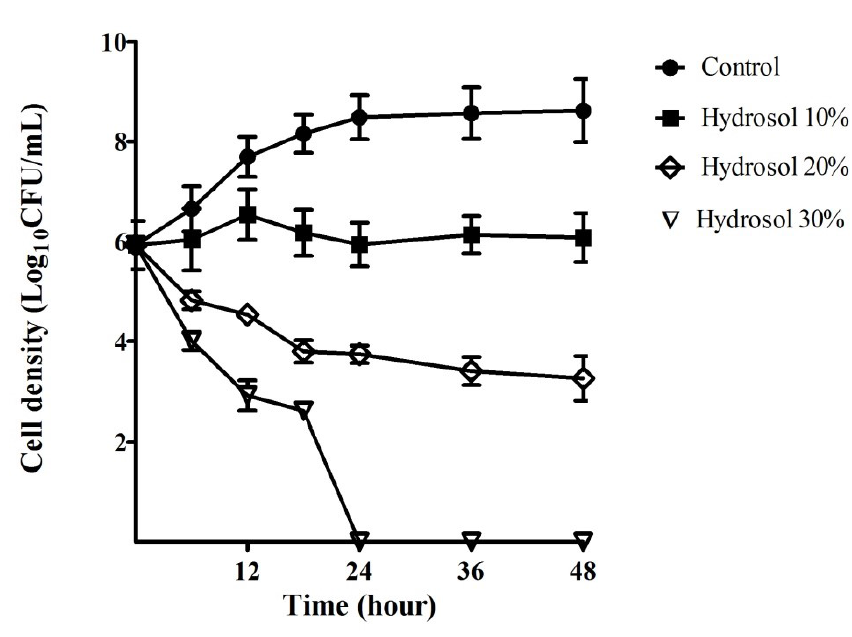
In vitro antimicrobial activity of hydrosol from Litsea cubeba (Lour.) Pers. against Helicobacter pylori and Candida albicans
- 1Faculty of Biology and Biotechnology, VNUHCM University of Science, Ho Chi Minh City, Viet Nam
- 2Thu Dau Mot University, Binh Duong Province, Vietnam
- Faculty of Biology and Biotechnology, VNUHCM University of Science, Ho Chi Minh City, Viet Nam
- aculty of Biology and Biotechnology, VNUHCM University of Science, Ho Chi Minh City, Viet Nam
Abstract
Introduction: Helicobacter pylori and Candida albicans are classified as the most common pathogenic agents in humans. H. pylori is responsible for gastroduodenal diseases and greatly associated with gastric carcinogenesis, while C. albicans is the main cause of fungal urinary tract, genital yeast, and fungal skin infections. The increasing appearance of drug-resistant strains of H. pylori and C. albicans has made the treatment of the infections more serious. Hydrosols from plant steam distillation have been traditionally used in medicine, cosmetics, and culinary uses. They have been recently suggested as antimicrobial agents owing to their safety and ability to reduce the potential of resistance. The aim of the present study is to assess antibacterial and antifungal activities of hydrosols extracted from the fresh fruits of Litsea cubeba against H. pylori and C. albicans. Methods: The L. cubeba fruit hydrosol was obtained by steam distillation method. Evaluation of the growth-inhibiting and microbicidal effects of the hydrosol towards the H. pylori ATCC 43504 and C. albicans ATCC 10231 was determined through MIC (minimal inhibitory concentration), MBC (minimal bactericidal concentration), and MFC (minimal fungicidal concentration) measurements using broth dilution assays. Compositions of the dissolved essential oil (dEO) from the hydrosol were analyzed by GC-MS (gas chromatography-mass spectrometry). Results: The results indicated that the L. cubeba fruit hydrosol exhibited strong antimicrobial ability towards the bacterium H. pylori (MIC of 10%, MBC of 30%) and the yeast C. albicans (MIC of 10%, MFC of 40%). The cells of H. pylori and C. albicans were killed completely after 24 and 18 hours of treatment with 30% and 40% of the hydrosol, respectively. The major constituents of the dEO were geranial (32.92%), neral (27.12%), p-menthan-8-yl acetate (8.45%), 2-cyclopropyl-2-methylspiro[2.2]pentane-1-carboxylic acid (8.09%), linalool (4.24%), and methyl heptenone (4.15%). Conclusion: The results of the study suggest that L. cubeba fruit hydrosols could be used as potent natural antibacterial and antifungal preparations in the global effort to discover safe alternatives to toxic antimicrobial agents.Introduction
Hydrosol is an aqueous layer (or aromatic water) located just below the essential oil (EO) layer during steam distillation of aromatic and medicinal plant materials. Hydrosol is the byproduct of steam distillation of EOs. Almost all hydrosols contain small quantities of dissolved essential oil (dEO). However, some plants contain a high amount of dEO1. Since hydrosols possess a wide range of beneficial properties, such as pH 5.5, pleasant scents, relaxing fragrance and antimicrobial ability, they are traditionally used in cosmetics, food applications, and medicinal purposes in many countries around the world2, 3. These days, hydrosols have attracted an increasing number of researchers because of their eco-friendly, health-benefitting and safe characteristics2, 3. Several recent studies have demonstrated that hydrosols from different plants exhibited strong antimicrobial activities against a wide variety of fungi and bacteria2, 4, 5, 6, 7. Therefore, research on hydrosols would bring promising solutions for controlling pathogenic fungi and bacteria.
