Abstract
Background: Asthma is a very common, non-communicable, chronic respiratory disease, with a high incidence rate worldwide. Currently, no permanent therapeutic strategies are available for the treatment of asthma. Drugs, such as corticosteroids, β2-agonists, and anticholinergics, only temporarily reduce symptoms and have various adverse effects. In this study, we investigated the use of fisetin, a readily available natural product found in fruits, such as strawberries and apples, to treat acute asthma in a preclinical mouse model.
Methods: We induced asthma in BALB/c mice using intraperitoneal sensitization and repeated intratracheal challenges with ovalbumin (Ova), followed by oral administration of 0.6 mg/kg (MPK) aqueous fisetin (primary stock in DMSO) before each intratracheal challenge. The mice were subsequently evaluated with whole-body plethysmography, 24 hours after the last intratracheal challenge, to measure airway hyper-responsiveness (AHR). The mice were sacrificed, and their tissues removed to evaluate a range of disease parameters.
Results: In mice treated with fisetin, AHR was significantly reduced, as shown by plethysmography. Acute inflammation associated with asthma was also reduced, as determined by inhibition of cellular infiltration, serum IgE, and serum nitric oxide (NO), as well as a reduction in goblet cell hyperplasia. We also found that fisetin potentially acts by blocking the TH2 response, including pro-inflammatory signaling molecules such as NFκB, STAT6, HIF1α, iNOS, TNFα and IL13.
Conclusion: Our study showed that a low dose of fisetin (50 nM), administered orally in an aqueous solution, may offer a safe and economical therapeutic strategy for asthma.
Introduction
Asthma is a debilitating disease, particularly if not managed in the early stages. Most over-the-counter or prescription medications, however, may result in refractory disease within a short timespan and/or are ineffective in a substantial percentage of patients. Asthma affects 334 million individuals worldwide, with up to 300,000 deaths and 22 million disability-adjusted life years lost annually1. Clinical manifestations of the disease are characterized by an infiltration of the airways with eosinophils, mast cells, and CD4+ TH2 cells2. When a susceptible individual is exposed to allergens, antigen-presenting cells (APCs) of the airways in the lungs are activated, leading to the release of pro-inflammatory cytokines, including TNFα, NFκB, chemokines, and other mediators that activate the TH2 immune response2, 3. The TH2 response subsequently activates the humoral response and the production of antibodies. Upon subsequent challenges with an allergen, the humoral response activates further TH2- and IgE‑mediated inflammation of the airways3. The airways become swollen, and the epithelial cells lining the airways are stimulated to over-produce mucus. The excess mucus is deposited along the walls of the airways, leading to their constriction and subsequent restriction in the passage of air2, 3. This airway restriction and constricted air passage leads to the characteristic symptoms of asthma, including coughing, wheezing, chest tightness, and shortness of breath.
Currently, the principal limitation in asthma treatment is the absence of a safe, permanent cure. The exact incidence rate of asthma occurrence is difficult to determine due to the lack of conclusive data4. Deaths due to asthma are often preventable, but they continue to occur as the result of poor symptom management4, 5. Under-treatment of asthma patients is common, often due to high costs, especially in low-income countries. In many countries, appropriate treatment is either unavailable, very expensive, or of unreliable quality4. In India, many people consider asthma to be a stigma and thus hide their disease4. Some patients stop taking their prescribed medication as soon as the symptoms disappear, due to the costs of treatment4, 5. In addition, some people do not respond to existing therapy.
Fisetin (3,7,3′,4′-tetrahydroxyflavone) is a polyphenol of the flavonoid group (Figure 1). It is obtained from various fruits and vegetables, such as strawberries and apples, at concentrations ranging from 2-160 µg/g, with the highest content found in strawberries6. Several studies have reported that fisetin exhibits anti-inflammatory effects by inhibiting NFκB and TNFα. Studies have also found that fisetin has anti-oxidative, anti-proliferative and anti-cancer effects6.
In light of the above-mentioned limitations of asthma therapy, we decided to test the effects of the natural compound, fisetin, in a preclinical mouse model of allergic asthma. Even though asthma is a chronic disease involving degeneration of the lungs and airways, the first stages in the onset of asthma typically involve acute inflammation. Thus, the objective of this study was to determine the anti-inflammatory effect of fisetin in an acute preclinical model of asthma. In our study, we induced asthma in mice by repeated intratracheal challenges with ovalbumin (Ova), following initial intraperitoneal sensitization. Fisetin was administered orally at a relatively low dose, and its anti-inflammatory effects were assessed with a range of assays. Plethysmography was carried out to determine the airway resistance of the mice exposed to Ova and fisetin. To our knowledge, this is the first time fisetin has been used orally at such a low dose in a preclinical model for the treatment of acute asthma.
Methods
Ethical approval
All experiments were performed according to the rules established by the institutional and departmental animal ethics committee. The animals were housed under specific pathogen-free conditions at the animal house of the Department of Zoology, University of Calcutta.
Mice
6-week-old female BALB/c mice, weighing 20 – 25 g, were divided into three groups, with n = 4 for each group:
Induction of disease & treatment with fisetin
Mice in the Ova and Ova+F groups were sensitized with an intraperitoneal injection of 100 µg Ova (Sigma Aldrich), with aluminum hydroxide (Al3OH) (Sigma Aldrich) as an adjuvant, on day 07, 8, 9. The mice were subsequently challenged intratracheally with 250 µg Ova on day 8, and 125 µg Ova on days 15, 18, and 219, 10. Intratracheal administration was performed after anesthetizing the mice with isoflurane in an anesthesia chamber. Mice in the control group were given normal saline.
One hour before each intratracheal administration of Ova, mice in the Ova+F group were administered 50 nM of fisetin (corresponding to 0.6 mg/kg [MPK]) orally. Fisetin was obtained from the Cellular Neurobiology Laboratory, Salk Institute for Biological Studies, USA. A stock solution of 1 mg/ml fisetin (equivalent to 3.5 mM) was initially prepared in DMSO, and subsequently diluted with water to obtain the final aqueous solution of 50 nM fisetin. This dose was chosen based on previous in vitro studies, in which the most significant anti-inflammatory effect was found at this concentration. Figure 2 shows a diagrammatic representation of the treatment regimen.
Measurement of airway hyper-responsiveness (AHR)
The mice were exposed to unrestrained, whole-body plethysmography, which measured the enhanced pause (Penh) between each inhalation and exhalation. This measurement provided information about airway resistance in mice from the three groups in response to increasing concentrations of methacholine (Mch). On day 22, plethysmography was performed 24 hours after the last intratracheal treatment8, 11. The double-chambers of the plethysmograph (emka Technologies, France) were initially calibrated with 5 ml air, and the mice from each group were placed in the chamber for 10 min to acclimatize before the start of the experiment. The mice were then exposed to increasing concentrations (0 mg/ml, 5 mg/ml, 10 mg/ml, 15 mg/ml, and 20 mg/ml) of Mch (TCI, Japan) in phosphate-buffered saline (PBS) for 1 min, followed by recording the Penh for 4 min after each challenge.
Sacrifice and collection of tissues
After plethysmography, the mice were sacrificed by cervical dislocation, and the following tissues were collected for various assays:
Peripheral blood (PB)
PB was obtained by cardiac puncture and collected in K2-EDTA tubes (BD Biosciences, USA). For differential cell counts, a smear was prepared on a microscope slide.
