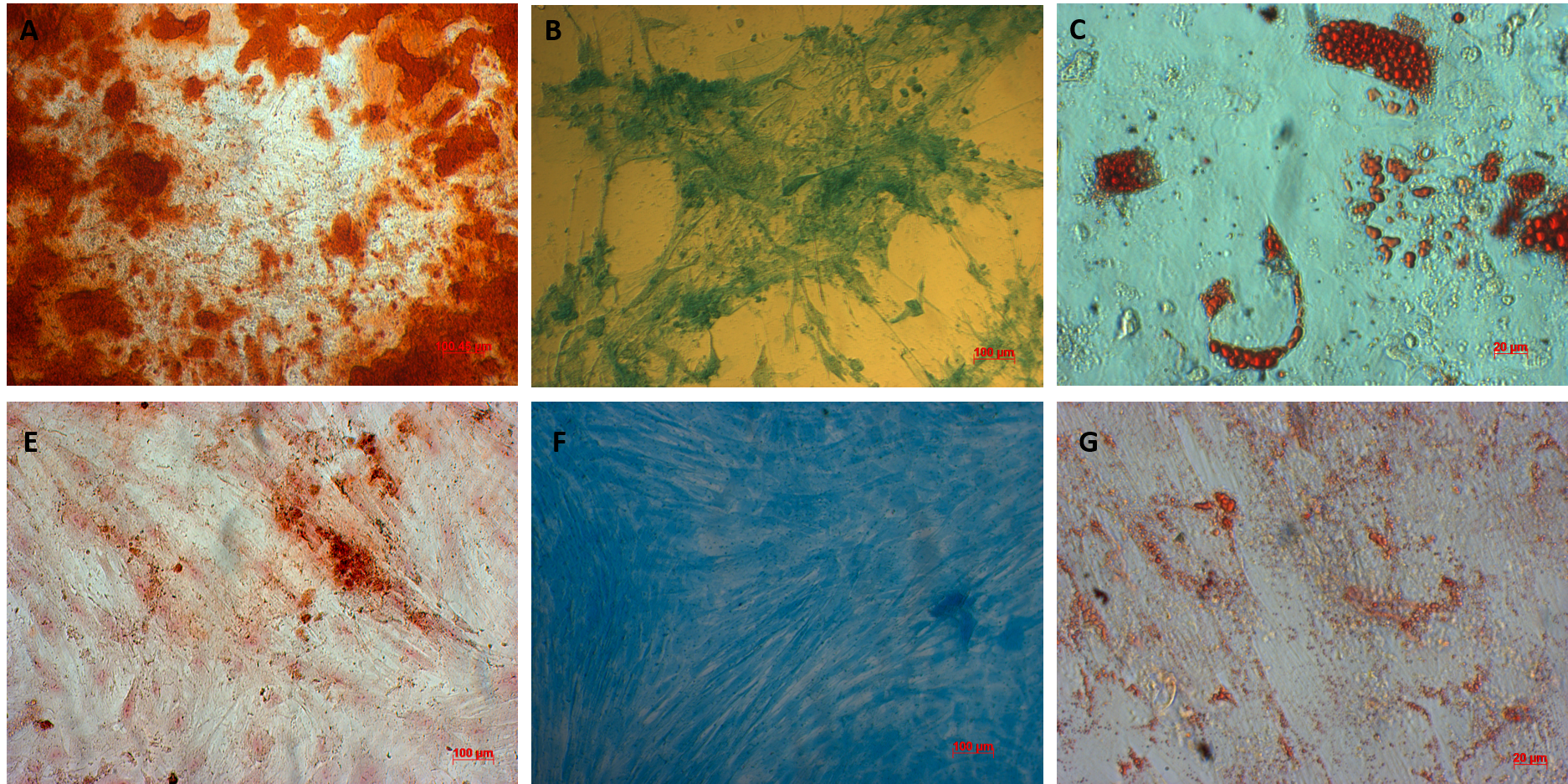
The subpopulation of CD105 negative mesenchymal stem cells show strong immunomodulation capacity compared to CD105 positive mesenchymal stem cells
- Department of Plastic and Aesthetic Surgery, Pham Ngoc Thach University of Medicine, Ho Chi Minh City, Viet Nam
- Stem Cell Institute, VNUHCM University of Science, Ho Chi Minh City, Viet Nam
- Laboratory of Stem Cell Research and Application, VNUHCM University of Science, Ho Chi Minh City, Viet Nam
Abstract
Introduction: Human mesenchymal stem cells (MSCs) are the most popular stem cells applied in disease treatment. MSCs can be isolated and in vitro expanded from various sources such as bone marrow, peripheral blood, umbilical cord blood, umbilical cord tissue, and adipose tissue. According to Dominici et al. (2006), MSCs should express CD105, an essential marker used to confirm MSCs. However, some recent studies have show that MSCs contained a subpopulation that is negative for CD105. This study aimed to compare the immune modulation capacity of 2 populations of CD105 positive (CD105+) and negative (CD105-) MSCs derived from 2 sources: human adipose tissue (AT) and human umbilical cord (UC).
Methods: MSCs were isolated from human adipose tissues (adipose tissue-derived mesenchymal stem cells – AT-MSCs) and human umbilical cord (umbilical cord-derived mesenchymal stem cells – UC-MSCs) according to previously published protocols. The two populations of CD105- and CD105+ MSCs were sorted based on the expression of CD105 from AT-MSCs and UC-MSCs. Four populations of CD105 (AT-MSCs, CD105+ AT-MSCs, CD105- UC-MSCs, and CD105+ UC-MSCs) were used to compare the phenotype as well as in vitro differentiation potential; then they were used to evaluate the immune modulation capacity by allogeneic T cell suppression and cytokine release.
Results: The results showed that CD105- MSCs from AT and UC exhibited an immune modulation capacity that was much stronger than CD105+ MSCs from the same source of AT and UC. The strong immunomodulation of CD105- MSCs may relate to autocrine production of TGF-beta 1 by MSCs.
Conclusion: The results suggested that CD105- MSCs are promising MSCs for application in regenerative medicine, especially for the treatment of diseases related to inflammation.