and are two of the most common human pathogens that cause a wide range of serious diseases for humans. is a Gram-negative spiral bacterium with the ability to produce urease, persistently colonizing in the gastric mucosa of at least half of the world’s population8, 9. In some developing countries, the rate of infection with can reach up to 80% or higher10. The bacterium has been proven to be responsible for gastroduodenal diseases and is greatly associated with gastric carcinogenesis 11, 12. is a polymorphic fungus that is the common infectious agent of the skin, oral cavity and esophagus, gastrointestinal tract, vagina, and vascular system of humans 13, 14. The fungus, which is uniquely adapted to its human host, often exists as a harmless organism at various mucosa15, 16. However, the pathogen can cause serious conditions under certain circumstances, including life-threatening systemic infection14.
Antibiotic and antifungal therapies were successful in controlling the and infections, greatly contributing to reduction of diseases caused by these pathogens 17, 18. However, the side effects which resulted from the drugs and the rapid emergence of drug-resistant strains have made the therapies for the treatment of and lose their effectiveness 19, 20, 21. Hence, there is an urgent need for new antimicrobial agents for more effective management of these infectious agents.
Increasing public concern about healthy and natural products nowadays has promoted much research focusing on developing new medicines from plant sources. Numerous extracts and EOs were demonstrated to exhibit strong antimicrobial activities towards and without any resistance development of these microbes 22, 23, 24, 25, 26. Fruit of , a member of Lauraceae family, has been used for stimulating the digestive system and in treatment of stomach ache in Vietnam 27, 28, 29 and Malaysia 30. The EO from the fruit of was found to have a strong activity against both Gram-negative and Gram-positive bacteria 31, 32, 33. In addition, the EO and terpenoid extraction from fruits of exhibited effective activity against several pathogenic fungi in plants and humans, including . However, no information has been obtained related to the constituents and the potential of the hydrosol from the fruit to control and .
In the present study, the growth-inhibiting, bactericidal, and fungicidal effects of the hydrosol extracted from steam distillation of the fruit against ATCC 43504 and ATCC 10231 were assessed. Chemical constituents of dEO extracted from the hydrosol were also determined by gas chromatography-mass spectrometry (GC-MS).
Materials and Methods
Plant collection
The fresh samples of (Lour.) Pers. (1806) were collected from the Mang Den Medicinal Plants Garden, in Kon Tum province (the Central Highlands of Vietnam) in June 2018. The identification to species of the specimen was conducted by the method of morphological descriptions. A voucher specimen has been deposited in the PHH Herbarium of Vietnam National University Ho Chi Minh City.
Preparation of hydrosol
An amount of 800 gram of fresh fruit of was finely ground in a mixer and then subjected to steam distillation at 100°C using a Clevenger-type apparatus with 3000 mL of water for 3 hours. The hydrosol was separated by a separation funnel; afterwards, the hydrosol (~1800 mL) was obtained and stored in dark bottles at 4°C until used.