Serum
PB was collected in tubes (without EDTA, to allow for coagulation), and allowed to stand for at least 30 min. It was subsequently centrifuged at 10,000 rpm for 10 min at 4°C12, and the supernatant collected in fresh tubes. The serum was stored at –80°C for later use.
Bronchoalveolar lavage fluid (BALF)
BALF was collected from both lungs by injecting 1 ml of cold PBS slowly into the lungs, through the trachea, and drawing out the resultant solution13. BALF was centrifuged at 200 x g for 10 min at 4°C. The cell pellet was resuspended in media for assays and a smear was made on a microscope slide for differential cell count.
Lung parenchyma
Immediately after collecting BALF, the lungs were washed with PBS. For the assays, one lung was placed in a Petri dish and macerated into small pieces. The lung tissue was digested using a 1X collagenase/hyaluronidase cocktail (Stem Cell Technology) overnight at 37°C. The digested tissue was subsequently filtered through a cell strainer to obtain a single-cell suspension for total cell (TC) count, CFU assay, and immunophenotyping. For gene expression studies, whole lung was stored in RNAlater solution (Ambion, Inc.) at –80˚C. For protein expression studies, whole lung was removed, washed with PBS, and stored at –80˚C. Whole lung was kept in 10% buffered formalin for histological studies.
Bone marrow (BM)
The femur bones were collected, the ends were cut, and the cells flushed out with fresh media for TC count and collected in 1X PBS for flow cytometry.
TC count
A PB/BALF/lung/BM suspension was mixed with an equal volume of Trypan Blue dye (Himedia, India), and cell viability was determined using a hemocytometer.
Differential cell (DC) count
The PB/BALF smears were air-dried, fixed with methanol (SRL, India), stained with Geimsa stain (SRL, India) for 15 min, washed, and viewed under a light microscope (Dewinter Fluorex LED) at 40X magnification14. Cell types were distinguished based on their nuclear morphology.
Immunophenotyping
100 µl of PB (pooled from 4 mice) was mixed with an equal volume of antibody cocktail and incubated in the dark for 15 min. A total of 4 ml of 1X RBC lysis buffer (Himedia, India; diluted in distilled water) was added, incubated for 20 min, and centrifuged at 1500 rpm for 5 min. The pellet was washed in 2 ml of FACS buffer (PBS + 10% FBS + 0.3% sodium azide) and centrifuged at 1500 rpm for 5 min. The pellet was finally resuspended in PBS for analysis.
Digested lung and BM cells were strained, washed in FACS buffer, and stained with an antibody cocktail for 15 min. The stained cells (pooled from 4 mice) were centrifuged at 1500 rpm for 5 min, and the pellet was resuspended in PBS for analysis.
Cell surface staining was performed using the following antibodies: CD45 for hematopoietic cells (conjugated with PerCP/Cy5.5; BioLegend), CD3e for T cells (conjugated with PE; BD Biosciences, USA), CD4 for helper T (TH) cells (conjugated with V450; BD Biosciences, USA), CD8a for cytotoxic T (TC) cells (conjugated with Alexa Fluor 488; BD Biosciences, USA), B220 for B cells (conjugated with FITC; BD Biosciences, USA), GR-1 for neutrophils (conjugated with FITC; MACS), and F4/80 for macrophages and monocytes (conjugated with PE; Invitrogen). Flow cytometry was performed on BD FACSVerse (BD Biosciences, USA), and the results were analyzed using FACSSuite software (BD Biosciences, USA).
Estimation of serum IgE
The concentration of total IgE in the serum was determined using ELISA, according to the datasheet (BD OptEIA Mouse IgE ELISA Set), with some modifications. The wells of a 96-well, high-binding flat bottom plate (Corning, USA) were coated with 100 µl of serum sample (pooled from 4 mice), diluted 1:10 in sterile PBS, and incubated overnight at 4°C. The following day, the wells were washed three times with PBST (PBS + 0.05% Tween 20), and then blocked with 200 µl of blocking buffer (1% BSA in PBS) for 30 min at room temperature. The wells were washed four times with PBST, after which 100 µl of 2 µg/ml biotin-conjugated rat anti-mouse IgE (BD Biosciences), diluted in blocking buffer, was added to each well and incubated for 1 hour at room temperature. The wells were washed four times with PBST and blotted dry. A total of 100 µl of 1:1000 avidin-HRP (BD Biosciences), diluted in blocking buffer, was added to each well and incubated at room temperature for 30 min in the dark, then washed four times with PBST and blotted dry. A total of 100 µl of TMB substrate (3, 3′, 5, 5′-tetramethylbenzidene; Sigma Aldrich) was added to each well and incubated for 10 min at room temperature in the dark. The reaction was stopped with 50 µl of 1M phosphoric acid (Merck, India), and absorbance was measured at 405 nm in an ELISA plate reader (ThermoFisher Scientific). The concentration of IgE in each well was calculated with a standard curve prepared using purified IgE (BD Biosciences, USA).
Nitric oxide (NO) assay
100 µl of PB/BALF/lung suspension (pooled from 4 mice) was seeded in the wells of a 96-well plate (Tarsons, India). To each well, 100 µl of Griess reagent (1% sulfanilamide [SRL, India] + 0.1% NED [SRL, India] in 5% o-phosphoric acid [Merck, India]; prepared at least a day before) was added and incubated for 10 min at room temperature in the dark. Absorbance was measured at 540 nm in a multi-well plate reader (ThermoFisher Scientific). The concentration of NO in each sample was determined from a standard curve.
Gene expression by reverse transcriptase PCR (RT-PCR)
Total RNA was extracted from lung tissues with TRIzol reagent according to the manufacturers’ instructions (Life Technologies, USA). The lungs of mice from each treatment group were homogenized in 1 ml TRIzol reagent and incubated at room temperature for 5 min. Subsequently, 200 µl chloroform (Merck, USA) was added, the tubes were shaken vigorously for 15 seconds, incubated at room temperature for 2-3 min, and centrifuged at 12,000 x g for 15 min at 4°C. The upper aqueous layer was carefully transferred to new tubes. A total of 500 µl of chilled, 100% isopropanol (Amresco) was added, and the tubes were incubated overnight at –20°C. The tubes were centrifuged at 12,000 x g for 10 min at 4°C. The supernatants were discarded, and the gel-like pellets were washed with 750 µl of chilled, 75% ethanol (Merck, USA), vortexed briefly, and centrifuged at 7500 x g for 5 min at 4°C. The pellets were air-dried and resuspended in RNase-free water. The concentration of RNA in each sample was measured using a Nano-spectrophotometer (Jenway, UK), and the purity was assessed by the ratio of the absorbance at 260 nm to absorbance at 280 nm.
A total of 1 µg RNA from each sample was used to prepare cDNA, using the ThermoFisher Scientific Verso cDNA Synthesis kit. A master mix, containing 1X cDNA synthesis buffer, dNTP mix (with 500 µM of each nucleotide), random hexamer primer, RT enhancer, and Verso enzyme mix (containing the reverse transcriptase enzyme), was prepared. To this mixture, 1 µg of the RNA was added, and the volume was made up to 20 µl with nuclease-free water. The cDNA was prepared using the cycle shown in Table 1 below.
Temperature | Time | No. of cycles | Purpose |
---|---|---|---|
42˚C | 30 mins | 1 cycle | cDNA synthesis |
95˚C | 2 mins | Inactivation of enzymes |
The cDNAs prepared were added to the PCR reaction mixture, containing 1X PCR buffer (Invitrogen), 1.5 mM MgCl2 (Invitrogen), dNTPs (Invitrogen), 0.5 µg each of forward and reverse gene-specific primers (Eurofins), and Taq polymerase (Invitrogen), and amplified by PCR (Applied Biosystems, USA), using the protocol shown in Table 2.