Introduction
Mesenchymal stem cells (MSCs) were used in the clinic to treat various diseases 1,2,3. Although the mechanisms of MSCs in the different therapies may differ, there are three main mechanisms of MSCs which have been elucidated. The first mechanism relates to the homing and differentiation of MSCs at injured sites to help to promote healing and regeneration at those injury sites. The second mechanism relates to the production and secretion of cytokines, , growth factors from MSCs that affect other cells in a paracrine manner. The last mechanism is immune modulation (immunomodulation), which refers to the ability of MSCs to modulate the immune system via direct interactions with immune cells or via indirect effects mediated by cytokines produced by MSCs 4,5. Of these mechanisms, the mechanism relating to immunomodulation has been a central focus, leading to the wide use of some approved MSC-based products for graft versus host disease (GVHD) and knee osteoarthritis.
Various studies have introduced MSC manufacturing with GMP compliance in the production of MSC for clinical applications. However, MSCs consist of a heterogeneous cell population, which is the reason why they cannot be confirmed by a unique marker. The expression of CD105 in MSCs is considered as one of the essential markers for MSC confirmation, according to Dominici (2016)6. However, various studies have show that the expression of CD105 is so variable between different culture conditions. Some recent studies have suggested that the expression of CD105 is related to the differentiation potential. For example, CD105 adipose tissue-derived MSCs (AT-MSCs) were shown to be more prone to differentiate into chondrocytes compared to CD105 AT-MSCs7,8, while CD105 AT-MSCs were more osteogenic 9,10. CD105 MSCs were also shown to be more efficient in the infarcted heart 11, with stronger proliferation and colony formation ability compared with CD105 MSCs 12. However, some other studies have suggested contrary results. For example, Cleary (2016) showed that the expression of CD105 did not relate to chondrogenesis potential 13. The authors also showed that there was no significant difference in chondrogenesis potential between CD105 MSCs and CD105MSCs.
The first report about the difference of immune modulation between CD105 and CD105MSCs in murine AT-MSCs was published by Anderson . (2013) 14. In this study, the authors showed that CD105 MSCs were prone to suppress the proliferation of CD4T cells more efficiently than CD105 MSCs 14. In the study herein, we aimed to evaluate the differences in the in vitro differentiation capacities into osteoblasts and adipocytes and to compare the immune modulation capacities of CD105MSCs versus D105 MSCs derived from human adipose tissue and human umbilical cord.
Materials-Methods
Isolation of MSCs from adipose tissues
Adipose-derived stem cells (ADSCs),or adipose derived mesenchymal stem cells (AT-MSCs), were isolated following the published protocol 15. Briefly, adipose tissues were digested by collagenase using the Cell Extraction kit (Regenemedlab, Ho Chi Minh City, Viet Nam). The stromal vascular fractions (SVFs) were then resuspended into ADSCCult medium (Regenmedlab Co. Ltd, HCMC, VN). These cells were then sub-cultured to the 5 passage and used for experiments. The adipose tissues were collected from donors with consent forms and all manipulations were approved by institutional ethical committee (Van Hanh General Hospital, HCMC, Viet Nam).
Isolation of MSCs from umbilical cord tissues
Umbilical cord-derived mesenchymal stem cells (UC-MSCs) were collected and expanded from umbilical cord tissue . The umbilical cord tissues were collected with consent form. All manipulations were approved by institutional ethical committee (Van Hanh General Hospital, HCMC, Viet Nam). They were placed in saline with antibiotics and transferred to the laboratory. In the laboratory, the umbilical cord vein and artery were removed before the cord was cut into small pieces (1-2 mm). These fragments were placed into a flask with MSCCult medium (Regenmedlab Co. Ltd, HCMC, VN) for seven days. The stem cells would migrate out to the tissues and adhere to the flask surface. After subculturing 3-5 times, these stem cell candidates were verified for MSC phenotype, and then the two cell populations (CD105 and CD105) were isolated.
Characterization of MSCs derived from adipose tissue and umbilical cord
Immunophenotyping by flow cytometry
Cell markers were analyzed following a previously published protocol 15. Briefly, cells were washed twice in PBS containing 1% bovine serum albumin (Sigma-Aldrich, St. Louis, MO, USA). The cells were then stained with anti-CD14-FITC, anti-CD34-FITC, anti-CD44-PE, anti-CD45-FITC, anti-CD73-FITC, anti-CD90-PE, or anti-HLA-DR-FITC antibody (all antibodies were purchased from BD Biosciences, San Jose, CA, USA). Stained cells were analyzed by a FACSCalibur flow cytometer (BD Biosciences). Isotype controls were used in all analyses.
differentiation
For differentiation into adipogenic cells, MSCs were differentiated as previously described 15. Briefly, cells from the 5 passage were plated at a density of 1x10 cells/well in 24-well plates. When cells reached 70% confluency, they were cultured for 21 days in DMEM/F12 containing 0.5 mmol/L 3-isobutyl-1-methyl-xanthine, 1 nmol/L dexamethasone, 0.1 mmol/L indomethacin, and 10% FBS (all reagents were purchased from Sigma-Aldrich). Adipogenic differentiation was evaluated by observing lipid droplets in cells; cells were stained with Oil Red and examined under a fluorescent microscope.
For differentiation into osteogenic cells, MSCs were plated at a density of 1x10 cells/well in 24-well plates. At 70% confluence, cells were cultured for 21 days in DMEM/F12F12 containing 10% FBS, 10 mol/L dexamethasone, 50 μmol/L ascorbic acid-2 phosphate, and 10 mmol/L β-glycerol phosphate (all reagents were purchased from Sigma-Aldrich). Osteogenic differentiation was confirmed by Alizarin red staining, as visualized under a fluorescent microscope.
For differentiation into chondroblasts, MSCs were differentiated according to the guidelines of the Chondrogenesis kit (Thermo Fisher, Waltham, MA, USA).
Cell sorting based on CD105 expression
AT-MSCs and UC-MSCs were suspended up to 10 × 10 cells/ml in PBS solution with human albumin and stained with monoclonal mouse IgG1-anti-human CD105-PE (2.5 μl – 50 μl/10 cells) for 30 min at 4°C. Viability staining was performed using 7-AAD (BD Biosciences). Sorting and analysis of cells were performed using BD FACSMelody apparatus (BD Biosciences).
Mixed lymphocyte reaction (MLR) and CD38 counting
To evaluate the effects of MSCs on phytohemagglutinin (PHA)-stimulated allogeneic lymphocyte proliferation, freshly isolated lymphocytes (1 × 10/well) were stimulated with 2.5 μg/ml PHA (Sigma-Aldrich) and added to MSC cultures, previously seeded and treated with Mitomycin C. Cultures of unstimulated and PHA-stimulated lymphocytes seeded without MSCs were used as controls. After 48 h, the supernatant with lymphocytes and adherent MSCs were collected and stained with an anti-CD38 monoclonal antibody (BD Biosciences). The level of proliferation was measured by evaluating the ratio of CD38+ cells. All experiments were performed in triplicate.