Reagents
Brucella broth (BB), brain heart infusion broth (BHIB), sabouraud dextrose agar (SDA), and sabouraud dextrose broth (SDB) were purchased from Becton Dickinson, Inc. (Sparks, MD, USA). Newborn bovine serum (NBS) was obtained from Hyclone (Longan, UT, USA). Amoxicillin (≥98%) was purchased from Santa Cruz Biotechnology Inc. (Dallas, TX, USA). Nystatin was provided by Merck (Kenilworth, NJ, USA). All other chemicals and reagents used in this study were of analytical grade quality and available commercially.
Microbialstrains and culture conditions
The reference strains of ATCC 43504 and ATCC 10231 were provided by the Oxford University Clinical Research Unit Vietnam (OUCRU - VN) and Department of Plant Biotechnology and Biotransformation, Faculty of Biology and Biotechnology, University of Science, VNU - HCM, respectively. Authentication of these strains was done using MALDI-TOF MS (OUCRU - VN). The bacterial and the yeast strains were stored in BHIB and SDB media, respectively, supplemented with 25% glycerol and placed in a nitrogen liquid container until use.
The strain was cultured on Brucella agar added with 10% NBS, and then incubated at 37C for 3 days under a microaerophilic condition created by a 2.5 L Oxoid AnaeroJar and Oxoid CampyGen sachet (Thermo Fisher Scientific, Waltham, MA, USA). The bacterial suspensions (~ 5x10 CFU/mL) used for the bioassay were made in Brucella broth using a 72-h subculture of on Brucella agar. The strain was activated in SDB medium at 37C for 2 days. The yeast suspensions for bioassay (~ 5x10 CFU/mL) were prepared in SDB medium from a 48-h subculture of the activated . Microbial density was determined using McFarland turbidity standards.
Separation and analysis of chemical constituents of dissolved essential oil
Dissolved essential oil (dEO) was extracted by vigorously shaking the hydrosol (900 mL) with hexane (10:1, v/v) for 30 minutes using the separation funnel. The mixture was allowed to settle and the hexane layer saturated with the dEO was separated from the water layer. The hexane layer was then evaporated at 42C under reduced pressure to obtain the dEO. The remaining water was removed from dEO by using anhydrous sodium sulphate. The yield of the dEO was determined based on the fresh weight of the sample. The dEO was kept in a dark vial and put in a fridge at -20°C until analyzed.
The dEO was analyzed by GC-MS, conducted at the Central Lab for Analysis, University of Science, VNU - HCM. In brief, 1 µL of the dEO was diluted in 1 mL hexane, and then 1 µL of the solution was analyzed by GC-MS using a Agilent 6890N gas chromatograph coupled to a mass spectrometer (Agilent 5975C inert MSD, Santa Clara, CA, USA). Compositions were separated on a fused silica capillary column (HP-5MS) coated with polydimethylsiloxane (60 m × 0.32 mm internal diameter, 0.30 μm film thickness). The following oven temperature program was initiated at 50°C for 2 minutes, ramped at a rate of 2°C/minute to 80°C, 5°C/minute to 150°C, 10°C/minute to 200°C, and then increased at the rate of 20°C/minute to a final temperature of 300°C and held for 5 minutes. The MS operating parameters were an ionization voltage of 70 eV and electron multiplier energy of 1,024 V. Injector, interface, and ion source were kept at 220, 250 and 230°C, respectively. Compound identifications were based on comparisons of their mass spectra (MS) with the MS obtained from a MS database of the National Institute for Standard Technology (NIST) (USA/Wiley, 2011).
Microbiological Assay
Broth dilution assay
The values of MICs (Minimal Inhibitory Concentrations)and MBCs/MFCs (Minimal Bactericidal/Fungicidal Concentrations) were determined by the method of broth dilution in sterile 15 mL test tubes 26, 36. In brief, an amount of 100 mL bacterial suspension (~ 5x10 CFU/mL) or yeast suspension (~ 5x10 CFU/mL) was dispensed into each tube containing 1.9 mL of culture medium (BB medium for and SDB medium for )- without or with the hydrosol at various percentages of 10, 20, 30, 40, or 50% (v/v). The tubes were then shaken at 150 rpm and incubated at 37°C for 48 hours. After incubation, 50 μL of resazurin indicator solution (0.01%) was added to each test tube. After the post incubation for 1 hour at 37°C, the change of color in each tube was assessed visually. MIC values of the hydrosol against the microbialstrains were determined as the lowest percentage values at which blue color of the indicator remained (indicating no microorganism growth) or changed from blue to slightly purple (equivalent to prominent growth inhibition). Test tubes containing bacterial or yeast suspensions, culture medium, and sterile distilled water were used as negative controls. Antibiotic amoxicillin and antifungal nystatin (as positive controls) were used to assure the reliability of the experiment results, and were similarly prepared. All bioassays were repeated three independent times and on triplicate samples.
