Effects of ricin extracted from seeds of the castor bean (ricinus communis) on cytotoxicity and tumorigenesis of melanoma cells
Abstract
Ricin has been reported as a potential therapeutic agent for the treatment of various cancers due to its potency. In this study, we succeeded in isolating and purifying total ricin from seeds of the castor bean (ricinus communis) from Vietnam. We also revealed that total ricin showed strong cytotoxicity against melanoma cells; IC50 at 48 h was 34.1 ng/mL for SKMEL28 cells and 5.2 ng/mL for HaCaT cells. We examined the ability of total ricin to inhibit tumorigenesis of SKMEL28 cells in vitro. At low concentrations (< 3ng/mL) total ricin did not cause death of HaCaT or SKMEL28 cells but strongly reduced the size of SKMEL28 tumor colonies formed in soft agar. The effect of ricin on tumorigenesis was also confirmed by apoptotic and immunoblot analyses. Our results showed that treatment with total ricin (3 ng/mL) resulted in decreased ERK and p-ERK expression in SKME28 cells but did not affect expression levels of those genes in HaCaT cells. We showed that, although ricin at 1 and 3 ng/mL did not induce apoptosis of HaCaT cells, it significantly increased apoptosis of SKMEL28 up to 1.4 folds and 2.1 folds, respectively. The results from our study suggest that although ricin is listed as one of the most poisonous substances in nature, it has potential to be used as a drug for melanoma treatment.
Introduction
While malignant melanoma accounts for less than 5% of all skin cancers, it is responsible for 80% of skin cancer deaths Gray-Schopfer et al., 2007. Outlook for patients with metastatic melanoma remains quite bleak, with a 5-year survival rate of only 5-15%. The rate has not changed significantly in recent decades despite intensive efforts to develop more effective therapies Gray-Schopfer et al., 2007. Since melanoma is aggressive cancer with high metastatic ability, the increase in incidence is a threat to public health. It is therefore important to find new effective therapies.
Extracts derived from natural sources for cancer treatments are being increasingly studied because of their advantages, such as low price and availability. There are many natural compounds applied in treating various types of cancers,including melanoma. Previous studies have shown that natural compounds extracted from medicinal plants are capable of inhibiting cancer properties of melanoma cells by reducing the expression of ERK and/or AKT Fagone et al., 2011Osmond et al., 2013Rebecca et al., 2014Shin et al., 2008.
Ricin, extracted from seeds of the castor bean, is a glycoprotein of the lectin family Jang and Kim, 1993Kumar et al., 2004. Castor bean seeds contain up to 50% oil, 25% albuminoside, and other compounds, including ricin, ricinin, malic acid, and saccharide Jang and Kim, 1993Kumar et al., 2004. Ricin consists of two polypeptide chains (A chain: 30 kDa and B chain: 34 kDa), which link with each other via disulfide bridges (-S-S-) Funatsu et al., 1976, and the molecular weight of ricin is about 64 kDa
Ricin is one of the most poisonous substances in nature and highly toxic in humans and animals Funatsu et al., 1976Lord et al., 1994. When this toxic protein enters a cell, particularly via inhalation or ingestion, it blocks the ability of cells to synthesize its own proteins, leading to cellular and organ damage. Due to its toxicity, ricin can be used for bioterrorism. Ricin can bind with high affinity to galactose residues on the cell surface and is transported through the cell membranes to cause cell death Funatsu et al.,1976Kumar et al., 2004Lord et al., 1994.Although both chains contribute to toxicity, the A chain is associated with higher poisonous property and has been more studied. The A chain of ricin has been reported to be a strong immunotoxin for cells Engert et al., 1994Engert et al., 1990Weidle et al., 2014.
Beside its toxic effects, ricin is also considered to have potential benefit in the treatment of a variety of cancers, including leukemia, breast cancer, cervical cancer and melanoma Ghosh et al., 2013LoRusso et al., 1995Spitler, 1986Spitler et al., 1987Tyagi et al., 2015. Ricin A chain (but not ricin B chain and/or total ricin), as an immunotoxin conjugated with antibody, has been evaluated for melanoma treatment Ghosh et al., 2013LoRusso et al., 1995Spitler, 1986Spitler et al., 1987Tyagi et al.,2015. In recent years, the application of nanotechnology, such as the use of robust nanoparticle carriers for drug delivery to target cancer cells, has been rapidly developing. There are increasingly more investigations on the role of ricin as anticancer agent encapsulated into a polymer and/or liposome carrier, and used as nano drugs for the treatment of specific target cancers Epler et al., 2012Ghosh et al., 2013Tyagi et al., 2015.
