Abstract
Severe burns result in deep and extensive wounds that are associated with a high mortality rate. While wound closure is an essential part of the treatment for such injuries, merely providing superficial coverage of the defect is inadequate. Adequate reconstruction requires repairing the damaged area from the innermost layers outward. Hydrogel dressings have become a very popular choice due to their unique properties: contributing to wound moisture, cooling and soothing, and autolytic debridement. This type of dressing offers the potential to overcome disadvantages of traditional treatments and allows partial skin regeneration. This review aims to outline the benefits of hydrogel dressings, emphasizing their role in wound healing and tissue regeneration, particularly in the context of chronic burn wounds. The discussion also covers how these dressings may address current shortcomings in wound care and provides a focused overview of specific attributes and potential future improvements in the field. This review enhances the general understanding of their therapeutic implications by examining the benefits of hydrogel dressings.
Introduction
It is estimated that between 7 and 12 million people (8,955,228 new burns in 20191) with burns require medical attention each year2. It is one of the leading causes of morbidity and mortality, especially in underdeveloped countries. Even when skin damage has healed, it is still profoundly transformed. This has a negative impact on the patient's physical, mental, and emotional well-being.
Burns occur due to exposure to heat sources, which can come from many different agents: fire, boiling water, electricity, chemicals, etc. The extent of the burn correlates with the severity of illness, causing long-term disability and death. Based on the severity of the burn, burns can be classified as follows: Burns that affect only the epidermis (pink or red, dry, without blisters, and moderately painful) are classified as group 1. They do not leave scars and heal relatively quickly, within 5 to 10 days. An example of this type 1 burn is a sunburn. Next, burns that spread into the dermis are classified as group 2; blisters and wet spots appear on the burn surface, and treatment lasts from 3 to 8 weeks, often leaving scars. This is the most common type of burn injury in daily life. Third-degree burns involve the full thickness of the skin and subcutaneous structures. These burns are complex, with dry, rough (central) and wet (marginal) areas. When left to heal naturally, the tissue often shrinks and changes structure, causing long-term injury. It takes more than eight weeks for this group to recover. Burns in the third degree and above typically require the implant method. Burns with charred (blackened) skin, where the entire skin structure is severely damaged and may expose bone, are classified as category 4. This is the most severe type of burn, with a high likelihood of death. The burn wound is shown in Figure 1.
Treating burns is a major challenge because systemic inflammatory response syndrome, sepsis, and multi-organ dysfunction syndrome—often caused by infection3—are still the leading causes of illness and death in burn patients. Burn injuries are complex and can easily progress from acute to chronic4. Complications of burns include scarring, contractures, and eschars, which have long-term negative impacts on a patient’s physical and mental health [4, 5]. These complications are the leading cause of death in most cases5.
Burn wounds disrupt the normal skin barrier and weaken many host defense mechanisms, the most important of which is preventing infection3. The main methods for managing burn infections often include wound care, isolation of the affected area from the environment, systemic antibiotic treatment, and autologous, allogeneic, or xenogeneic skin grafts to improve wound healing6. However, each of these methods has significant drawbacks: excessive and long-term antibiotic use can lead to bacterial resistance and changes in the human microbiome, while there is a limited supply of skin grafts and potential immune reactions to non-autologous grafts. New approaches to designing wound dressings have shown considerable promise by providing active processes for wound treatment. The unique three-dimensional network of hydrogels has a high water affinity compared to traditional wound dressings—which can cause skin irritation, dryness, and inadequate protection—thereby creating a moist healing environment with biological compatibility and biodegradability. Hydrogels protect the wound by offering ideal conditions for skin regeneration, thereby supporting effective treatment of infectious diseases. This is most important for contributing to smaller wound size, restricting the proliferation of infectious agents, and assisting tissue repair and healing by enhancing the body’s inherent healing capabilities7.