In order to determine the MBC/MFC values, the suspensions of the tubes without the color change of resazurin in the MIC assay were taken and made into 10-fold serial dilutions. Subsequently, the dilutions (each 100 μL) were spread on the surface of Petri dishes with Brucella agar and SDA for and , respectively. The dishes were incubated at 37C for 3 days, and the growth of the microorganisms was then checked. The lowest hydrosol percentage values that showed no growth on the subcultures were determined as MBC or MFC values.
Time-killing assay
The growth curves of ATCC 43504 and ATCC 10231 treated with the hydrosol 10, 20, 30 and 40% (v/v) were established. An amount of 100 μL of the microbial suspensions was inoculated onto test tubes containing 1.9 mL of cuture medium alone (control curve) or containing 10, 20, 30 or 40% of the hydrosol. Then, the test tubes were put in the incubator at 37C with shaking (150 rpm) for 0, 6, 12, 18, 24, 36, and 48 hours of incubation. At each of the incubation times, the suspensions were taken and 10-fold serial dilutions were made. The dilutions (each 100 μL) were spread on the surface of Petri dishes with Brucella agar and SDA for and , respectively. After the 3-day incubation, colonies were counted by a plate colony count technique.
Data analysis
All bioassays were repeated three to five times in triplicate, and mean values ± SD were presented. The Bonferroni multiple-comparison method was used to test for significant differences among the treatments using GraphPad Prism 5 software (GraphPad Software, Inc., La Jolla, CA, USA).
Results
Chemical constituents of the dissolved essential oil from the fruit hydrosol
The dissolved essential oil (dEO) extracted from fruit hydrosol had a yield of 0.18% (w/w on fresh weight basis). The constituents of the dEO were identified by GC-MS and presented in
The percentage of chemical components (%) of dissolved essential oil (dEO) from the
No. | Chemical Compound | Molecular formula | Retention time (minute) | dEO (%) | Hydrosol (%) |
---|---|---|---|---|---|
1 | Methyl heptenone | C8H14O | 7.118 | 4.15 | 0.0075 |
2 | 3,4-pentadienal | C5H6O | 8.609 | 0.20 | 0.0004 |
3 | Eucalyptol | C10H18O | 8.707 | 0.85 | 0.0015 |
4 | Linalool | C10H18O | 11.691 | 4.24 | 0.0076 |
5 | Octanol acetate | C10H20O2 | 12.553 | 0.15 | 0.0003 |
6 | Isopulegol | C10H18O | 13.578 | 0.82 | 0.0015 |
7 | (R)-(+)-citronellal | C10H18O | 14.016 | 2.10 | 0.0038 |