In this study, we focused on extracting, isolating and purifying total ricin from castor bean seeds and investigated the effects of the purified ricin on cytotoxicity and tumorigenesis of human melanoma cells in vitro.
Materials and methods
Castor bean seeds
Castor bean seeds were collected from Vietnam Pharmacy Institute in September 2013 and identified at the Department of Biochemistry and Plant physiology, Faculty of Biology, VNU University of Science, Vietnam National University, Hanoi, Vietnam. The castor bean seed specimen is depositing at the Biological Museum of VNU University of Science and the purified ricin is depositing at the Institute of Chemistry and Environment, High Command of Chemistry, Ministry of National Defense. We state that this purified ricin will be used for the research purposes in the laboratory only.
Preparation of seed extract
Castor bean seeds were cleaned and washed thoroughly with water and re-washed with distilled water. Washed seeds pressed under high pressure to remove oil. The press cake was then dried and refluxed in acetic acid 5% with 1/10 (w/v) for 12hrs to extract proteins. The extract was filtered, and the solvent was removed under reduced pressure at 40±5 °C using a rotary flash evaporator. The scheme of the extraction procedure was presented in the Figure 1 and the effectiveness of extraction procedure was presented in the Table 1
Protein precipitation
Ammonium sulfate with different concentrations wasadded into acetic acid extract solution to precipitate total protein. The examined concentrations of ammonium sulphate were 30, 40, 50, 60 and 70%. The extraction efficiencies were showed in Table 1 .
Protein separation
Crude protein got in precipitation step was applied for protein separation by ion exchange using DEAEsepharose (Amersham Biosciences). DEAE was equilibrated in 0.05M Tris-HCl buffer at pH 8.0 to create the DEAE gel. Dissolving of 20 mL crude protein solution in 50 mL DEAE gel, and stirring for 15 minutes for adsorbing of protein on the gel. Loading the protein binding gel on glass column (1.5 cmx40 cm) then washing with 100mL Tris-HCl buffer 0.05 M. Standing 30 minutes for stability before desorbing by 0.05M Tris-HCl buffer at pH 8.0 with supplementing of NaCl 0.05 M. Desorbing rate was set at 0.2 mL/minute. Protein fractions were collected with 2 mL for each.Ricin in each fraction was tested by quick test kit of Osborn, United State.
Protein purification
Ricin was purified by gel filtration used gel sephadex G-100 (Amersham Biosciences). Firstly, gel was activated in distilled water at room temperature (1g/10mL H20) for 6 hrs. Then, gel was loaded on glass column (2.5 cmx50 cm) and soaked phosphate buffer 0.002 M at pH 7.2 with ratio of protein solution/gel = 1:6 (v/v). Protein fractions were eluted with phosphate buffer 0.002 M at rate of 0.2 mL/minute. Protein fractions were collected with 2 mL for each fraction. Ricin in each fraction was tested by quick test kit of Osborn, United State. Standards of ricin (A chain and B chain) were purchased from Vectorlabs, USA by High Command of Chemistry, Ministry of National Defense for research purposes.
Mass spectronic (MS/MS) analysis
Purified protein was sent to Mission Biotech Co., LTD., Taipei, Taiwan for ricin qualitative analysis.
Cell culture
The human transformed normal skin keratinocyte (HaCaT) cells and malignant melanoma SKMEL28 cells were used. HaCaT cell wasa gift fromProf. Dr. Masashi Kato, School of Medicine, Nagoya University and SKMEL28 cells were purchased from ATCC, United State. All cell lines were cultured in RPMI supplemented with penicillin (400 U/mL), streptomycin (50 mg/mL), L-glutamine (300 mg/mL) and 10% fetal bovine serum (FBS; Sigma, Deisenhofen, Germany) under a humidified atmosphere of 5% CO2 at 37°C.
Cellular toxicity assay
A crystal violet assay was performed by the method described in the previous report Yajima et al., 2012to primarily assess the viability of cells. Briefly, 3x104 cells were plated in six-well plates and cultured in the medium with or without ricin for 48hrs. The viable adherent cells were fixed with 10% formalin for 1 hour and stained with 0.1% crystal violet for 30 minutes after washing two times with PBS. The stained cells were solubilized with 0.1% SDS, and the absorbance of the solution was measured at 595 nm by a spectrophotometer.