ELISA for quantification of human cytokines
Cell-free supernatants were collected and kept frozen at –80°C until assayed for cytokine concentrations by enzyme-linked immunosorbent assay (ELISA). ELISA kits for IL-1 beta, IFN-gamma, IL-2, PGE2, TNF-alpha and TGF-beta 1 were used following the supplier's instructions (Abcam, Cambridge, UK).
Statistical analysis
The data were analyzed for statistical significance using GraphPad Prism (GraphPad Software, Inc., La Jolla, CA, USA). Data were presented as mean ± SEM. When applicable, a Student's unpaired t-test and one-way ANOVA were used to determine significance; p<0.05 was considered to be statistically significant.
Results
Adipose tissue- and umbilical cord-derived MSCs expressed the particular characteristics of MSCs
Both the adipose-derived MSCs (AT-MSCs) and umbilical cord-derived MSCs (UC-MSCs) adhered well to the surface of the plastic flasks and exhibited the particular fibroblast-like shape (Figure 1). At passage 4, the cells were analyzed for the expression of some common markers of MSCs. The results showed that both AT-MSCs and UC-MSCs expressed the following common markers of MSCs: CD44 (99.67±0.58% and 100% for AT-MSCs and UC-MSCs, respectively), CD73 (99.67±0.58% and 100% for AT-MSCs and UC-MSCs, respectively), CD90 (100% and 99.67±0.58% for AT-MSCs and UC-MSCs, respectively). However, both AT-MSCs and UC-MSCs were negative (or low) for the following hematopoietic markers: CD14 (2.00±2.00% and 3.33±1.16% for AT-MSCs and UC-MSCs, respectively), CD45 (2.00±1.00% and 2.00±1.00% for AT-MSCs and UC-MSCs, respectively), HLA-DR (2.33±1.53% and 1.68±.058% for AT-MSCs and UC-MSCs, respectively); and CD34 (3.67±1.16%, and 2.00±1.73% for AT-MSCs and UC-MSCs, respectively) (Figure 2).
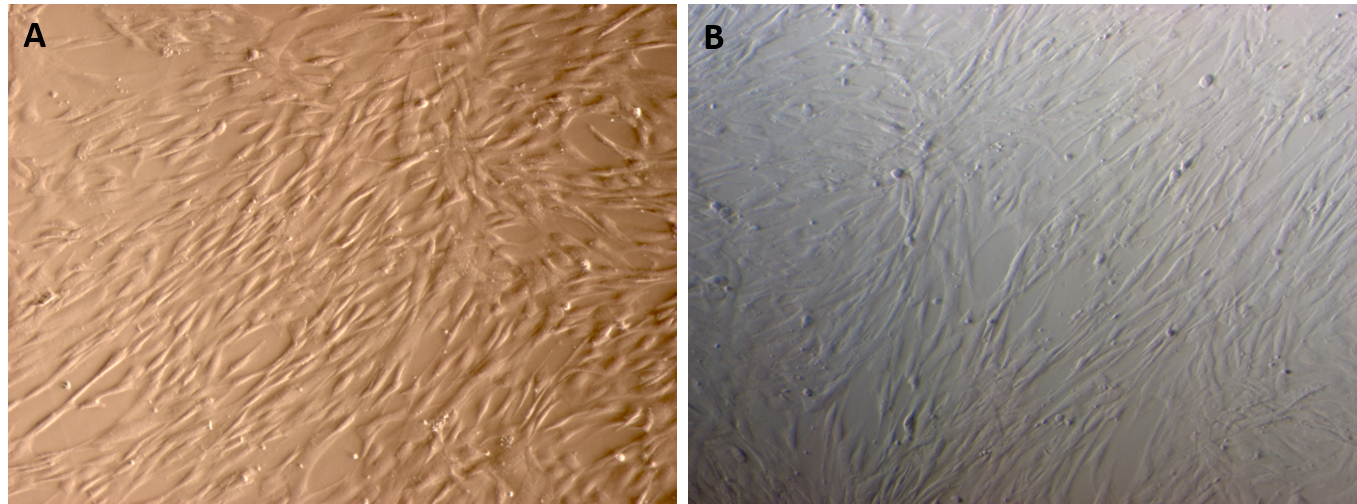
The morphology of AT-MSCs and UC-MSCs on the plastic surfaces of flask. The AT-MSCs (A) and UC-MSCs (B) exhibited fibroblast-like shape when grown in adherent conditions. The magnification x 20.