8 | Terpinen-4-ol | C10H18O | 14.987 | 1.02 | 0.0018 |
9 | Santolinyl acetate | C12H20O2 | 15.127 | 0.09 | 0.0002 |
10 | 2,5-octadiene | C8H14 | 15.372 | 0.65 | 0.0012 |
11 | α-terpineol | C10H18O | 15.640 | 1.04 | 0.0019 |
12 | Neral | C10H16O | 17.975 | 27.12 | 0.0488 |
13 | Geranial | C10H16O | 19.348 | 32.92 | 0.0593 |
14 | 2-cyclopropyl-2-methylspiro[2.2]pentane-1-carboxylic acid | C12H14O2 | 20.009 | 8.09 | 0.0146 |
15 | -menthan-8-yl acetate | C12H22O2 | 21.380 | 8.45 | 0.0152 |
Total | 91.89 | 0.1654 |
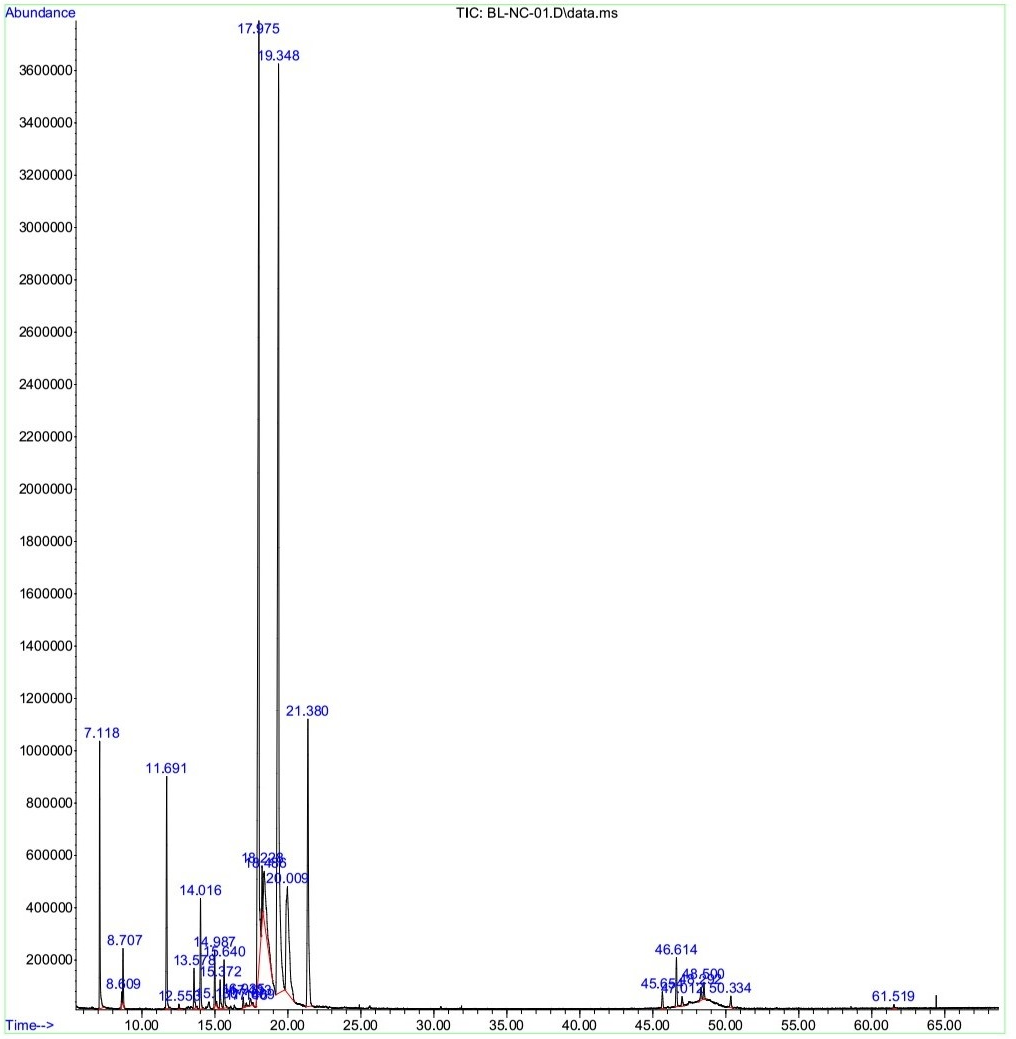
GC-MS chromatogram of the dissolved essential oil from the
Antimicrobial effects of the fruit hydrosol on and
The MIC and MBC values of the hydrosol towards the strain ATCC 43504 are shown in
MIC and MBC/MFC of the
Organism | Sample | MIC | MBC/MFC |
---|---|---|---|
H. pylori strain ATCC 43504 | Hydrosol | 10 % | 30 % |
Amoxicillin | 0.04 μg/mL | 0.10 μg/mL | |
C. albicans strain ATCC 10231 | Hydrosol | 10 % | 40 % |
Nystatin | 4 μg/mL | 16 μg/mL |
The growth-inhibiting and fungicidal effects of the hydrosol on the strain ATCC 10231 are shown in
Microbicidal time-kill of the fruit hydrosol against and
The growth curves of ATCC 43504 were performed in order to evaluate the bactericidal activity of the hydrosol over time. The results provided in Figure 2 indicated that the bacterial cells grew in a log phase from the start to 18 hours of the culture (5.92 – 8.15 LogCFU/mL) and in a stationary phase (8.48 – 8.62 LogCFU/mL) after 18 – 48 hours in the negative control culture. In the cultures with the hydrosol, the viable count of the bacteria decreased in a concentration- and time-dependent manner. The population treated with 10%, 20% and 30% of the hydrosol decreased significantly (p<0.05) compared with the negative control after 12 - 48 hours. In the cultures treated with 10% hydrosol, the strain was inhibited and could survive for 48 hours with no significant change in cell density (5.92 – 6.08 LogCFU/mL). The populations of the test strains declined significantly in the treatments of with the hydrosol (20%) with cell density ranging from 3.74 – 3.25 LogCFU/mL in comparison with 8.48 – 8.62 LogCFU/mL for the controls after 24 – 48 hours of treatment. In the cultures of 30% hydrosol, the microbial strains had sharp decreases in the viable count- from 5.92 to 2.61 LogCFU/mL after 18 hours- and completely killed after 24 hours of treatment.