In vitro tumorigenesis assay
A colony formation assay was performed to assess the development of tumor in vitro. Anchorageindependent growth was evaluated by the colony formation assay according to the method reported in the previous study Thang et al., 2011. After preincubating in the medium for 48 hours, 2.5×104 cells were mixed with 2 mL of 0.36% soft agar in RPMI medium, poured onto slightly solid 0.72% hard agar in RPMI medium, and then cultured for 4 weeks. Colonies exceeding 50 μm in diameter were counted and presented as an activity of anchorageindependent growth.
Immunoblotting
Immunoblotting was performed to investigate the protein expression of molecules using the method described in the previous report Kato et al., 2010. Briefly, cells were washed twice with ice-cold PBS and lysed in 0.3 mL of lysis buffer (20 mM HEPES [pH 7.4], 150 mM NaCl, 12.5 mM b-glycerophosphate, 1.5 mM MgCl2, 2 mM EDTA, 10 mM NaF, 2 mM DTT, 1 mM Na3VO4, 1 mM phenylmethylsulfonyl fluoride, 20 mM aprotinin, 0.5% Triton X-100). Whole cell lysates or/and purified ricin protein were resolved on SDSPAGE and transferred to nitrocellulose Hybond-P membranes (GE Health Sciences). After protein transfer, nitrocellulose sheets were blocked at room temperature for 60 mins with skimmed milk powder (5%) in PBS and incubated for 120 mins with different antibodies at 1:1000dilutions.
The bound antibodies on the treated membranes were visualized with horseradish peroxidase-conjugated antibodies against rabbit or mouse IgG (Calbiochem) at 1:2000 dilutions for 90 mins at room temperature. The nitrocellulose sheets were washed three times for 5 mins with phosphate buffer saline-Tween 20 (PBST) and developed with 3,3'-diaminobenzidine. Antibodies against ricin (Agrisera – USA), ERK, p- ERK, and Tubulin (Thermo scientific) were used.
Annexin V apoptosis assay
Annexin V apoptosis assay was performed to investigate the protein expression of molecules using the method described by Schutte (1998). Cells were seeded at 30 to 40% confluence in 6-cm plates. After overnight incubation, the medium was aspirated and replaced with medium with or without ricin. After 36 hours, the medium was collected. Cells were washed with PBS. Collected cells were resuspended in annexin binding buffer (Life technology) at 1×106 cells/ml. Cells were stained with propidium iodide (Invitrogen) and annexin V-FITC according to the manufacturer’s protocol and assayed on a FACSCanto II (BD Biosciences). The percentage of apoptotic cells was measured as the percentage of annexin V-positive cells.
Statistical analysis
Statistical analysis in this study was performed according to the method described in the previous report Yajima et al., 2012. Results from three independent experiments in each group were statistically analyzed by Student’s t-test. The SPSS (version 18) software package (SPSS Japan Inc.) was used for statistical analyses, and the significance level was set at p < 0.05.
Results
Isolation of ricin from castor bean seeds
Ricin was isolated and purified using a 4-step procedure presented in Figure 1. Firstly, castor bean seeds were pressed to eliminate the oil. The press cake was dried in an oven at 40°C. Then, 100 gram of dried press cake was extracted in 1000 mL acetic acid 5% (1/10 v/v) at room temperature for 12 h with shaking at 400 revolutions/minute. After extraction, the solution was filtered through a filter paper to collect the crude protein solution (called solution I). The dried weight of crude protein extracted in acetic acid was 2.25 mg/mL ( Table 1 ). To precipitate the total protein, ammonium sulfate was applied. After elimination of ammonium sulfate, the harvested protein was measured. Per the literature, the optimum concentration of the ammonium sulfate solution used for protein precipitation was 60% with a precipitation efficiency of up to 63%; the other ammonium sulfate solutions of 30, 40, 50 and 70% yielded protein precipitation efficiencies of 49.7, 51.8, 53.0 and 61.4%, respectively ( Table 1 ).
Protein solution (called solution II) obtained from precipitation with ammonium sulfate weretested for the presence of ricin by quick test kit (Osborn, USA) with a limit of detection (LOD) of 50 ng. The quick test results indicated that solution II contained ricin. The crude solution II extracts were evaluated for the presence ol ricin by SDS-PAGE ( Figure 2 ); results revealed the expected bands of approximately 64 kDa ( Figure 2A ). The band with expected size in lane 4 (protein sample from precipitation with 60% ammonium sulfate solution) had the highest intensity compared with those in the other lanes, i.e. protein samples from precipitations with 30% (lane 1), 40% (lane 2), 50% (lane 3) and 70% (lane 5) ammonium sulfate solutions ( Figure 2A ). These results showed that protein from solution II extracts is more likely to contain ricin protein and that 60% ammonium sulfate solution is the most suitable solution for ricin precipitation.