Temperature | Time | No. of cycles |
---|---|---|
95˚C | 5 mins | 1 cycle |
95˚C | 45 secs | 30 cycles |
T˚C | 30 secs | |
72˚C | 45 secs | |
72˚C | 10 mins | 1 cycle |
4˚C | hold |
The sequence and annealing temperature (T°C) of each primer is provided in Table 3. Gene expression of inflammatory cytokines, such as iNOS and TNFα, and TH2 cytokines, such as IL‑13, were assessed, with GAPDH as the housekeeping gene. PCR products were run in 1% agarose gels (G-Biosciences, USA), with a 100 bp marker (100 – 2000 bp; GeNet Bio, Korea) to detect the expression of inflammatory genes. Bands were observed under UV light in a gel-doc system (BioRad), and the intensities of the bands were determined with ImageJ software.
Gene | Primer sequence | Tm | |
---|---|---|---|
GAPDH | F | 5’- GAGGGGCCATCCACAGTCTTC | 63.73˚C |
R | 5’- CATCACCATCTTCCAGGAGCG | 61.78˚C | |
IL-13 | F | 5’- CGGCAGCATGGTATGGAGTG | 61.40˚C |
R | 5’- ATTGCAATTGGAGATGTTGGTCAG | 59.30˚C | |
iNOS | F | 5’- AATGGCAACATCAGGTCGGCCATCACT | 66.48˚C |
R | 5’- GCTGTGTGTCACAGAAGTCTCGAACTC | 66.48˚C | |
TNFα | F | 5’- GGCAGGTCTACTTTGGAGTCATTGC | 64.62˚C |
R | 5’- ACATTCGAGGCTCCAGTGAATTCGG | 64.62˚C |
Protein expression by Western blot
Lungs were homogenized, and the total cellular protein was extracted in RIPA buffer containing Tris (pH 7.4) (Amresco), 150 mM NaCl (SRL, India), 1% Triton-X-100 (SRL, India), 0.5% sodium deoxycholic acid (SRL, India), 0.1 % SDS (Merck, USA), 1mM EDTA (SRL, India), 10 mM sodium fluoride (SRL, India), 1 mM PMSF (SRL, India) and 1X protease inhibitor cocktail (Cell Signaling Technology, USA). Cell lysate was incubated at 4°C for 2 hours with continuous stirring, and residual tissue was removed by centrifugation at 10,000 rpm at 4°C for 20 min. Supernatant was removed and stored at –80°C for future use. Total protein in the supernatant was estimated by Bradford reagent (Himedia, India). The concentration of total lung protein was determined from a standard graph prepared using BSA. Equal amounts (40 µg) of lung protein were run in a 12% SDS gel with a pre-stained marker (10 – 250 kDa; BioRad) and transferred onto a PVDF membrane (Merck, USA). The membrane was blocked with 5% nonfat dried milk in PBST for 2 hours at room temperature, hybridized overnight with the primary antibody (1:100 rabbit anti-mouse NFκB [Santa Cruz], 1:100 rabbit anti-mouse NOS2 [Santa Cruz], 1:100 rabbit anti-mouse STAT6 [Santa Cruz], 1:100 rabbit anti-mouse HIF1α [Santa Cruz] and 1:1000 rabbit anti-mouse GAPDH [Santa Cruz]) at 4°C, and incubated with the secondary antibody (1:5000 goat anti-rabbit IgG-HRP [Santa Cruz]) at room temperature for 2 hours. The membrane was washed with PBST between hybridizations. The blot was developed using Western ECL substrate (BioRad) and visualized using a chemidoc system (Azure Biosystems C400). The intensities of the bands were determined using ImageJ software.
Histology
Lung tissues were fixed with 10% formalin at room temperature for 72 hours. Tissues were first dehydrated by passing them through a series of increasing concentrations of ethanol, beginning with 50% and progressing in graduated steps to 100%. The tissues were subsequently treated with xylene, embedded in paraffin and cut into 5 µm sections in a rotary microtome. The amount of cellular infiltration around pulmonary blood vessels was assessed by hematoxylin and eosin staining. Mucus-containing goblet cells were visualized by Alcian blue staining, and goblet cell hyperplasia determined. The stained sections were observed under a light microscope (Dewinter Fluorex LED) at 4X, 10X and 40X magnifications.
CFU-c assay
A colony-forming unit-cell (CFU-c) assay was performed using a semisolid medium to evaluate the clonogenic potential of the tissue cells (i.e., the ability of the cells to grow and proliferate into colonies). The methylcellulose semisolid media was prepared with IMDM (Himedia, India), 30% FBS (Himedia, India), 20 mg/ml BSA (Biosera), 1% Pen-Strep (Himedia, India), and 5 ng/ml stem cell factor (Biovision). All constituents, except methyl cellulose, were added to a 50 ml centrifugation tube and vortexed. Finally, 1.5% methyl cellulose (Himedia, India) was added to this mixture, vortexed to mix completely, and 1 ml of the semisolid medium was added to the wells of a 24-well plate (Merck Millipore). A total of 500 µl of PB/ BALF/ lung-cell suspension (pooled from 4 mice) was added to each well according to treatment group and incubated at 37°C in a 5% CO2 humidified chamber (ThermoFisher Scientific) for 7 days. The total number of colonies formed in each sample was counted, and the clonogenic potential of the samples was calculated by dividing the number of colonies obtained by the total number of cells plated.
Statistical analysis
All data obtained from the three independent experiments were analyzed with GraphPad Prism 6. Data are represented as Mean ± SEM, and p-values less than 0.05 are considered to be significant. Statistical significance was calculated by t-test in GraphPad Prism 6. An asterisk (*) was used to denote significance with respect to control samples, and a hashtag (#) was used to denote significance in comparison to Ova-treated samples.
Results
Plethysmography
With increasing concentration of Mch (0 mg/ml, 5 mg/ml, 10 mg/ml, 15 mg/ml and 20 mg/ml), the Penh value of the Ova-treated mice increased, by 1.62-fold, 2.06‑fold, 2.45-fold (p < 0.05), 2.70-fold (p < 0.05) and 2.52-fold (p < 0.05), respectively, compared to untreated control (Figure 3). Fisetin reduced the airway resistance by 1.20-fold, 1.25-fold, 1.91‑fold, 1.84-fold and 1.20-fold, with respect to Ova (Figure 3).
TC of blood, lung, BALF & BM
Upon treatment with Ova, the TC count of blood, BALF, lung, and BM increased, indicating the establishment of inflammation. The TC of blood increased by 2.78-fold (p < 0.05) with Ova, compared to control (Figure 4 A). The TC count of PB decreased by 1.83-fold (p < 0.05) with fisetin treatment (Figure 4 A). The TC count of lung (Figure 4 B) and BALF (Figure 4 C) increased by 2.34-fold (p < 0.05) and 41.40-fold (p < 0.05), respectively, with Ova. This observation indicates there was an infiltration of cells into the sites of inflammation. Fisetin successfully inhibited this infiltration in both lungs (Figure 4 B) and BALF (Figure 4 C), by 1.31‑fold (p < 0.05) and 2.01-fold (p < 0.05), respectively. Compared to the control, the TC count in BM (Figure 4 D) increased by 2.66-fold (p < 0.05) with Ova treatment and was inhibited significantly with fisetin treatment by 1.62-fold (p < 0.05). The observed increase in the TC count in BM is an indication of increased production of immune cells in response to the antigen.