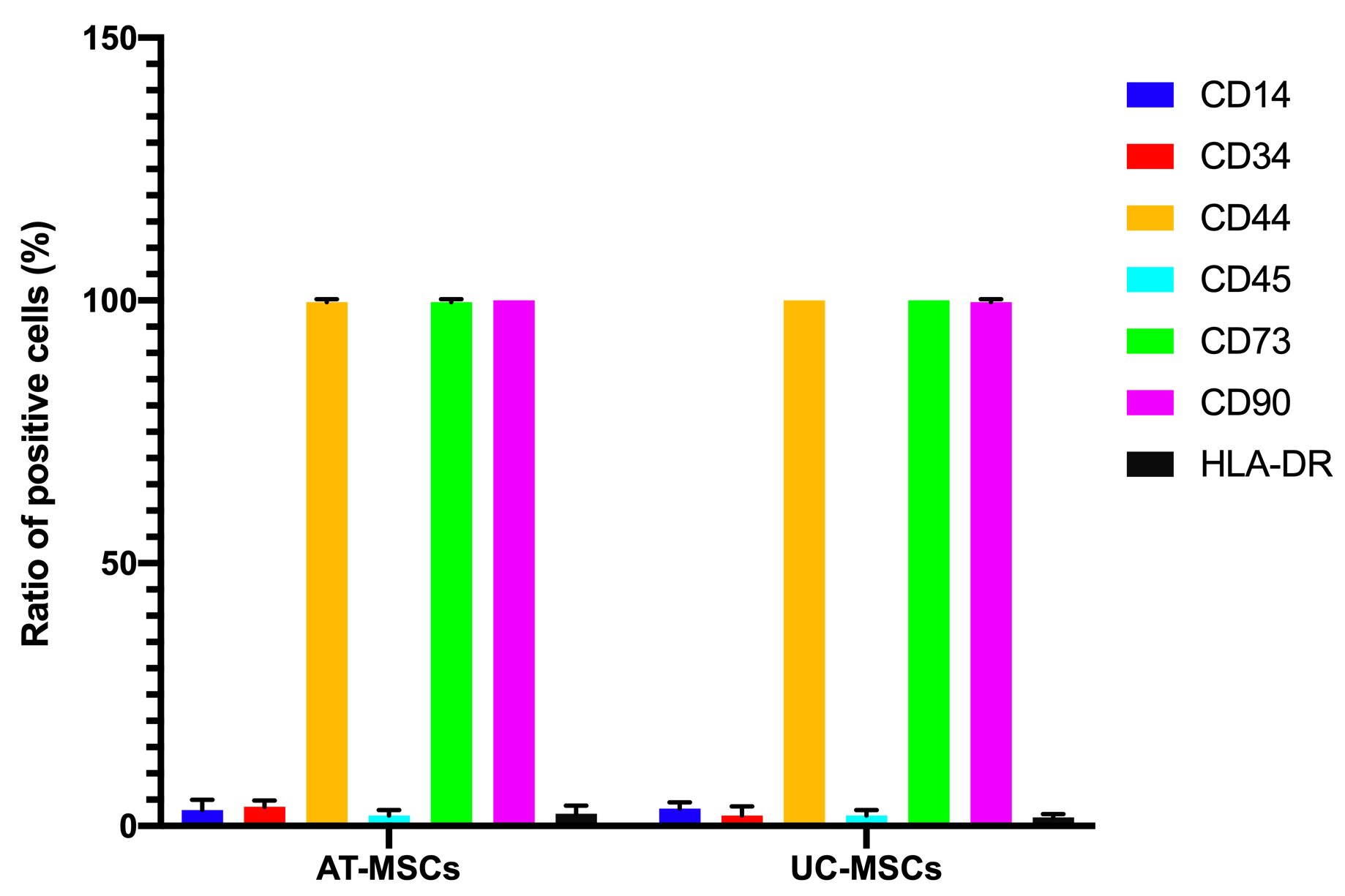
AT-MSCs and UC-MSCs displayed the marker profile of MSCs. Both AT-MSCs and UC-MSCs were negative for hematopoietic markers, such as CD14, CD34, CD45, and HLA-DR, but were strongly positive for markers such as CD44, CD73, and CD90.
AT-MSCs and UC-MSCs could be successfully differentiated into osteoblasts, adipocytes, and chondroblasts. In the induced medium geared toward osteoblast differentiation, both AT-MSCs and UC-MSCs gradually changed their morphology to longer shape, and gradually produced more matrix. After 21 days of induction, the differentiated samples were positive with Alizarin red staining. The dyes combined with calcium in the matrix and displayed the red color.
The AT-MSCs and UC-MSCs were also successfully differentiated into adipocytes. These cells could accumulate lipid droplets (in the cytoplasm) which were capable of being stained with Oil Red. The differentiation of AT-MSCs and UC-MSCs into chondroblasts was also recorded after inducing MSCS for 21 days in the induced medium. The overexpression and accumulation of proteoglycans and collagen I were evaluated in these differentiated cells (Figure 3).
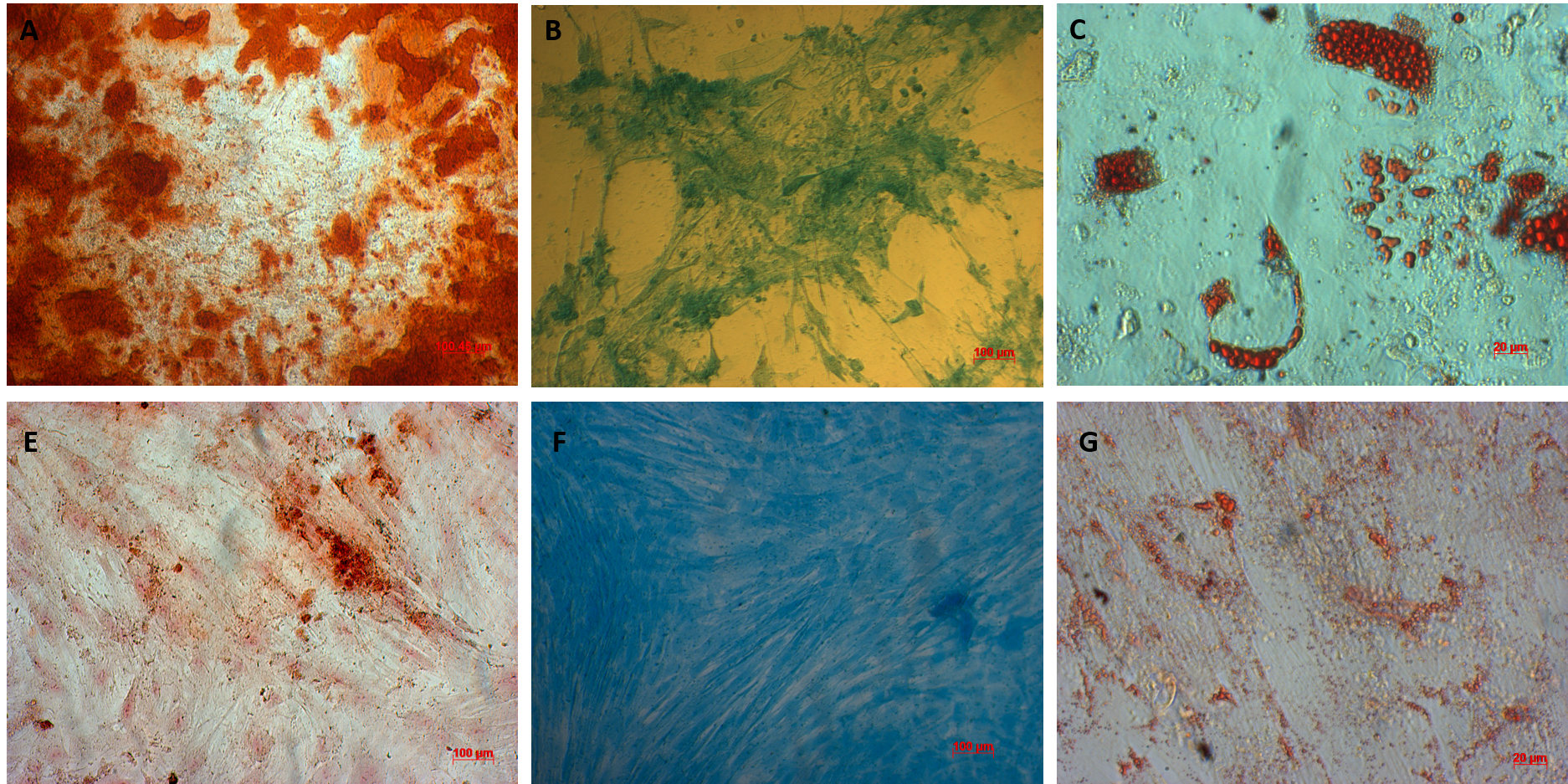
AT-MSCs and UC-MSCs could be differentiated into osteoblast, adipoblasts, and chondroblasts. AT-MSCs and UC-MSCs can be differentiated into osteoblasts that positive with alizarin Red staining (A, E respectively), chronblasts that positive with alcian blue staining (B, F respectively); and adipocytes that positive with oil red staining (C, G respectively).