Purification of ricin from castor bean seeds
Protein solution II extracts was separated into 20 Iractions ( Figure 2B ) by ion exchange using DEAE-sepharoseeolumn chromatography. All fractions weretested for presenee of ricin by quick test kit (LOD of 5Ong); positive signals were detected in 4 fractions, including 8, 9, 10 and 11. Among these 4 fractions, protein eoncentrations (presented as OD values) of fractions 9 and 10 were highest compared to the other fractions ( Figure 2B ). Protein from fractions 9 and 10 were mixed and referred to as solution III. This solution was used for ricin purification via gel filtration using gel sephadex G100. Purified protein solution collected after gel filtration (called solution IV) was used for immunoblot analysis with ricin antibodies. Immunoblot analysis showed a band of 64 kDa for protein samples without 2-mereaptoethanolamine (2-ME) treatment ( Figure 2C ,lane 1) and a double band of 30 kDa and 34 kDa lor protein samples with 2-ME treahnent, required to break disullide bridges ( Figure 2C , lane 2). The immunoblot results for commercial standard ricin with A chain (lane 1) and B chain (lane 2) are presented in Figure 2D . These results indieate that isolation and purifieation of total ricin protein was sueeessful. For our 4-step procedure, isolation efficiency for eaeh step was ealculated and presented in Table 2 , with the overall efficiency for purified ricin isolation at 9.0 %.
Qualitativeanalysis for ricin by MS/MS
Further, LC-MS/MS analysis was performed to identify ricin protein in the purified samples (protein solution IV). Gel bands from the immunoblotting experiment were excised and digested with trypsin and then analyzed by LC-MS/MS. Protein identification was evaluated using a Mascot score higher than 40; results are shown in Figure 3 . Fragmentation of the peptide backbone in the MS/MS produced a series of b and y peaks, marked in the MS/MS. Amino acid sequence of ricin protein derived from EST gi|255587301is presented in Figure 3A . Data analysis of the b and y peaks from the MS/MS spectrum led to the identification of four peptides with sequences including HEIPVLPNR Figure 3B , SFIICIQMISEAAR ( Figure 3C ), SAPDPSVITLENSWGR ( Figure 3D ) and LSTAIQESNQGAFASPIQLQR ( Figure 3E ). All the sequences are parts of the ricin protein. These results confirm that the protein excised from the appropriate bands was ricin and demonstrated successful isolation and purification of total ricin from castor bean seeds.
Cytotoxicity of ricin on human normal skin keratinocyte (HaCaT) cells and human melanoma SKMEL28 cells
Cytotoxicity of ricin was examined on HaCaT and SKMEL28 cells. The effects of ricin at various concentrations, including 0.1, 0.5, 1.0, 2.5, 5.0, 10, 100, 1000 and 10000 ng/mL, on inducing cell death of HaCaT cells after 48 h were evaluated to find the IC50 value ( Figure 4A-H ). Results showed that the IC50 for HaCaT cells was 5.2 ng/mL ( Figure 4l ). Similarly, the dose-dependent effects of ricin were evaluated at various concentrations, including 0.1, 0.5, 1.0, 2.5, 5.0, 10, 100, 1000 and 10000 ng/mL. Induction of cell death of SKMEL28 cells after 48 h was also evaluated ( Figure 5A-H ); the IC50 of ricin for SKMEL28 cells was 34.1 ng/mL after treatment with ricin for 48 h ( Figure 5l ). These results revealed that cytotoxicity of ricin on normal skin cells was higher than that for melanoma skin cancer cells. A substance with IC50 in the range of 1-10 μg/mL is normally considered as very toxic. Our results confirm that ricin is a highly toxic substance for cells with IC50 in the range of 5- 40ng/mL.