Differential count of eosinophils in the blood and BALF
Upon treatment with Ova, which induces eosinophilic asthma, an increase in eosinophils in blood and BALF was observed. The percentage of eosinophils in blood (Figure 6 A) and BALF (Figure 6 B) increased significantly by 5.76-fold (p < 0.05) and 8.40-fold (p < 0.05), respectively, with Ova treatment, compared to the control. In contrast, fisetin treatment led to a decrease in the percentage of eosinophils by 2.43‑fold (p < 0.05) in blood (Figure 6 A), and by 1.29-fold in BALF (Figure 6 B).
Immunophenotyping of immune cells in the blood, lungs, and bone marrow
In blood, Ova increased CD45+B220+ B cells (Figure 7 A) by 1.67-fold (p < 0.05), CD45+CD3+ T cells (Figure 7 A) by 2.26-fold (p < 0.05), CD45+CD3+CD4+ TH cells (Figure 7 A) by 2.27-fold (p < 0.05), CD45+CD3+CD8+ TC cells (Figure 7 A) by 2.31-fold (p < 0.05), CD45+GR1+ neutrophils (Figure 7 B) by 3.58-fold (p < 0.05), and CD45+F4/80+ macrophages and monocytes (Figure 7 B) by 9.74-fold (p < 0.05). Fisetin reduced B cells (Figure 7 A) by 1.27-fold (p < 0.05), TH cells (Figure 7 A) by 1.14-fold (p < 0.05), neutrophils (Figure 7 B) by 1.17-fold and F4/80+ macrophages and monocytes (Figure 7 B) by 1.48-fold (p < 0.05). Fisetin, however, increased the number of CD3+ T cells (Figure 7 A) by 1.06-fold and CD8+ TC (Figure 7 A) cells by 1.24-fold (p < 0.05) in blood.
In the lung, Ova increased CD45+B220+ B cells (Figure 8 A) by 19.27-fold (p < 0.05), CD45+CD3+ T cells (Figure 8 A) by 13.25-fold (p < 0.05), CD45+CD3+CD4+ TH cells (Figure 8 A) by 15.38-fold (p < 0.05), CD45+CD3+CD8+ TC cells (Figure 8 A) by 11.07-fold (p < 0.05), CD45+GR1+ neutrophils (Figure 8 B) by 3.05-fold (p < 0.05) and CD45+F4/80+ macrophages and monocytes (Figure 8 B) by 4.18-fold (p < 0.05). Fisetin reduced B cells (Figure 8 A) by 1.92-fold (p < 0.05), T cells (Figure 8 A) by 2.04-fold (p < 0.05), TH cells (Figure 8 A) by 2.36-fold (p < 0.05), TC cells (Figure 8 A) by 1.75‑fold (p < 0.05), neutrophils (Figure 8 B) by 8.02-fold (p < 0.05), and F4/80+ macrophages and monocytes (Figure 8 B) by 1.21-fold. Ova treatment led to a 1.39-fold (p < 0.05) increase in the ratio of CD4+/CD8+ cells, indicating an increased influx of CD4+ TH cells under asthmatic conditions (Figure 8 C). This ratio was reduced by 1.35-fold (p < 0.05) with fisetin treatment (Figure 8 C).
In bone marrow, Ova treatment also led to a significant increase in immune cells, indicating an upsurge in the production of these cells in response to the antigen. A 2.34-fold (p < 0.05) increase in B cells (Figure 9 A), a 4.00-fold (p < 0.05) increase in T cells (Figure 9 A), a 4.25-fold (p < 0.05) increase in CD4+ TH cells (Figure 9 A), a 3.00-fold (p < 0.05) increase in CD8+ TC cells (Figure 9 A), a 4.21-fold (p < 0.05) increase in the neutrophils (Figure 9 B) and a 6.42-fold (p < 0.05) increase in monocytes and macrophages (Figure 9 B) was observed. Fisetin significantly reduced the number of B cells by 2.24-fold (p < 0.05) (Figure 9 A), T cells by 2.59-fold (Figure 9 A), TH cells by 2.13-fold (p < 0.05) (Figure 9 A), TC cells by 3.00-fold (Figure 9 A), neutrophils by 1.80-fold (Figure 9 B), and macrophages by 2.56-fold (p < 0.05) (Figure 9 B).
Estimation of total IgE in serum
Elevations in serum IgE levels are a characteristic feature of eosinophilic asthma. This finding was confirmed in our results, where the level of serum IgE rose by 1.68-fold (p < 0.05) with Ova treatment, as compared to the control (Figure 10 ). Fisetin successfully restored serum IgE to nearly control levels, by 1.33-fold (p < 0.05), compared to Ova (Figure 10 ).
Estimation of total NO concentration in the blood, BALF, and lung
NO is an inflammatory marker, and Ova treatment led to a 2.32-fold (p < 0.05) increase of NO concentration in the blood (Figure 11 A), a 1.39-fold (p < 0.05) increase in the lung (Figure 11 B), and a 3.57-fold increase in BALF (Figure 11 C). Fisetin reduced NO concentration in all three tissues, by 1.84‑fold (p < 0.05) in the blood (Figure 11 A), 1.13-fold (p < 0.05) in the lung (Figure 11 B), and 1.01‑fold in BALF (Figure 11 C).
Estimation of expression of pro-inflammatory cytokines and signaling molecules at the gene level
We assessed the gene expression of pro-inflammatory cytokines and signaling molecules, such as IL13, TNFα, and iNOS, by RT-PCR. We used GAPDH as the housekeeping gene, the expression of which did not change with either treatment (Figure 12 A). On the other hand, expression of the genes under study increased significantly with Ova treatment, compared to control, as determined by the intensities of the bands on the gels. With Ova treatment (lanes marked O in Figure 12 A), the expression of IL13, TNFα, and iNOS increased by 18.69-fold (p < 0.05), 12.40-fold (p < 0.05), and 7.87-fold (p < 0.05), respectively (Figure 12 B), compared to control (lanes marked C in Figure 12 A). With fisetin treatment (lanes marked O+F in Figure 12 A), the expression of IL13, TNFα, and iNOS decreased by 17.54-fold (p < 0.05), 10.87-fold (p < 0.05), and 3.66-fold (p < 0.05), respectively (Figure 12 B). These findings suggest a down regulation in the expression of inflammatory molecules.
Estimation of expression of pro-inflammatory cytokines and signaling molecules at the protein level
We also assessed the protein expression of pro-inflammatory cytokines and signaling molecules like NFκB (molecular weight 65 kDa), HIF1α (molecular weight 132 kDa), NOS2 (molecular weight 130 kDa) and STAT6 (molecular weight 119 kDa). We used GAPDH (molecular weight 37 kDa) as the housekeeping protein, whose expression did not change with any treatment (Figure 13 A). On the other hand, the expression of the proteins under study increased significantly with Ova treatment, compared to control, as determined by the intensities of the bands on the blots. With Ova treatment (lanes marked O in Figure 13 A), the expression of NFκB, HIF1α, NOS2 and STAT6 increased by 4.34-fold (p < 0.05), 3.79-fold (p < 0.05), 3.27-fold (p < 0.05) and 3.66-fold (p < 0.05), respectively (Figure 13 B), compared to control (lanes marked C in Figure 13 A). With fisetin treatment (lanes marked O+F in Figure 13 A), the intensities of NFκB, HIF1α, NOS2 and STAT6 decreased by 2.98-fold (p < 0.05), 2.92-fold (p < 0.05), 1.13-fold and 2.02-fold (p < 0.05), respectively (Figure 13 B). This finding suggests there was a successful down regulation in the expression of the pro-inflammatory molecules.
