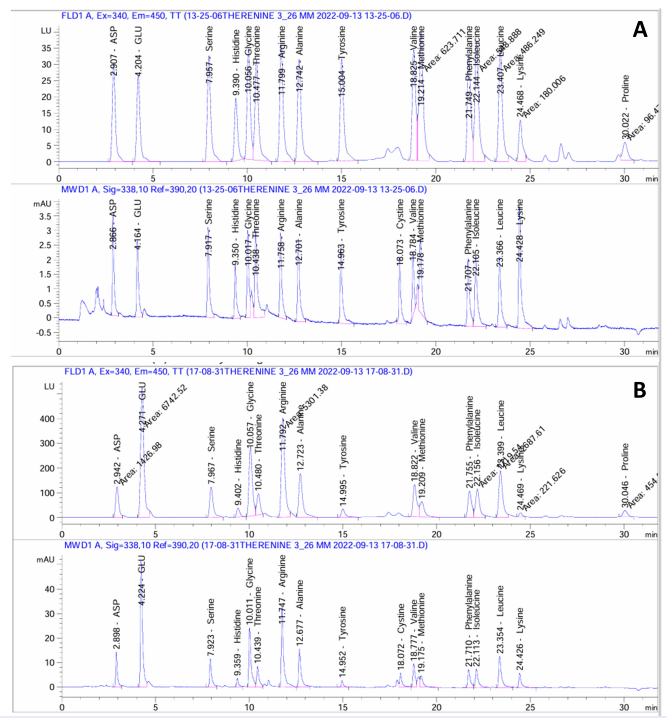
Article Details
Volume & Issue : Vol 11 No 10 (2024)
Page No.: 6813-6824
Published on: 2024-10-31
Citations
Copyrights & License

This work is licensed under a Creative Commons Attribution 4.0 International License.
Search Panel
Pubmed
Google Scholar
Pubmed
Google Scholar
Pubmed
Search for this article in:
Google Scholar
Researchgate
- HTML viewed - 188 times
- PDF downloaded - 104 times
- XML downloaded - 20 times