Effect of ricin on tumorigenesis of SMEL28 cells in vitro
We next investigated the role of ricin as an anticancer agent. We evaluated ricin at low concentrations (0.0, 1.0 and 3.0 ng/mL), which do not cause induce cell death of SKMEL28 cells or HaCaT cells. Our results showed that treatment with 1 or 3 ng/mL ricindid not decrease the number of colonies but did reduce the size of colonies of SKMEL28 cells formed in soft agar ( Figure 6A , top), compared to untreated SKMEL28 colonies ( Figure 6A , bottom). Figure 6B shows the colonies formed in agartreated with ricin at 0, 1.0and 3.0 ng/mL; the average sizes of colonies were also examined Figure 6C . Additionally, we also investigated the effects of ricin at concentrations of 1 and 3 ng/mL on colony formation of HaCaT cells. However, the HaCaT cells could not form colony in soft agar because these cells lackthe typical characteristics of cancer cells ( Figure 6D ). SKMEL28 and HaCaT cells were treated with or without ricin at 3.0 ng/ml for 48h before harvesting to isolate total protein. Immunoblot experiments were carried out to examine the changes of ERK and p-ERK; tubulin was used as an internal control in ricin-treated cells and compared with those in untreated cells. Our results showed that treatment SKMEL28 cells with ricin led to decreased protein expression levels of ERK and p-ERK while tubulin levels wereunchanged ( Figure 6E , lane 1), when compared with those in untreated-SKMEL28 cells ( Figure 6E , lane 2). The expression levels of ERK and p- ERK in ricin-treated SKMEL28 cells ( Figure 6F , lane 1) were 2.38- and 3.12-fold lower than those of control SKMEL28 cells ( Figure 6F , lane 2), while treatment with ricin did not affect expression levels of ERK and p- ERK in HaCaT cells ( Figure 6G ). These results suggestthat ricin mayreduce the tumorigenesis of melanoma skin cancer SKMEL28 cells via downregulation of ERKs (ERK and p-ERK).
Effect of ricin on cellular apoptosis
The effect of ricin on apoptosis of the HaCaT and SKMEL28 cells were investigated by using annexin V apoptosis assay on FACSCanto system. Dot blot map with intensity value of FITC (Y axis) and PI (X axis) presented the percentage of cells at different stages of early apoptosis (Q1), late apoptosis (Q2), normal (Q3) and necrosis (Q4) ( Figure 7 ). Total percentages of cellular apoptosis were calculated as quadrant Q1+Q2.
It was indicated that treatment with ricin at 1 and 3 ng/mL did not induce apoptosis of HaCaT cells but SKMEL28 cells. Dot blot map reflected the highest number of cells induced apoptosis in SKMEL28 cells treated with 3 ng/mL, 2.1 folds higher than the control, and 1.4 folds at 1 ng/mL. Meanwhile the percentages of either early (Q1) or late stage (Q2) of apoptosis are similar on both control and ricin-treated HaCaT cells.
Discussion
Ricin is comprised of a catalytic A subunit (A chain)and a lectin B subunit (B chain) which mediates cellular uptake. For ricin, these chains are referred to as ricin toxin A-chain and ricin toxin B-chain, respectively Ready et al., 1986. Toxicity of ricin is expressed as its ability to chemically inactivate the eukaryotic ribosome by hydrolysis of a single adenine base (A4324) on the sarcin-ricin loop (SRL) of the 28S rRNA of the large subunit. This leads to loss of ribosomal activity and finally results in cell and/or tissue death Endo and Tsurugi, 1987Endo et al., 1988.Ricin A chain has been long reported to be a potential drug for various cancers, including leukemia, Hodgkin’s diseases, breast cancer, cervical cancer and melanoma Ghosh et al., 2013LoRusso et al., 1995Spitler, 1986Spitler et al., 1987Tyagi et al., 2015. However, other previous reports have shown that ricin B chain and total ricin also have antitumor activities in cervical cancer (Sadraeian et al., 2013; Liao et al., 2012).
Although ricin has potential as an anticancer agent, its specificity is one of the greatest challenges for the application of ricin in cancer therapy. Therefore, for practicality, ricin is mainly used in immunotoxic therapy as monoclonal antibody-conjugated forms to increase the specificity of the drug towards target cells and to decrease side effects (Epler et al., 2012Alipour et al., 2013; Buonocore et al., 2011). Recently, developmentsin nanotechnology, especially nanoliposomes and its applications, have led to investigations of drug delivery systems to target cancer cells. From this, many new generations of liposomal ricin have been developed. Several reports have shown that ricin encapsulated into nanoliposomes showed antitumor abilities in various types of cancers Epler et al., 2012Tyagi et al., 2015 Buonocore et al., 2011). This led to our interest in the design and development of a nanoliposome system carrying ricin for future targeted treatment of cancers, including melanoma.
Melanoma is one of the most aggressive cancers in the world, particularly in Europe, United State and Australia Gray-Schopfer et al., 2007Meier, 2005. So far, there is no effective drug for treating melanoma. In the past, ricin A chain,but not ricin B chain and/or total ricin,was used as an immunotoxin for treatment of melanoma Spitler, 1986Spitler et al., 1987.