Goblet cell hyperplasia in the airways of the lungs
In asthma, the goblet cells, which are mucus-producing epithelial cells of the lungs and airways, produce excessive amounts of mucus, leading to the swelling of the cells. This metaplasia is a marker of asthma. Our study showed that Ova treatment led to a 2.97-fold (p<0.05) increase in the percentage of metaplastic goblet cells (Figure 14 B, Figure 14 E, Figure 14 G), as compared to the control (Figure 14 A, Figure 14 D, Figure 14 G). Fisetin treatment led to a 1.54-fold (p < 0.05) reduction in the percentage of metaplastic goblet cells (Figure 14 C, Figure 14 F, Figure 14 G), confirming the efficacy of fisetin in reducing the symptoms of asthma.
Estimation of clonogenic potential of cells
Clonogenic potential is the ability of cells of a tissue to proliferate and form colonies. In cases of inflammation or degeneration, the cells lose their ability to proliferate to their full potential. This effect was observed in the Ova-treated groups, where the clonogenic potential of blood (Figure 15 A), lung (Figure 15 B) and BALF (Figure 15 C) decreased by 6.16-fold (p < 0.05), 4.57-fold (p < 0.05), and 110.18-fold (p < 0.05), respectively. Fisetin, in contrast, restored clonogenic potential to a moderate degree. The clonogenic potential of blood (Figure 15 A), lung (Figure 15 B) and BALF (Figure 16 C) increased by 2.95-fold (p < 0.05), 2.31-fold (p < 0.05), and 5.07-fold (p < 0.05), respectively, suggesting that fisetin treatment as potential pro-regenerative effects.
Discussion
Asthma is a chronic respiratory disorder that affects a substantial fraction of the global population. Over 15% of deaths worldwide are caused by asthma4. Currently, the primary limitation in asthma therapy is the lack of a permanent cure with no adverse effects. Current therapeutic strategies include the use of corticosteroids, long- and short-acting β2-agonists and anticholinergics, all of which have a variety of adverse effects15, 16. Moreover, none of these strategies are permanent cures, and only provide temporary relief to patients. Management of the disease, especially in under-developed countries, is poor, due to the high cost or low availability of therapy4, 5. In addition to these factors, many patients do not respond, or become refractory (i.e., develop non-responsiveness), to existing therapies and/or react to the adverse side effects (contra-indications) of prolonged use of steroids and long-acting bronchodilator (LABA) inhalers and short-acting beta agonists (SABAs).
With these limitations in mind, we designed this study to assess the efficacy of fisetin, an easily available natural compound, as a treatment for asthma in a preclinical mouse model. Previous studies have shown that fisetin possesses anti-oxidative, anti-proliferative and anti-inflammatory activity6. Although fisetin has been investigated in previous studies, the novelty of our study lies its non-invasive approach, ease of administration, and dosing. Previous studies have utilized fisetin at doses starting from 1 MPK and as high as 50 MPK, in a vehicle of DMSO11, 17, 18, 19. In our study, we used a significantly lower dose of 0.6 MPK fisetin in an aqueous solution. In addition, fisetin was orally administered, as opposed to the intratracheal, intravenous, or intraperitoneal administrations used in previous studies11, 17, 18, 19. Oral administration provides an easier form of treatment and acts on the disease in a systemic manner.
In our study, we induced asthma in BALB/c mice with ovalbumin (Ova) for 21 days. The mice were sensitized with 100 µg Ova on day 0, followed by repeated challenges with 250 µg Ova on day 8 and 125 µg Ova on days 15, 18, and 21. Assessment of lung function 24 hours after the last Ova challenge showed significantly higher Penh (i.e., the enhanced pause between inhalation and exhalation), in response to increasing concentrations of methacholine. This observation suggests that repeated challenges with Ova led to increased AHR, which is a characteristic symptom of asthma. Fisetin reduced AHR to nearly control levels (Figure 3), suggesting that it was successful in reversing the airway constriction that occurs in asthma.
After sacrificing the mice, their tissues were collected for a variety of assays. The TC count of PB, lung, and BALF increased significantly with Ova treatment, in addition to eosinophilic infiltration in the blood and BALF, and the serum IgE levels. The TC count of PB, lungs, BALF and BM (Figure 4), the eosinophil count of PB and BALF (Figure 6), and level of serum IgE (Figure 10) all decreased significantly with fisetin treatment. Hematoxylin and eosin staining of lung sections also showed reduced cellular infiltration in the lungs with fisetin, compared to Ova-treated lungs (Figure 5). Furthermore, fisetin significantly reduced the infiltration of CD45+B220+ B cells, CD45+CD3+ T cells, CD45+CD3+CD4+ TH cells, CD45+CD3+CD8+ TC cells, CD45+GR1+ neutrophils and CD45+F4/80+ macrophages in the blood, lungs, and bone marrow, which had increased with Ova treatment (Figure 7, Figure 8, Figure 9). In addition, the ratio of CD4+ and CD8+ cells in the lungs was significantly downmodulated by fisetin (Figure 8 C). The concentration of NO, an inflammatory marker, increased significantly in all three tissues from the mice treated with Ova, which decreased significantly with administration of fisetin (Figure 11). Alcian blue staining of lung sections showed a reduction in the number of mucus-containing goblet cells along the epithelia of the lungs (Figure 14). These observations indicate the anti-inflammatory effects of fisetin in asthma.
Fisetin also restored clonogenic potential (i.e., the ability of cells to form colonies) of cells in the blood, lungs, and BALF, which had been significantly reduced with Ova treatment (Figure 15). The ability of fisetin to restore the clonogenic potential of cells in various tissues suggests that it has regenerative effects.
Studies have shown that, on encountering an antigen, the macrophages of the airways and lungs are activated through toll-like receptors (TLRs), pathogen-associated molecular patterns (PAMPs) and damage-associated molecular patterns (DAMPs)20, 21, 22. These macrophages either release cytokines, such as IL25 and IL33, activate signaling molecules including TNFα and NFκB, or endocytose and destroy the antigen themselves20, 21, 22. The released cytokines and signaling molecules subsequently induce the differentiation of naïve T cells into the TH2 phenotype, which release inflammatory cytokines, such as IL4, IL5 and IL1320, 21, 22. IL4 and IL13 further activate the TH2 pathway via STAT6, induce B cells to produce antibodies, induce goblet cells to over-express mucus, and activate additional monocytes and macrophages20, 21, 22. IL5 stimulates the increased production of eosinophils in the lungs and signals the bone marrow DCs to increase the production of CD3+ T cells20, 21, 22. These CD3+ cells in turn induce the increased production of macrophages in the BM, which subsequently move into the lungs and differentiate into alveolar macrophages20, 21, 22. CD3+ cells also differentiate into TH2 cells and increase the production of IL5, which induces eosinophilia in bone marrow, and their movement back to the lung, through the action of TNFα20, 21, 22.