Hydrogels in Burn Wound Management
Most hydrogels are highly hydrophilic and have good biocompatibility. In addition, hydrogels with suitable pore structures can meet the needs of gas exchange during the healing process in general and particularly for burns8. They act as a temporary protective barrier, replacing damaged skin tissue in the wound. This is important because hydrogels can be designed to release bioactive agents in ways that enhance their therapeutic potential. The controlled-release mechanism adds another layer of functionality to hydrogel dressings and makes them versatile tools in the handling of wounds—particularly burns—where the healing process is highly elaborate and warrants specialized care8. Hydrogels have been widely used and have played an indispensable role in both research and clinical practice (Figure 2 ). Several studies have demonstrated the effectiveness of hydrogels applied in the treatment of burns based on the following effects they provide:
Cools burn wounds and closes the wound
The initial burn wound is not the only problem. The accumulated energy at the burn wound surface should also be a concern. This accumulated energy can be transmitted deeper or spread around the wound, causing tissue near the damage to be affected7. The easiest and most common method of eliminating this energy is running water. Although there is still concern about infection in the water—especially in areas where living standards are uncertain—it remains the recommended method because of its prevalence. Hydrogel dressings may be a more optimal alternative. They quickly dissipate heat accumulated in the skin. The more water-based they are, the more effectively they can absorb heat, quickly cool the wound, and reduce pain, making the patient feel more comfortable. In addition, they serve as a temporary protective barrier against external infectious agents. The water content of the hydrogel is essential in cooling, as water helps stabilize the wound’s temperature, especially in pediatric patients. The ability to hold large amounts of water makes them an effective heat-regulating shield for wounds in particular and the body in general. Along with that ability, the diffusion of biologically active substances in the hydrogel sheet and the delivery of drugs to the wound site are also highly appreciated7.
Fiona M. Wood’s research at the Burn Registry of Australia and New Zealand (BRANZ) analyzed data from 2009 to 2012 regarding water first aid after burns. In total, 68% of the patients performed first aid before hospital admission. Forty-six percent cooled for more than 20 minutes, associated with a 13% reduction in the probability of skin graft surgery (p = 0.014) and a 48% reduced probability of ICU admission (p < 0.001). The length of hospital stay also decreased by 18% (2.27 days), from 12.9 days (p = 0.001). These results underscore the significant benefits of timely first aid in burn treatment9. One study reported that 25 women who were to undergo breast reconstruction surgery consented to test the burn and cooling model without developing any side effects. Increased contact times produced deeper burns: contact for 7.5 seconds at a temperature of 70°C resulted in partial-thickness burn injuries. However, cooling at 16°C for 20 minutes conserved 25.2% of skin thickness. These findings, therefore, indicate that cooling as an immediate first-aid treatment for burns exerts a beneficial effect10. A pig model was used to study partial-thickness burns. The groups were treated with flowing water at 15°C for varying durations (10, 20, 30 minutes, and 1 hour) and delay times (immediate, 10 minutes, 1 hour, and 3 hours) compared with an untreated control group. Subdermal temperature was monitored, and the wounds were observed weekly for 6 weeks to assess re-epithelialization, surface area, and aesthetics. The results showed that immediate cooling with cold water for 20 minutes improved re-epithelialization and reduced scar tissue, while other time intervals also provided benefits11.
The faster the wound closes, the lower the risk of infection and the formation of hypertrophic scars. An example is an ultra-short peptide assembled to form a super-coiled hydrogel scaffold. These scaffolds are non-cytotoxic and do not induce an immune response. For partial-thickness burns, these nanofibrous hydrogels accelerated wound closure and dermal and epithelial regeneration with wound closure rates of 86.2% and 92.9% after 14 days, respectively, compared with 62.8% of burned areas healed using Mepitel®—a commercial dressing12. Another study in rats showed that gelatin hydrogels also promote effective wound healing of second-degree burns in a rat model13. A hydrogel with quercetin reduced burn size by 45–50% by day four in a mouse model of second-degree burns, which was similar to what was observed in the group treated with silver sulfadiazine. In the wounds that healed with the treatment, tensile strength estimates, biochemical markers, connective tissue signs, and NF-κB levels were restored by day 21 to levels similar to those of normal skin. Silicone gels were shown to promote rapid epithelialization in 16 burn wounds at skin surface depth (mean 8.4 days), compared with ointment-impregnated dressings (median 14 days, p < 0.01)14. Results showed less pain and exudate by mere observation of the absorbent materials from both types of dressings. In nine mixed deep and superficial dermal burns, the silicone gel also provided fast epithelization—an average of 12 days—compared with control wounds with an average of 22 days (p < 0.01).
Antibacterial
Much importance must be given to the antimicrobial performance of hydrogels when they are used as wound dressings. In wound treatment, hydrogel dressings have gained wide acceptance due to their ability to provide an antimicrobial or bacteriostatic effect. Their key role is to inhibit the spread of unwanted microorganisms and fight against infection in the wound. Hydrogel dressings offer improved coverage of the wound surface to prevent infection, including acting as an effective barrier against external pathogens. Functional modifications of hydrogels are performed to provide enhanced antimicrobial activity by adding antimicrobial agents, including silver nanoparticles or antibiotics15. This increases the hydrogel’s potential to inhibit bacterial growth at the lesion site. Antibacterial interactions can occur by mechanisms such as disruption of the bacterial cell wall/membranes, interference with bacterial replication, or inhibition of bacterial metabolic pathways. Hydrogels have a complex structure that plays a twofold role in protecting against bacterial invasion. The connections in the hydrogel’s structure act like a physical barrier, blocking bacteria from reaching the wound surface.