However, almost all these studies were mainly focused on investigatingthe effects of ricin on metastatic melanoma in animal models rather than on cell lines. There have been only a few studies using melanoma cell lines for research; these studies showed that ricin-A-chain immunotoxin could penetrate into tumor spheroids and kill cells, though the mechanism was unclear Kikuchi et al., 1992.
In this study, for the first time, the toxic effects of total ricin on melanoma SKMEL28 cells and normal skin keratinocyte HaCaT cells will be evaluated and the IC50 values determined for purified ricin on these cells. We showed that purified total ricin could decrease the size of colonies of SKMEL28 cells. In general, during development and division stages, a colony should be in rounded shape with a bright color in the outer area. This reflects that cells in the outer area are still alive (bright color as indication) and dividing equally in every direction (round shape as indication). However, under the effect of a drug, cells may undergo apoptosis and colonies will be broken down, leading to change in shape and color. A darkening of color in the outer area means that cells in the outer area are dying from apoptosis or necrosis. Results in Figure 6B-D showed that treatment with ricin at low concentrationsnot only reduced the size of the colonies but caused a subtle change in shape of colonies and a slightly darker color in theouter area of those colonies, compared to control colonies ( Figure 6B ).
Furthermore, our data reveal that total ricin may reduce the tumorigenesis of cellsin vitro via downregulation of ERK and p-ERK protein levels. It is well-known that the development of cancer, including melanoma, is related to phosphorylation/activation of two signaling pathways: MAPK (RAS/RAF/MEK/ERK) Meier, 2005. Both ERK and phosphorylated ERK (p- ERK) are key molecules which contribute to regulation of proliferation, differentiation and invasion of cancer cells Gray-Schopfer et al., 2007Meier, 2005. It was reported that increased expression of ERK appears in more than 90% of melanoma cases Cohen et al., 2002 and inhibition of expression of molecules such as RAF (BRAF) and MEK leads to reduced expression of ERK McCubrey et al.,2007. Previous reports have also shown that inhibition of ERK/p-ERK will result in the reduction of colony formation ability of cancer cells. ERK is a downstream molecule of the MAPK signaling, which is known to regulate the proliferation of cells and colony formation in vitro and tumor formation in vivo Gray-Schopfer et al., 2007Meier, 2005. In accordance with these previous findings, our results showed the relationship between downregulation of ERK/p-ERK and reduction of colony size in soft agar ( Figure 6 ). Another interesting thing is the fact that ricin at low concentrations (1 and 3 ng/mL) barely affected apoptosis of HaCaT but significantly induced apoptosis of SKMEL28 cells ( Figure 7 ). These results suggested that total ricin might be a good candidate for melanoma treatment.
References
-
C.
Cohen,
A.
Zavala-Pompa,
J.H.
Sequeira,
M.
Shoji,
D.G.
Sexton,
G.
Cotsonis,
F.
Cerimele,
B.
Govindarajan,
N.
Macaron,
J.L.
Arbiser.
Mitogen-actived protein kinase activation is an early event in melanoma progression. Clinical Cancer Research.
2002;
8
:
3728-3733
.
-
Y.
Endo,
K.
Tsurugi.
RNA N-glycosidase activity of ricin A-chain. Mechanism of action of the toxic lectin ricin on eukaryotic ribosomes.. Journal of Biological Chemistry.
1987;
262
:
8128-8130
.
-
Y.
Endo,
K.
Tsurugi,
H.
Franz.
The site of action of the A-chain of mistletoe lectin I on eukaryotic ribosomes The RNA N -glycosidase activity of the protein. FEBS Letters.
1988;
231
:
378-380
.
-
A.
Engert,
C.
Gottstein,
U.
Winkler,
P.
Amlot,
S.
Pileri,
V.
Diehl,
P.
Thorpe.
Experimental Treatment of Human Hodgkin’s Disease with Ricin A-Chain Immunotoxins. Leukemia & Lymphoma.
1994;
13
:
441-448
.
-
A.
Engert,
G.
Martin,
M.
Pfreundschuh,
P.
Amlot,
S.- M.
Hsu,
V.
Diehl,
P.
Thorpe.
Antitumor effects of ricin A chain immunotoxins prepared from intact antibodies and Fab′ fragments on solid human Hodgkin’s disease tumors in mice. Cancer.
1990;
research50
:
2929-2935
.
-
K.
Epler,
D.