Earlier studies have found that asthma in general, as well as that induced by Ova, is regulated by key signaling molecules, of which TNFα and NFκB play important roles. TNFα is produced by macrophages upon activation of certain membrane-bound pattern-recognition molecules and is a major cytokine of the innate immune response23, 24. After being cleaved and activated by the TNFα-converting enzyme, TNFα binds to its receptor, leading to the activation of intracellular signaling cascades23, which in turn leads to phosphorylation and subsequent nuclear localization of the p50-p65 subunits of NFκB23, 24. The active NFκB then promotes the transcription of other pro-inflammatory genes of the TH2 response, including those of IL4 and IL13, which, in turn, activate genes like STAT6, iNOS and HIF1α25, 26, 27. NFκB also activates the transcription of more TNFα in a feedback loop23. TNFα is also involved in the interaction between mast cells and smooth muscles and, therefore, in the development of AHR23, 24. In addition, TNFα plays a major role in attracting eosinophils and T cells to the lungs23. Asthma leads to hypoxic conditions and increased production of NO in the tissue, which is reflected in the over expression of HIF1α and iNOS, respectively. In a state of inflammation, hypoxic conditions prevail, as a result of low oxygen availability due to damaged vasculature and increased infiltration of immune cells28. In allergic asthma, HIF1α promotes inflammation and stimulates the infiltration of eosinophils28.
Figure 16 shows a diagrammatic representation of the sequence of events by which Ova induces acute asthma. Our analysis of protein expression by Western blot, and gene expression by RT-PCR, confirmed the anti-inflammatory effects of fisetin. We found that fisetin significantly inhibited the expression of TNFα, IL13, and iNOS genes (Figure 12), and the expression of NFκB (p65 subunit), STAT6, HIF1α, and NOS2 proteins (Figure 13). Based on our observations, we hypothesized that fisetin exerts its principal effects in one the following ways:
Whether by path (i), (ii), or (iii), fisetin reduces inflammation, AHR, and other symptoms characteristic of asthma, including the release of inflammatory mediators, such as IgE and NO (Figure 16). Thus, fisetin could also potentially maintain mitochondrial function in the presence of oxidative stress as is evidenced by the potential mechanism outlined in Figure 16.
This study highlights the potential for asthma to be treated with natural compounds like fisetin, even when administered orally and at a significantly lower dose than that previously studied. Fisetin was found to reduce the acute inflammation that occurs in the first stages of asthma. Fisetin does not appear to have any adverse effects or toxicities when used long-term, as we also found in a separate study that fisetin was effective in a mouse model of chronic asthma29. The present study investigates the effect of fisetin on the sudden and severely acute, inflammatory phase of asthma, which, over time and without proper treatment, may develop into the chronic phase of the disease, involving tissue remodeling. The effect of fisetin in chronic asthma was investigated by Paul et al.29. We can surmise that, while the inflammation may be similar in the early stages, because the causative agent is the same, the subsequent downstream phenomenon, including target cells and the signals, in which the disease plays out, are different.
The anti-inflammatory, anti-oxidative, anti-proliferative and anti-cancer effects of fisetin are well-documented6, 10. In addition to the present study, we have investigated the effects of fisetin in several preclinical disease models, including peritonitis30, 31, idiopathic pulmonary fibrosis32, 33, and atopic dermatitis, with positive results. In the present study, fisetin was repurposed to elucidate its potential role in reversing allergic inflammation in the acute stage of disease, while in a previous study, its effects in the chronic phase were explored29, in which the key players and signaling pathways differ widely. As previously mentioned, the key events in the reversible, acute phase of asthma are eosinophilic infiltration, IgE, mucus hypersecretion by goblet cells, and AHR, with NFκB and TNFα guiding the underlying mechanisms. On the other hand, the chronic phase involves fibrosis and tissue remodeling, smooth muscle hypertrophy, epithelial cell damage, and collagen deposition, most of which are guided by the effects of transforming growth factor beta (TGFβ). TGFβ has been implicated in the induction of apoptosis of airway epithelial cells, mucus hypersecretion through stimulation of goblet cell proliferation, induction of proliferation and differentiation of fibroblasts and synthesis of extracellular matrix proteins, and tissue remodeling through the production and release of vascular endothelial growth factor (VEGF) and plasminogen activator inhibitor34, 35, 36. All these factors contribute to the swelling and obstruction of the airways, which is characteristic of prolonged asthma. TGFβ has also been found to create resistance to the effects of β2-agonist bronchodilators that are currently prescribed as asthma therapy37.
Fisetin may also exert its therapeutic effects through the gut–lung axis, as it has been shown that fisetin can regulate gut microbiota38, 39. Intact gut bacteria or their metabolites, especially short-chain fatty acids (SCFAs), may cross the intestinal barrier, reach the systemic circulation, and modulate immune response in the lungs40. SCFAs, whether from bacterial metabolites or high-fiber diets, may act in the lungs as signaling molecules to resident APCs to stimulate the immune response40. It has been reported that SCFA-deficient mice with induced asthma showed an elevated inflammatory response40.
Fisetin has also been found to have senolytic properties and may aid in the prevention of senescent tissue damage and the destruction of senescent cells, exacerbated by acute inflammation due to the repetitive onslaught of allergic asthma. Tissue aging is marked by enhanced cellular senescence, a stress-response that induces damaged cells to exit from the cell cycle and produce a pro-inflammatory, senescence-associated secretory phenotype (SASP). Recent research has reported further evidence that fisetin may act as a powerful senolytic41.
With the worldwide increase in asthma, the high cost of therapy, and the side effects of current therapeutic strategies, our study of fisetin in a pre-clinical mouse model may provide a starting point for the development of a safer, more efficient, and more cost-effective treatment for asthma.
Conclusions
Orally administered fisetin, in an aqueous solution and at a low dose of 50 nM (corresponding to 0.6 MPK), appears to be a safe and economical method for treating asthma in its early acute inflammatory phase, based on its effects in a preclinical mouse model. In the acute phase of the disease, fisetin acts by inhibiting eosinophilic infiltration, IgE production, CD3+CD4+ TH cell production and migration, activation of NFκB and TNFα, along with the TH2 cytokines.
Abbreviations
Ova: Ovalbumin; AHR: Airway hyper-responsiveness; MPK: Milligram (of therapeutic agent) per kg body weight of mouse; Penh: Enhanced pause; Mch: Methacholine; PB: Peripheral blood; BALF: Broncho-alveolar lavage fluid; IL: Interleukin; CD: Cluster of differentiation; IgE: Immunoglobulin E; iNOS: Inducible nitric oxide synthase; NFκB: Nuclear factor κ- light chain enhancer of activated B cells; STAT: Signal transducer and activator of transcription; IFNγ: Interferon γ; PBS: Phosphate buffered saline; PBST: Phosphate buffered saline with Tween-20; DMEM: Dulbecco’s Modified Eagle Medium; HRP: Horse radish peroxidase; IMDM: Iscove’s Modified Dulbecco’s Medium; FBS: Fetal bovine serum; BSA: Bovine serum albumin; PCR: Polymerase chain reaction; LABA: Long Acting Beta Agonist; SABA: Short Acting Beta Agonist.
Acknowledgments
We acknowledge the BD- CoE at the Centre for Research in Nanoscience & Nanotechnology (CRNN), University of Calcutta, Kolkata, for allowing us to use the BD FACSVerse, and the Indian Institute of Chemical Biology (IICB), Kolkata for allowing us to use the Azure Chemidoc system.
Author’s contributions
All authors have read and approved the manuscript. SM designed and performed the experiment and the assays, analyzed the data and wrote the manuscript. NG performed the experiment and some of the assays. PP assisted in the intratracheal experimental setup. ERB initiated the project, designed the experiments, analyzed the data and wrote the manuscript. All authors read and approved the final manuscript.