CD105 negative MSCs expressed all the main characteristics of CD105 positive MSCs
In this assay, we evaluated some main characteristics of CD105 negative and positive MSCs, including expression of markers, cellular shape, and differentiation potential. Both CD105 negative and positive MSC population were sorted based on the expression of CD105 on the surface of MSCs. The purity of CD105 positive and negative cells was confirmed by flow cytometry. The results showed that there were 96% CD105 positive cells in the CD105 positive MSC population, and 2.1% positive cells in the CD105 negative MSCs in AT-MSCs. Moreover, there were 97% CD105 positive cells in the CD105 positive MSC population, and 3.3% positive cells in the CD105 negative MSCs in UC-MSCs. They were cultured to display the differences in their shapes. However, their shapes were not significantly different when detected with naked eyes.
Both these MSCs populations were also evaluated for the expression of other markers such as CD34, CD44, CD45, CD73, CD90, and HLA-DR. The results are represented in Figure 4. There was no significant difference in the expression of these markers between CD105 negative and positive populations of both AT-MSCs and UC-MSCs.
Regarding differentiation capacity of CD105 positive and negative AT-MSCs and UC-MSCs, this was evaluated after inducing differentiation via chemicals. The results showed that the differentiation efficacy was similar between the CD105 negative and positive populations from both AT-MSCs and UC-MSCs by naked eye observations.
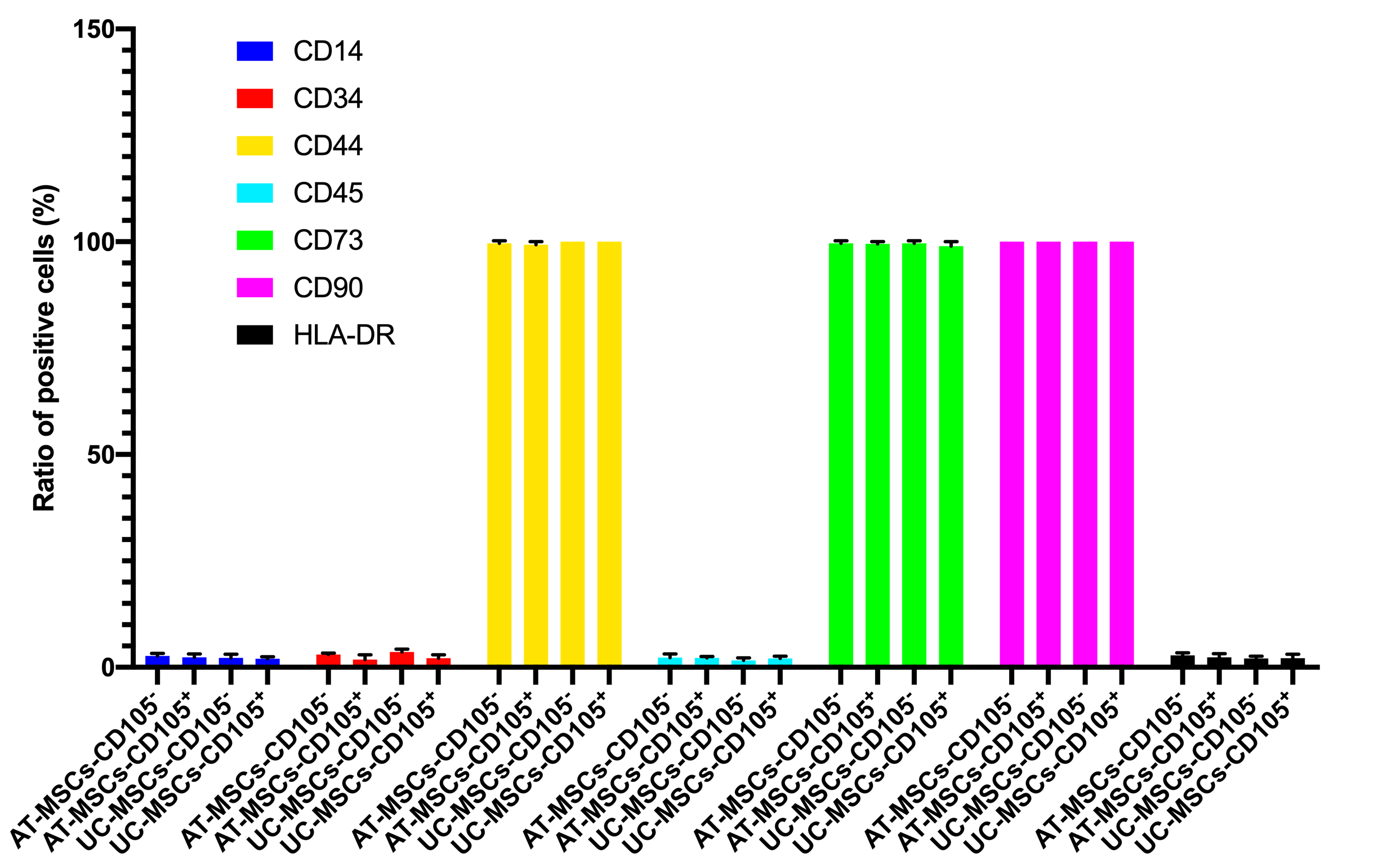
The expression of typical MSC markers in CD105- and CD105+ MSCs derived from AT and UC sources. There was not any significant difference in expression of typical markers between CD105- and CD105+ AT-ADSCs and UC-MSCs.