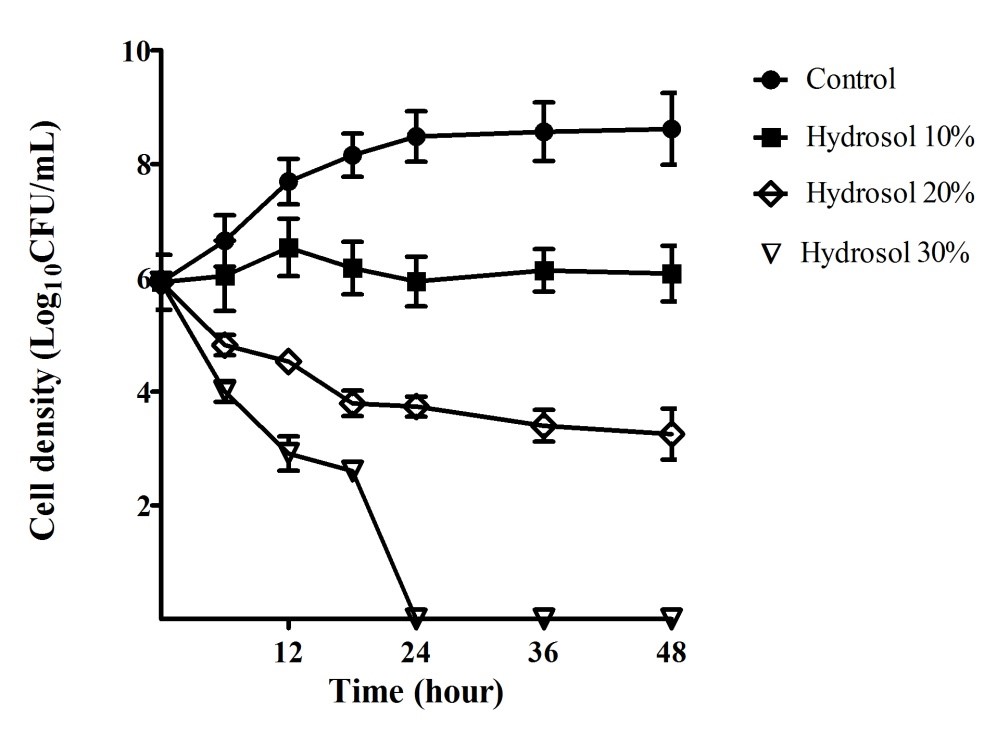
Bacterial growth inhibitory and bactericidal activities of
Fungal time-kill curves of after exposure to the hydrosol at various percentages were also determined and are shown in Figure 3. In the negative control cultures, the yeast population increased steadily (5.09 – 7.81 LogCFU/mL) during the period of 48 hours. In the cultures treated with the hydrosol, the growth of the yeast populations reduced in a concentration- and time-dependent manner. The population gradually increased (5.09 – 6.92 LogCFU/mL) for 48 hours treatment with 10% of hydrosol, but the growth was significantly slower (p<0.05) than that in the control culture at 48 h (7.81 LogCFU/mL). The population treated with 20%, 30% and 40% of the hydrosol decreased significantly (p<0.05), compared with the negative control after 12 - 48 hours. When treated with the hydrosol 20% and 30%, the number of the yeast cells had a significant fall (p<0.05) after 24 – 48 hours of treatments with cell density ranging 3.51 – 2.94 and 2.86 – 2.48 LogCFU/mL, in comparison with 7.19 – 7.81 LogCFU/mL of the controls, respectively. The exposure to the hydrosol 40% caused the yeast population to obviously drop, ranging from 5.09 to 2.60 LogCFU/mL after 12 hours and completely killed after 18 hours of treatment.