Padilla,
G.
Phillips,
P.
Crowder,
R.
Castillo,
D.
Wilkinson,
B.
Wilkinson,
C.
Burgard,
R.
Kalinich,
J.
Townson.
Delivery of Ricin Toxin A-Chain by Peptide-Targeted Mesoporous Silica Nanoparticle-Supported Lipid Bilayers. Advanced Healthcare Materials.
2012;
1
:
348-353
.
-
E.
Fagone,
E.
Conte,
E.
Gili,
M.
Fruciano,
M.P.
Pistorio,
D.
Lo Furno,
R.
Giuffrida,
N.
Crimi,
C.
Vancheri.
Resveratrol inhibits transforming growth factor-β-induced proliferation and differentiation of ex vivo human lung fibroblasts into myofibroblasts through ERK/Akt inhibition and PTEN restoration. Experimental Lung Research.
2011;
37
:
162-174
.
-
G.
Funatsu,
M.
Ishiguro,
M.
Funatsu.
Structure and Function of Ricin D. In Animal, Plant, and Microbial Toxins. Springer Science + Business Media.
1976;
:
123-130
.
-
P.C.
Ghosh,
N.
Tyagi,
S.S.
Rathore.
Efficacy of Liposomal Monensin on the Enhancement of the Antitumour Activity of Liposomal Ricin in Human Epidermoid Carcinoma (KB) Cells. Indian Journal of Pharmaceutical Sciences.
2013;
75
:
16
.
-
V.
Gray-Schopfer,
C.
Wellbrock,
R.
Marais.
Melanoma biology and new targeted therapy. Nature.
2007;
445
:
851-857
.
-
H.-Y.
Jang,
J.-H.
Kim.
Isolation and biochemical properties of ricin from Ricinus communis. KOREAN BIOCHEMICAL JOURNAL.
1993;
26
:
98-98
.
-
M.
Kato,
K.
Takeda,
K.
Hossain,
N.D.
Thang,
Y.
Kaneko,
M.
Kumasaka,
O.
Yamanoshita,
N.
Uemura,
M.
Takahashi,
N.
Ohgami.
A redox-linked novel pathway for arsenic-mediated RET tyrosine kinase activation. Journal of Cellular Biochemistry.
2010
.
-
T.
Kikuchi,
T.
Ohnuma,
J.F.
Holland,
L.E.
Spitler.
Penetration of anti-melanoma immunotoxin into multicellular tumor spheroids and cell kill effects. Cancer Immunology Immunotherapy.
1992;
35
:
302-306
.
-
O.
Kumar,
A.B.
Nashikkar,
R.
Jayaraj,
R.
Vijayaraghavan,
A.O.
Prakash.
Purification and Biochemical Characterisation of Ricin from Castor Seeds. Defence Science Journal.
2004;
54
:
345-351
.
-
J.M.
Lord,
L.M.
Roberts,
J.D.
Robertus.
Ricin: structure, mode of action, and some current applications. The FASEB Journal.
1994;
8
:
201-208
.
-
P.M.
LoRusso,
P.L.
Lomen,
B.G.
Redman,
E.
Poplin,
J.J.
Bander,
M.
Valdivieso.
Phase I Study of Monoclonal Antibody-Ricin A Chain Immunoconjugate Xomazyme-791 in Patients with Metastatic Colon Cancer. American Journal of Clinical Oncology.
1995;
18
:
307-312
.
-
J.A.
McCubrey,
L.S.
Steelman,
W.H.
Chappell,
S.L.
Abrams,
E.W.T.
Wong,
F.
Chang,
B.
Lehmann,
D.M.
Terrian,
M.
Milella,
A.
Tafuri.
Roles of the Raf/MEK/ERK pathway in cell growth, malignant transformation and drug resistance. Biochimica et Biophysica Acta (BBA) - Molecular Cell Research.
2007;
1773
:
1263-1284
.
-
F.
Meier.
The RAS/RAF/MEK/ERK and PI3K/AKT signaling pathways present molecular targets for the effective treatment of advanced melanoma. Frontiers in Bioscience.
2005;
10
:
2986
.
-
G.W.
Osmond,
E.M.
Masko,
D.S.
Tyler,
S.J.
Freedland,
S.
Pizzo.
In vitro and in vivo evaluation of resveratrol and 3,5-dihydroxy-4’-acetoxy-trans-stilbene in the treatment of human prostate carcinoma and melanoma. Journal of Surgical.
2013;
Research179
:
e141-e148
.
-
M.P.
Ready,
D.T.