The CD105 negative MSCs exhibited a stronger immune modulation than CD105 positive MSCs
The immune modulation of CD105 negative and positive AT-MSCs and UC-MSCs were evaluated by mixed lymphocyte reaction (MLR) and CD38 counting, and then by ELISA for quantification of human cytokines.
The effects of both AT-MSCs and UC-MSCs on inhibition of T cell proliferation were observed in both CD105 and CD105 cell populations. Based on CD38 cell counting, the results showed that in the ¼ ratio of MSC/T cell groups, CD105 negative and positive MSCs derived from both AT and UC sources could inhibit the growth of T cells. In the control groups (that lacked MSCs), the CD38 positive cells significantly increased and accounted for 42.67±2.51% and 40.33±1.53 of cells in the sample for AT-MSCs and UC-MSCs groups, respectively. However, this ratio was clearly reduced to 30.33±4.04% for CD105 AT-MSCs, 20.00±5.00% for CD105 AT-MSCs, 15.33±2.51% for CD105 UC-MSCs, and 10.00±2.00% for CD105 UC-MSCs (p<0.05) (Figure 5).
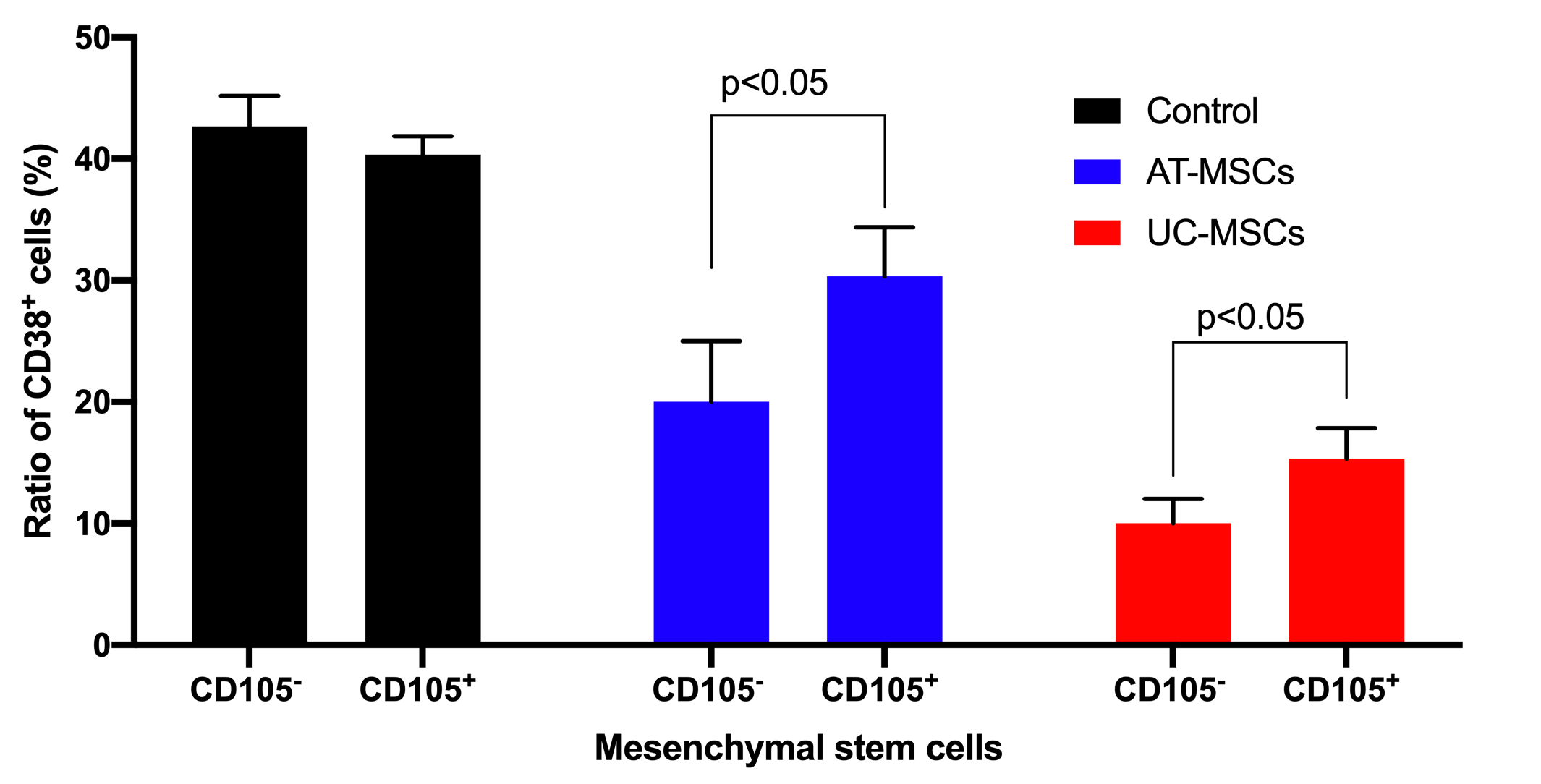
Allogenic T cell proliferation was suppressed by both CD105 negative and CD105 positive MSCs. CD105- MSCs derived from AT and UC sources suppressed T cell proliferation to a greater extent than CD105+ MSCs from AT and UC sources.
The supernatants of cultures (including the PHA-stimulated lymphocytes without MSCs, and PHA-stimulated lymphocytes mixed with MSCs) were analyzed for concentration of cytokines including IFN-gamma, IL-1 beta, IL-2, and TNF-alpha. The ELISA assays showed that these cytokines could be detected in all groups, but the highest cytokine concentrations were recorded in the controls (PHA-stimulated lymphocytes without MSCs). These cytokine concentrations were reduced in groups with MSCs. In the control , the concentrations of IFN-gamma, TNF-alpha, IL-1 beta, and IL-2, respectively, were: 146.67±15.28 pg/mL, 509.67±85.91 pg/mL, 59.00±11.53 pg/mL and 40.33±10.01 pg/mL for PHA-stimulated lymphocytes 72.33±6.80 pg/mL, 253.67±14.84 pg/mL, 38.33±7.64 pg/mL and 32.00±7.21 pg/mL for CD105 AT-MSCs; 48.33±7.64 pg/mL, 197.00±15.40 pg/mL, 11.67±3.22 pg/ML and 17.33±2.52 pg/ML for CD105AT-MSCs; 48.00±8.19 pg/mL, 69.33±11.02 pg/mL, 21.50±7.86 pg/mL and 20.00±5.00 pg/mL for CD105 UC-MSCs; and 27.00±3.61 pg/mL, 34.33±4.04 pg/mL, 11.83±1.89 pg/mL and 10.67±2.52 pg/mL for CD105 UC-MSCs. The results demonstrated that in the CD105 AT-MSC and CD105 UC-MSC groups, all inflammatory cytokines (IFN-gamma, TNF-alpha, IL-1 beta, and IL-2) concentrations were significantly lower than those of the CD105 AT-MSC and CD105 UC-MSC groups when comparing to the same kind of MSCs (p <0.05). The concentrations of these cytokines in the UC-MSC groups (both CD105 cells and CD105 cells) were significantly lower than those in the AT-MSC groups (p <0.05) (Figure 6).