Funding
This work was supported by Prof. Ray Banerjee’s grants from the Indian Council of Medical Research (ICMR), UGC-UPE-II and UGC-SAP. We acknowledge the University Grants Commission (UGC), for providing fellowship to SM, and ICMR for providing fellowship for NG.
Availability of data and materials
Data and materials used and/or analyzed during the current study are available from the corresponding author on reasonable request.
Ethics approval and consent to participate
Not applicable.
Consent for publication
Not applicable.
Competing interests
The authors declare that they have no competing interests.
References
-
Ndarukwa
P.,
Chimbari
M.J.,
Sibanda
E.N.,
Protocol on a systematic review of qualitative studies on asthma treatment challenges experienced in Sub-Saharan Africa. Systematic Reviews.
2019;
8
(1)
:
149-57
.
View Article PubMed Google Scholar -
Bonsignore
M.R.,
Profita
M.,
Gagliardo
R.,
Riccobono
L.,
Chiappara
G.,
Pace
E.,
Advances in asthma pathophysiology: stepping forward from the Maurizio Vignola experience. European Respiratory Review.
2015;
24
(135)
:
30-9
.
View Article PubMed Google Scholar -
Kudo
M.,
Ishigatsubo
Y.,
Aoki
I.,
Pathology of asthma. Frontiers in Microbiology.
2013;
4
:
263
.
View Article PubMed Google Scholar -
Global Asthma Network. The Global Asthma Report. 2018.
.
-
Milgram
L.J.,
Asthma medications should be available for over-the-counter use: con. Ann Am Thorac Soc.
2014;
11
(6)
:
975-979
.
View Article Google Scholar -
Khan
N.,
Syed
D.N.,
Ahmad
N.,
Mukhtar
H.,
Fisetin: a dietary antioxidant for health promotion. Antioxidants & Redox Signaling.
2013;
19
(2)
:
151-62
.
View Article PubMed Google Scholar -
Shakeri
F.,
Boskabady
M.H.,
Anti-inflammatory, antioxidant, and immunomodulatory effects of curcumin in ovalbumin-sensitized rat. BioFactors (Oxford, England).
2017;
43
(4)
:
567-76
.
View Article PubMed Google Scholar -
Subhashini
Chauhan
P.S.,
Dash
D.,
Paul
B.N.,
Singh
R.,
Intranasal curcumin ameliorates airway inflammation and obstruction by regulating MAPKinase activation (p38, Erk and JNK) and prostaglandin D2 release in murine model of asthma. International Immunopharmacology.
2016;
31
:
200-6
.
View Article PubMed Google Scholar -
Banerjee
E.R.,
Jiang
Y.,
Henderson
W.R.,
Latchman
Y.,
Papayannopoulou
T.,
Absence of alpha 4 but not beta 2 integrins restrains development of chronic allergic asthma using mouse genetic models. Experimental Hematology.
2009;
37
(6)
.
View Article PubMed Google Scholar -
Mitra
S.,
Paul
P.,
Mukherjee
K.,
Biswas
S.,
Jain
M.,
Sinha
A.,
Mesoporous Nano-carbon particle Loaded Fisetin has a Positive Therapeutic Effect in a Murine Preclinical Model of Ovalbumin Induced Acute Allergic Asthma. Journal of Nanomedicine & Biotherapeutic Discovery.
2015;
5
:
132
.
-
Wu
M.Y.,
Hung
S.K.,
Fu
S.L.,
Immunosuppressive effects of fisetin in ovalbumin-induced asthma through inhibition of NF-κB activity. Journal of Agricultural and Food Chemistry.
2011;
59
(19)
:
10496-504
.
View Article PubMed Google Scholar -
Kim
H.Y.,
Goo
J.H.,
Joo
Y.A.,
Lee
H.Y.,
Lee
S.M.,
Oh
C.T.,
Impact on inflammation and recovery of skin barrier by nordihydroguaiaretic Acid as a protease-activated receptor 2 antagonist. Biomolecules & Therapeutics.
2012;
20
(5)
:
463-9
.
View Article PubMed Google Scholar -
Han
H.,
Ziegler
S.F.,
Bronchoalveolar Lavage and Lung Tissue Digestion. Bio-Protocol.
2013;
3
(16)
:
e859
.
View Article PubMed Google Scholar -
Sood
R.,
Medical Laboratory Technology: Methods and InterpretationsJaypee Brothers Medical Publishers Pvt Ltd: India; 2009.
Google Scholar -
Newnham
D.M.,
Asthma medications and their potential adverse effects in the elderly: recommendations for prescribing. Drug Safety.
2001;
24
(14)
:
1065-80
.
View Article PubMed Google Scholar -
Gupta
P.,
O'Mahony
M.S.,
Potential adverse effects of bronchodilators in the treatment of airways obstruction in older people: recommendations for prescribing. Drugs & Aging.
2008;
25
(5)
:
415-43
.
View Article PubMed Google Scholar -
Guo
P.,
Feng
Y.Y.,
Anti-inflammatory effects of kaempferol, myricetin, fisetin and ibuprofen in neonatal rats. Tropical Journal of Pharmaceutical Research.
2017;
16
(8)
:
1819-26
.
View Article Google Scholar -
Goh
F.Y.,
Upton
N.,
Guan
S.,
Cheng
C.,
Shanmugam
M.K.,
Sethi
G.,
Fisetin, a bioactive flavonol, attenuates allergic airway inflammation through negative regulation of NF-κB. European Journal of Pharmacology.
2012;
679
(1-3)
:
109-16
.
View Article PubMed Google Scholar -
Huang
W.,
Li
M.L.,
Xia
M.Y.,
Shao
J.Y.,
Fisetin-treatment alleviates airway inflammation through inhbition of MyD88/NF-κB signaling pathway. International Journal of Molecular Medicine.
2018;
42
(1)
:
208-18
.
View Article PubMed Google Scholar -
Wood
L.J.,
Sehmi
R.,
Dorman
S.,
Hamid
Q.,
Tulic
M.K.,
Watson
R.M.,
Allergen-induced increases in bone marrow T lymphocytes and interleukin-5 expression in subjects with asthma. American Journal of Respiratory and Critical Care Medicine.
2002;
166
(6)
:
883-9
.
View Article PubMed Google Scholar -
Robinson
D.S.,
The role of the T cell in asthma. The Journal of Allergy and Clinical Immunology.
2010;
126
(6)
:
1081-91
.
View Article PubMed Google Scholar -
Zhu
X.,
Cui
J.,
Yi
L.,
Qin
J.,
Tulake
W.,
Teng
F.,
The Role of T Cells and Macrophages in Asthma Pathogenesis: A New Perspective on Mutual Crosstalk. Mediators of Inflammation.
2020;
2020
:
7835284
.
View Article PubMed Google Scholar -
Berry
M.,
Brightling
C.,
Pavord
I.,
Wardlaw
A.,
TNF-alpha in asthma. Current Opinion in Pharmacology.
2007;
7
(3)
:
279-82
.
View Article PubMed Google Scholar -
Manni
M.L.,
Trudeau
J.B.,
Scheller
E.V.,
Mandalapu
S.,
Elloso
M.M.,
Kolls
J.K.,
The complex relationship between inflammation and lung function in severe asthma. Mucosal Immunology.
2014;
7
(5)
:
1186-98
.
View Article PubMed Google Scholar -
Miyake
T.,
Miyake
T.,
Sakaguchi
M.,
Nankai
H.,
Nakazawa
T.,
Morishita
R.,
Prevention of Asthma Exacerbation in a Mouse Model by Simultaneous Inhibition of NF-κB and STAT6 Activation Using a Chimeric Decoy Strategy. Molecular Therapy. Nucleic Acids.