Brown,
J.D.
Robertus.
Extracellular localization of pokeweed antiviral protein. Proceedings of the National Academy of Sciences.
1986;
83
:
5053-5056
.
-
V.W.
Rebecca,
R.R.
Massaro,
I.V.
Fedorenko,
V.K.
Sondak,
A.R.A.
Anderson,
E.
Kim,
R.K.
Amaravadi,
S.S.
Maria-Engler,
J.L.
Messina,
G.T.
Gibney.
Inhibition of autophagy enhances the effects of the AKT inhibitor MK-2206 when combined with paclitaxel and carboplatin in BRAF wild-type melanoma. Pigment Cell & Melanoma Research.
2014;
27
:
465-478
.
-
D.-H.
Shin,
O.-H.
Kim,
H.-S.
Jun,
M.-K.
Kang.
Inhibitory effect of capsaicin on B16-F10 melanoma cell migration via the phosphatidylinositol 3-kinase/Akt/Rac1 signal pathway. Experimental and Molecular Medicine.
2008;
40
:
486
.
-
L.E.
Spitler.
Immunotoxin therapy of malignant melanoma. Medical oncology and tumor pharmacotherapy.
1986;
3
:
147-152
.
-
L.E.
Spitler,
M.
del Rio,
A.
Khentigan,
N.I.
Wedel,
N.A.
Brophy,
L.L.
Miller,
W.S.
Harkonen,
L.L.
Rosendorf,
H.M.
Lee,
R.P.
Mischak.
Therapy of patients with malignant melanoma using a monoclonal antimelanoma antibodyricin A chain immunotoxin. Cancer research.
1987;
47
:
1717-1723
.
-
N.D.
Thang,
I.
Yajima,
M.Y.
Kumasaka,
S.
Ohnuma,
T.
Yanagishita,
R.
Hayashi,
H.U.
Shekhar,
D.
Watanabe,
M.
Kato.
Barium Promotes Anchorage-Independent Growth and Invasion of Human HaCaT Keratinocytes via Activation of c-SRC Kinase. PLoS ONE.
2011;
6
:
e25636
.
-
N.
Tyagi,
M.
Tyagi,
M.
Pachauri,
P.C.
Ghosh.
Potential therapeutic applications of plant toxin-ricin in cancer: challenges and advances. Tumor Biol.
2015;
36
:
8239-8246
.
-
U.H.
Weidle,
G.
Tiefenthaler,
C.
Schiller,
E.H.
Weiss,
G.
Georges,
U.
Brinkmann.
Prospects of bacterial and plant protein-based immunotoxins for treatment of cancer. Cancer Genomics-Proteomics.
2014;
11
:
25-38
.
-
I.
Yajima,
N.
Uemura,
S.
Nizam,
M.
Khalequzzaman,
N.D.
Thang,
M.Y.
Kumasaka,
A.A.
Akhand,
H.U.
Shekhar,
T.
Nakajima,
M.
Kato.
Barium inhibits arsenic-mediated apoptotic cell death in human squamous cell carcinoma cells. Archives of Toxicology.
2012;
86
:
961-973
.
Comments
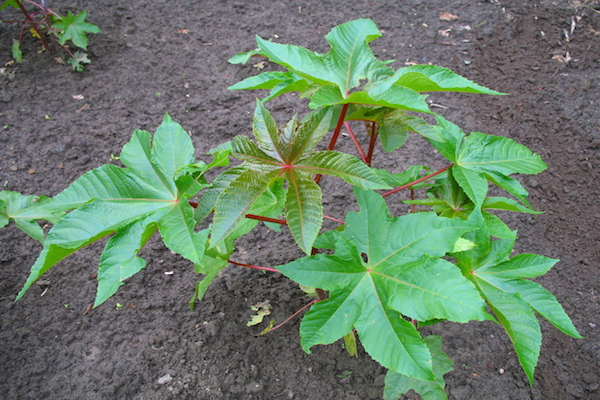
Downloads
Article Details
Volume & Issue : Vol 3 No 05 (2016)
Page No.: 633-644
Published on: 2016-05-27
Citations
Copyrights & License

This work is licensed under a Creative Commons Attribution 4.0 International License.
Search Panel
Pubmed
Google Scholar
Pubmed
Google Scholar
Pubmed
Google Scholar
Pubmed
Google Scholar
Pubmed
Search for this article in:
Google Scholar
Researchgate
- HTML viewed - 20116 times
- Download PDF downloaded - 2532 times
- View Article downloaded - 15 times