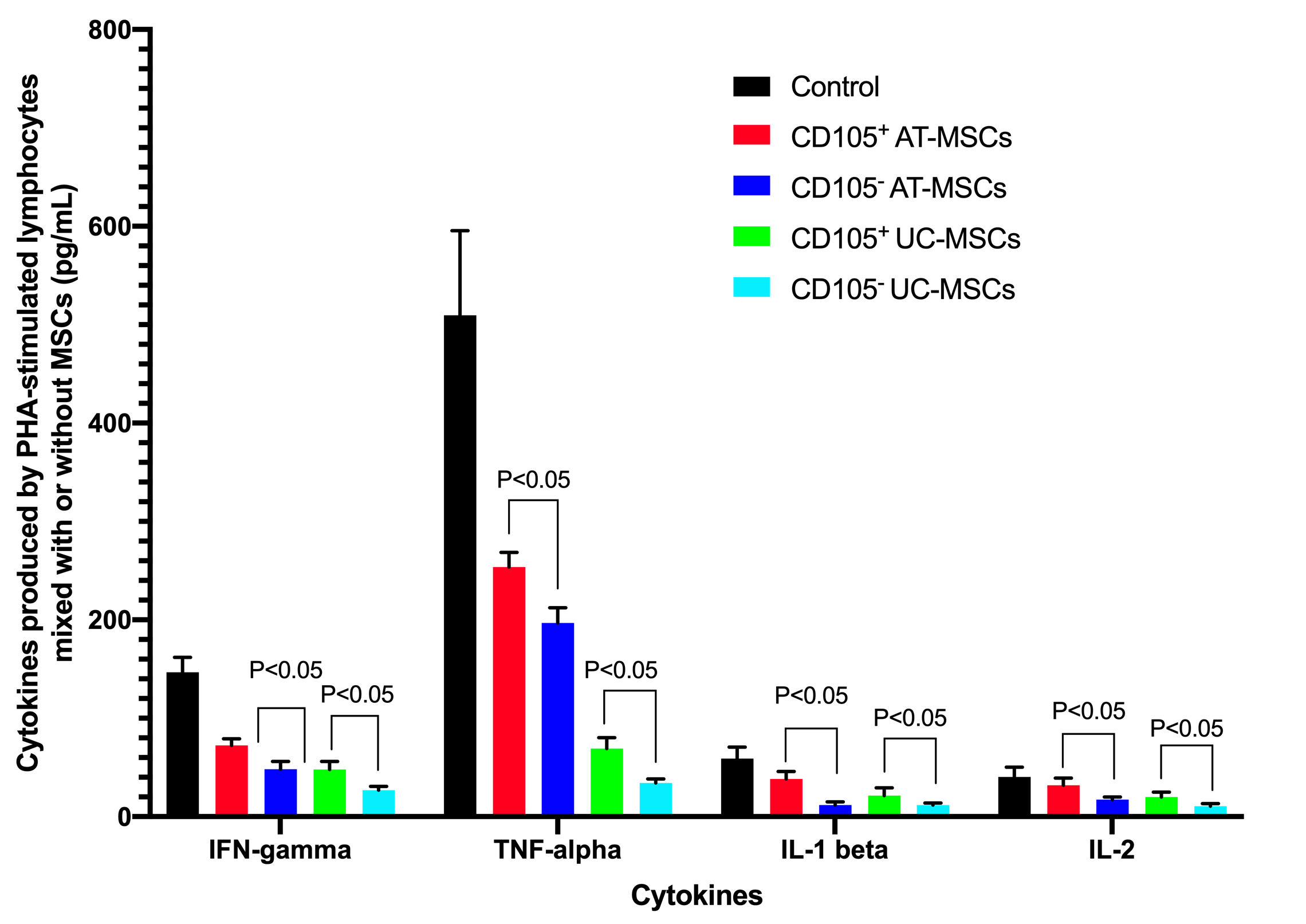
Evaluation of cytokines produced and released by PHA-stimulated lymphocytes mixedwith or without MSCs. MSCs markedly reduced the production of IFN-gamma, TNF-alpha, IL-1 beta and IL-2, compared to cultures without MSCs. The results showed that CD105- MSCs strongly inhibited the production of these cytokines, and to a greater extent than that of CD105+ MSCs from AT and UC sources.
The concentration of TGF-beta 1 in conditioned medium was different between CD105and CD105 cells
TGF-beta 1 is considered the essential factor that affects the immune modulation capacity of MSCs 16. Therefore, in this assay, the TGF-beta 1 concentration was measured to detect the cytokine production difference between CD105and CD105MSCs derived from AT and UC sources. The results showed that at the same number of cells seeded in the 96-well plates, the production of TGF-beta 1 by CD105MSCs were always significantly higher than that of CD105 MSCs (detection of 142 pg/mL in conditioned medium produced by CD105 AT-MSCs. 213 pg/mL in conditioned medium produced by AT-MSCs; 531 pg/mL in conditioned medium by CD105 UC-MSCs . 862 pg/mL in conditioned medium by CD105 UC-MSCs) (p<0.05).
Discussion
MSC now are widely used for certain treatments, particularly for inflammatory diseases. Some products containing MSCs have been approved by the Ministry of Health in countries such as Canada, Korea, and Japan for graft versus host disease and osteoarthritis17,18. The main mechanism of MSCs in treating these diseases is immune modulation. Although immune modulation is an important characteristic of MSCs, the heterogeneous population of MSCs which carry out this function can be so widely different from batch to batch, donor to donor, and from laboratory to laboratory. The expression of CD105 is considered to vary hugely between culture conditions and laboratories 19,20 . Therefore, in this study, we aimed to evaluate the immune modulation of CD105 and CD105cells isolated from AT and UC sources (., termed AT-MSCs and UC-MSCs).