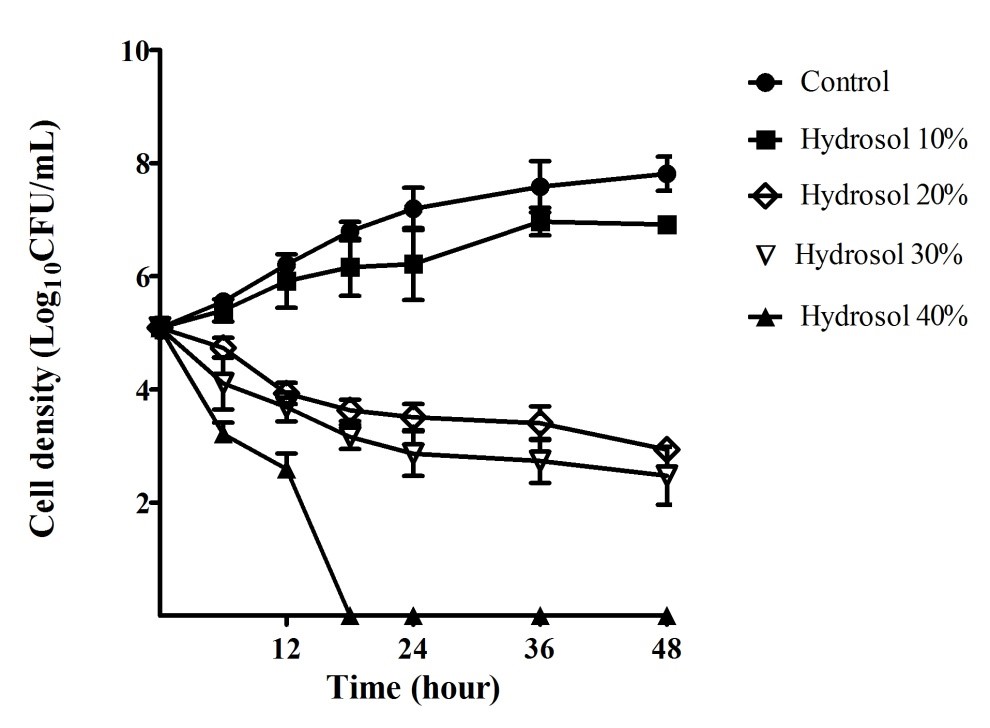
Fungal growth inhibitory and fungicidal activities of
Discussion
Hydrosols from some plants were identified chemical constituents 37, 38, 39, 40, 41, but there was no information about the composition of the fruit hydrosol. The previous studies of EOs from fruits collected in Vietnam, China, and India indicated that neral and geranial were the two main components of the EOs (66.3 – 83.9%) 42, 43, 44. Both of the two compounds were also the predominant constituents accounting for 60.04% of the dEO from the hydrosol in our study. Furthermore, other compounds, including methyl heptenone, eucalyptol, linalool, isopulegol, citronellal, terpinen-4-ol, and -terpineol, were found present in the fruit EOs in several previous studies 42, 43, 44. Interestingly, theywere also found in the hydrosol in our study herein. In addition, the dEO in the hydrosol had hydrophilic properties because it mainly contained oxygenated compounds (91.24%) yet with less hydrocarbons (0,65%), whereas the EO extracted from the fruit in Thua Thien - Hue Province, Vietnam showed a lower amount of oxygen-containing components (78.8%) and a higher amount of hydrocarbons (21%) 44. Studies on hydrosols of Indian oregano (), caraway (), bergamot-mint () also led to similar results 19, 40, 41, 45, 46.