2018;
10
:
159-69
.
View Article PubMed Google Scholar -
Rael
E.L.,
Lockey
R.F.,
Interleukin-13 signaling and its role in asthma. The World Allergy Organization Journal.
2011;
4
(3)
:
54-64
.
View Article PubMed Google Scholar -
Marone
G.,
Granata
F.,
Pucino
V.,
Pecoraro
A.,
Heffler
E.,
Loffredo
S.,
The Intriguing Role of Interleukin 13 in the Pathophysiology of Asthma. Frontiers in Pharmacology.
2019;
10
:
1387
.
View Article PubMed Google Scholar -
Lin
N.,
Simon
M.C.,
Hypoxia-inducible factors: key regulators of myeloid cells during inflammation. The Journal of Clinical Investigation.
2016;
126
(10)
:
3661-71
.
View Article PubMed Google Scholar -
Paul
P.,
Majhi
S.,
Mitra
S.,
Banerjee
E.R.,
Orally administered fisetin as an immunomodulatory and therapeutic agent in a mouse model of chronic allergic airway disease. Biomedical Research and Therapy.
2019;
6
(7)
:
3262-73
.
View Article Google Scholar -
Mitra
S.,
Mukherjee
K.,
Biswas
S.,
Banerjee
E.R.,
Prophylactic Use of Fisetin in Thioglycollate-Induced Peritonitis in Mice. Biological Systems, Open Access.
2015;
4
(2)
:
144
.
View Article Google Scholar -
Mitra
S.,
Biswas
S.,
Sinha
A.,
Jana
N.R.,
Banerjee
E.R.,
Therapeutic use of Fisetin and Fisetin Loaded on Mesoporous Carbon Nanoparticle (MCN) in Thioglycollate-induced Peritonitis. Journal of Nanomedicine & Nanotechnology.
2015;
6
(6)
:
332
.
View Article Google Scholar -
Kar
S.,
Konsam
S.,
Hore
G.,
Mitra
S.,
Biswas
S.,
Sinha
A.,
Therapeutic use of fisetin, curcumin, and mesoporous carbon nanoparticle loaded fisetin in bleomycin-induced idiopathic pulmonary fibrosis. Biomedical Research and Therapy.
2015;
2
(4)
:
250-62
.
-
Kar
S.,
Biswas
S.,
Banerjee
E.R.,
Evaluating the ameliorative potential of plant flavonoids and their nanocomposites in bleomycin induced idiopathic pulmonary fibrosis. Biomedical Research and Therapy.
2016;
3
(7)
:
707-22
.
View Article Google Scholar -
Makinde
T.,
Murphy
R.F.,
Agrawal
D.K.,
The regulatory role of TGF-beta in airway remodeling in asthma. Immunology and Cell Biology.
2007;
85
(5)
:
348-56
.
View Article PubMed Google Scholar -
Halwani
R.,
Al-Muhsen
S.,
Al-Jahdali
H.,
Hamid
Q.,
Role of transforming growth factor-β in airway remodeling in asthma. American Journal of Respiratory Cell and Molecular Biology.
2011;
44
(2)
:
127-33
.
View Article PubMed Google Scholar -
Al-Alawi
M.,
Hassan
T.,
Chotirmall
S.H.,
Transforming growth factor β and severe asthma: a perfect storm. Respiratory Medicine.
2014;
108
(10)
:
1409-23
.
View Article PubMed Google Scholar -
Wortley
M.A.,
Bonvini
S.J.,
Transforming Growth Factor-β1: A Novel Cause of Resistance to Bronchodilators in Asthma?. American Journal of Respiratory Cell and Molecular Biology.
2019;
61
(2)
:
134-5
.
View Article PubMed Google Scholar -
Chen
T.J.,
Feng
Y.,
Liu
T.,
Wu
T.T.,
Chen
Y.J.,
Li
X.,
Fisetin Regulates Gut Microbiota and Exerts Neuroprotective Effect on Mouse Model of Parkinson's Disease. Frontiers in Neuroscience.
2020;
14
:
549037
.
View Article PubMed Google Scholar -
Lin
J.,
Nie
X.,
Xiong
Y.,
Gong
Z.,
Chen
J.,
Chen
C.,
Fisetin regulates gut microbiota to decrease CCR9+/CXCR3+/CD4+ T-lymphocyte count and IL-12 secretion to alleviate premature ovarian failure in mice. American Journal of Translational Research.
2020;
12
(1)
:
203-47
.
PubMed Google Scholar -
Enaud
R.,
Prevel
R.,
Ciarlo
E.,
Beaufils
F.,
Wieërs
G.,
Guery
B.,
The Gut-Lung Axis in Health and Respiratory Diseases: A Place for Inter-Organ and Inter-Kingdom Crosstalks. Frontiers in Cellular and Infection Microbiology.
2020;
10
:
9
.
View Article PubMed Google Scholar -
Ellison-Hughes
G.M.,
First evidence that senolytics are effective at decreasing senescent cells in humans. EBioMedicine.
2020;
56
:
102473-4
.
View Article PubMed Google Scholar
Comments
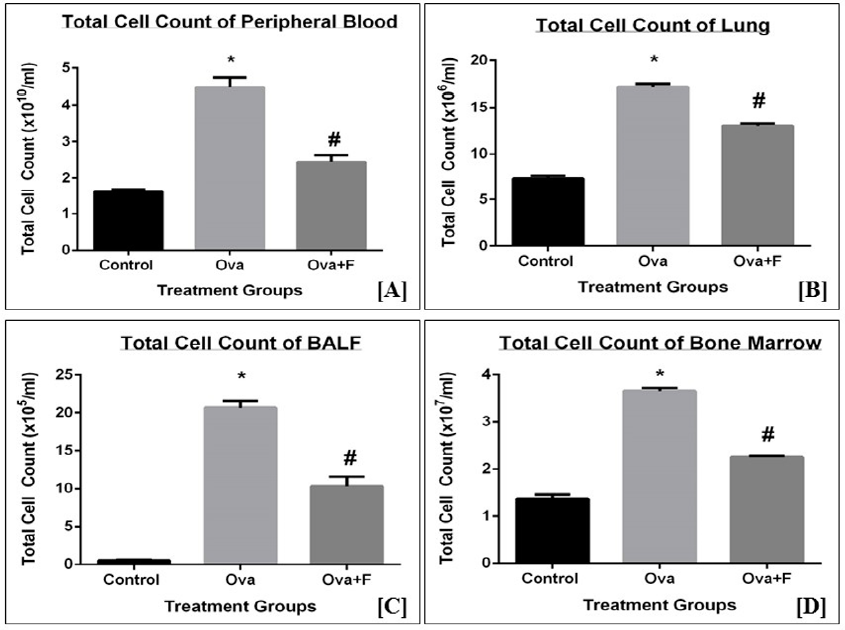
Article Details
Volume & Issue : Vol 9 No 3 (2022)
Page No.: 4953-4970
Published on: 2022-03-30
Citations
Copyrights & License

This work is licensed under a Creative Commons Attribution 4.0 International License.
Search Panel
Pubmed
Google Scholar
Pubmed
Google Scholar
Pubmed
Google Scholar
Pubmed
Search for this article in:
Google Scholar
Researchgate
- HTML viewed - 6576 times
- PDF downloaded - 1771 times
- XML downloaded - 0 times