AT-MSCs and UC-MSCs were isolated from adipose tissue and umbilical cord samples, respectively. Before they were used to sort two subsequent populations (CD105and CD105) by FACS, they were confirmed for MSC phenotype based on some criteria that Dominici (2006) have suggested. The results showed that both AT-MSCs and UC-MSCs displayed the following phenotypes of MSCs: (1) adhering on the plastic surface and displaying fibroblast-like shape; (2) expressing mesenchymal markers including CD44, CD73 and CD90 at high levels, and CD105 at low level, while not expressing hematopoietic markers such as CD14, CD34, CD45 and HLA-DR; and (3) successfully differentiating into mesoderm cell lineage including osteoblasts, adipocytes, and chondroblasts. These results are similar and in accordance with results from our studies and those of other groups. The lower expression of CD105 may be related to the serum-free medium culture conditions that were also stated in the previous study. In this study, the reason for the low expression of CD105 was not explained; however, it was noted that the expression of CD105 on MSCs was lower in serum-free culture medium compared to the serum-containing culture medium 19.
Moreover, two cell populations with different expression of CD015 were sorted with high purity and high viability for use in subsequent experiments. The CD105and CD105 cells were directly sorted into 6-well plates for differentiation, into 96-well plates for immune modulation evaluation, and into 8 mL tubes for marker phenotyping. The results confirmed that there was no significant difference in expression of the following MSC markers: CD14, CD34, CD44, CD73, CD90, and HLA-DR. The differentiation potential was also not significantly different in terms of osteoblast differentiation, adipocyte differentiation, and chondroblast differentiation. CD105 expression did not relate to expression of other markers of MSCs or differentiation into osteoblasts, chronblasts, and adipocytes. However, there are some studies that have suggested that the expression of CD105 in MSCs is related to senescence and chondrogenesis of MSCs 9,21. In a study by Cleary . (2016), CD105 expression was not associated with the chondrogenic phenotype nor chondrogenic differentiation 13. The CD105 MSCs from synovial membrane tissues of patients with osteoarthritis had the chondrogenic capacity 9. However, in the study herein, we observed that the expression of CD105 did not affect the stemness or differentiation of MSCs in both AT-MSCs and UC-MSCs. Quantitative analysis of gene expression related to the differentiation of osteoblasts, chondroblasts, and adipocytes was not evaluated in this study.
A recent report showed that the expression of CD105 was related to repairing of infarcted hearts 11. Indeed, the role of CD105 in development and function of the cardiovascular system was determined, including its role in angiogenesis 22, regulation of migration 23, and survival of endothelial cells 24.
However, we found that the expression of CD105 on MSCs derived from both AT and UC sources did affect their immune modulation capacity. The CD105 MSCs strongly inhibited the expression of lymphocytes to a greater extent than CD105 MSCs. That is the reason why the concentrations of all inflammatory cytokines evaluated for the CD105 cells were lower than those for the controls (lymphocytes showed strong proliferation under the effects of PHA).
The effects of CD105 expression on MSCs may depend on the response to TGF-beta. Indeed, CD105 is a component of the TGF-beta receptor. The role of TGF-beta on the differentiation potential and immunomodulation have been evaluated 16,25,26,27. Niu (2017) showed that MSCs inhibited T cell activation by releasing TGF-beta 1 16. Therefore, they showed that if TGF-beta 1 production was reduced, the capacity of T cell inhibition also was decreased. These observations suggested a correlation of CD105 expression on the MSC surface and its effect on immune modulation, and that it could relate to the competition of CD105 with TGF-beta 1, produced by MSCs. Under normal condition , MSCs can produce TGF-beta 1; a part of this TGF-beta 1 can bind to the TGF-beta receptor of which CD105 is a component. The lack of CD105 expression may inhibit TGF beta receptor from binding to TGF-beta 1. Thus, the total TGF-beta 1 from MSCs can affect lymphocytes and inhibit T cell activation.
The effects of TGF-beta 1 on the MSCs in an autocrine manner have been supported in some published studies. In a mouse model, Anderson (2013) showed that in CD105- AT-MSCs exhibited reduced TGF-beta/smad2 signaling in mice 14. The feedback effects of TGF-beta 1 on the CD105 MSCs could inhibit the production of iNOS/NO and IL-6 28. The significant decrease of iNOS/NO and IL6 could reduce the immune modulation mediated by CD105 MSCs.
Lastly, the concentrations of TGF-beta 1 in the supernatant of CD105 MSCs from both AT and UC sources were higher than those for CD105 AT-MSCs and UC-MSCs, respectively. Perhaps there are two reasons for this observation. Firstly, the TGF-beta 1 that was produced by MSCs were found to TGF receptors on the MSC surface. Secondly, there might have been a change in the signaling pathway of TGF- beta 1 production/secretion by CD105 MSCs.
Conclusion
The MSC populations derived from adipose tissue and umbilical cord are heterogeneous. The difference in CD105 expression reflected two different populations with immune modulation. The CD105MSCs and CD105 MSCs have similar stemness and differentiation potential to become osteoblasts, chondroblasts, and adipocytes. The CD105MSCs have a strong capacity of immune modulation compared to CD105 MSCs. The mechanism of this observation is unclear. However, we suggest that the effects of immune modulation of MSCs depend on the level of TGF-beta 1 that is produced by MSCs and secreted into the culture medium. The CD105 MSCs may use TGF-beta 1 in an autocrine mechanism to cause the reduction of TGF-beta 1 in the culture medium and increase immune modulation capacity. The results from our study suggest that CD105 MSCs can be a suitable source for inflammatory disease treatment.
Abbreviations
AT-MSC: Adipose tissue derived mesenchymal stem cell
CD: Cluster of differentiation
GVHD: Graft versus host disease
UC-MSC: Umbilical cord derived mesenchymal stem cell
Competing Interests
Authors declare no conflict of interest.
Authors' Contributions
LHP performed the AT-MSCs and UC-MSCs preparation: isolation and expansion, draft the manuscript; NBV performed the in vitro differentiation and characterization; PVP collected data, analysed the data; performed the immune modulation assays. All authors read and approved the final manuscript for publication.
Funding
This research was funded by Fostering Innovation through Research, Science and Technology, Viet Nam via project 15/FIRST/2a/SCI.