Hydrosols have been widely used in many regions of the world for food flavoring and medicinal purposes for a long time 2, 3. Moreover, the hydrophilic property makes hydrosols safe for skin. Since hydrosols are well-tolerated by the skin, they have thus been used in cosmetic products 47. Hydrosols of several spices and aromatic plants were demonstrated to possess high antifungal and antibacterial properties against a variety of bacteria and fungi48, 49, 50, 51. For example, hydrosols extracted from cardamom (), thyme (), and cinnamon () showed the complete growth inhibition against , and 49. The hydrosol 15% (v/v) was shown to have fungicidal effects, inhibiting 100% of mycelial growth of , and However, hydrosol of exhibited weak antimicrobial activities towards tested Gram-negative bacteria ( and ) and yeast (), with MIC values of 70% (v/v); towards Gram-positive bacteria ( and ), the MIC values were greater than 90% 47. In the present study, the fruit hydrosol exhibited strong antimicrobial activities towards the bacterium (MIC of 10%, MBC of 30%) and the yeast (MIC of 10%, MFC of 40%). The cells of and were killed completely after 24 and 18 hours of treatment with the 30% and 40% hydrosol, respectively.
There have been no data of antibacterial and antifungal activities of the hydrosol extracted from the fruits, yet the information of those of the fruit EO have been well-known. The fruit EO showed strong inhibitory effects on Gram-positive bacteria (,, ) and Gram-negative bacteria ( and), and , and high growth-inhibiting activity towards methicillin-resistant . In addition, the EO exhibited good fungicidal activities against , , and . Bacteriostatic and bactericidal activities of the EO significantly depend on concentration and exposure time . The EO at concentration of 0.0625% (v/v) was able to prolong the growth lag phase of cells to approximate 12 hours, whereas the 0.125% (v/v) concentration killed the cells completely within 2 hours.
The antibacterial and antifungal effects of plant EOs and their hydrosols have been attributed to their compositions. The richness in oxygenated monoterpenes, oxygenated sesquiterpenes, and phenolic compounds contributed to the strong antimicrobial activities of hydrosols 7, 53, 54. Phenolic compounds can interfere with microbial membranes, cell walls, or the action of microbial enzymes 2. Terpenoid compounds may disrupt the lipid structure and thus cause loss of membrane integrity, membrane protein functions (proton pumps and enzymes), and synthesis of cellular metabolites, leading to cell death 31, 55. The antimicrobial effects of the fruit hydrosol may be mainly due to the presence of citral (geranial and neral) 32, 56. Neral and geranial were found to be the two main components of lemongrass EO, which was demonstrated to have strong antibacterial activity against Gram-negative and Gram-positive bacteria 57 and resulted in a considerable decrease in the density of in mouse stomach 24. Citral and sabinene were reported to exhibit strong anti- activities 58. Citral was also shown to exhibit excellent activities against dermatophytes, thereby suggesting its potency as a fungicidal agent59. Furthermore, citral showed strong anti-fungal abilities towards pathogenic microbes and, and was considered as a potential agent to control skin and mucosal infections60. The synergistic effects among major and minor components of the fruit hydrosol against and need to be further studied.
Conclusion
The present study revealed that the fruit hydrosol exhibited pronounced inhibitory and microbicidal effects against and . The results suggest that the fruit hydrosol warrants further study and could be developed as a potent antimicrobial product for treating and infections.
Abbreviations
ATCC: American Type Culture Collection
dEO: dissolved essential oil
EO: Essential oil
GC-MS: Gas chromatography-mass spectrometry
MALDI-TOF MS: Matrix-assisted laser desorption ionization-time of flight mass spectrometry
MIC: Minimal inhibitory concentration
MBC: Minimal bactericidal concentration
MFC: Minimal fungicidal concentration
Acknowledgments
Authors gratefully acknowledge the financial support from Vietnam National Foundation for Science and Technology Development () under grant number .
Author’s contributions
All authors made substantial contributions to conception and design, acquisition of data, or analysis and interpretation of data; took part in drafting the article or revising it critically for important intellectual content; gave final approval of the version to be published; and agree to be accountable for all aspects of the work.
Funding
Vietnam National Foundation for Science and Technology Development () under grant number .
Availability of data and materials
Data and materials used and/or analysed during the current study are available from the corresponding author on reasonable request.
Ethics approval and consent to participate
Not applicable.
Consent for publication
Not applicable.
Competing interests
The authors declare that they have no competing interests.