Conversely, this structure permits the evaporation of water and the penetration of oxygen into the wound, thus providing favorable conditions for wound healing. Importantly, most hydrogel sheets are not intrinsically antimicrobial, although their structure provides a suitable framework for incorporating antimicrobial agents that can be added to achieve the overall antibacterial activity of the hydrogel dressing16. Such flexibility in action makes hydrogel dressings adaptable and powerful tools in medicine, specifically in wound management, where preventing infection is one of the most essential aspects.
There are many ways to impart antibacterial ability to hydrogel bandages, such as using nanoparticles, using antibiotics, using hydrogels with inherent antibacterial properties, or synergistic approaches, etc. All are shown in Table 1 . Nanotechnology is particularly interesting due to its broad-spectrum antibacterial ability and its lack of drug resistance.
Hydrogel dressing | Antibiotic | Antibacterial Activity | Result |
Nanoparticle | |||
Polyvinyl alcohol and maleic acid 17 , Peptide 18 Nanocompozit 19 Polyethylene glycol (PEG) and polycaprolactone (PCL) 20 | Ag | Nano-silver interacts with bacterial membranes; thus, it causes membrane damage and results in cytoplasmic leakage. It ultimately inhibits growth and leads to the death of bacteria. Moreover, silver nanoparticles have a great binding ability and affinity with most of the macromolecules, having a great effect on the survival of bacterial species. | ++ |
Gelatin 21 , HCZ 22 | ZnO | Similar to silver nanoparticles, ZnO nanoparticles can sustainably bind to bacterial cell membranes and destroy cell membranes, including lipids and proteins. Membrane integrity occurs and this causes the bacteria to die. Besides, Zn 2+ can destroy bacterial cells as a strong oxidant, induce oxidative stress on cytoplasm and induce cytotoxicity. | + |
Poly(AAm-co-AMPS)-g-CMC 23 | Titanium Dioxide | Acts as a ROS-generating agent. It can lead to oxidative stress, causing damage to various cellular components and ultimately leading to cell death. | + |
Polysaccharide 24 | Other | Ditto | + |
Cellulose Nanocrystals 25 , Hydrogel 26 , N-(2-hydroxypropyl)-3-trimetylammonium chitosan clorua 27 | Antibiotic | Glycopeptides bind to the D-alanyl-D-alanine terminus of the peptidoglycan precursor, preventing its incorporation into the growing cell wall. These antibiotics are effective against Gram-positive bacteria; Aminoglycosides, including gentamicin and streptomycin, act by blocking protein synthesis. They bind to the bacterial 30S ribosomal subunit, causing a misreading of the genetic code during translation. This results in the incorporation of incorrect amino acids into the growing polypeptide chain. Quinolones, such as ciprofloxacin and levofloxacin, inhibit bacterial DNA synthesis. They target bacterial DNA gyrase and topoisomerase IV, essential DNA replication and repair enzymes. By inhibiting these enzymes, quinolones prevent the relaxation of supercoiled DNA, leading to DNA strand breakage and inhibition of nucleic acid synthesis. | +++ |
CL/nHA 28 , O-Mannosylation 29 | Glycopeptide | 26 | ++ |
Chitosan 30 , Hydroxypropyl methylcellulose and biodegradable microfibres 31 | Quinolone | 25 , 26 | + |
Chitosan Peptides Polymers | Natural | Chitosan interacts with microbial cell membranes. The positively charged amino groups in chitosan can bind to the negatively charged microbial cell membranes, leading to disruption. Peptides, Polymers: wound dressings and surface coatings | + |
Axit acrylic (AA), acrylamide (AAm), and 2-hydroxyethyl acrylate (HEA) 32 | Amphibian ionic antibacterial | The polymer film exerts a profound influence on bacterial metabolism, leading to the destabilization and ultimate disruption of the bacterial cell membrane. This mechanism showcases the specificity and effectiveness of the positively charged polymer in targeting and compromising bacterial integrity. | ++ |
Ethylene glycol 33 | Antimicrobial peptides | The amphiphilic nature of AMP is characterized by a hydrophobic core and a positively charged polycationic surface. This special structure enhances its ability to interact specifically with negatively charged membranes of bacteria, enabling it to show its antimicrobial action more effectively. AMPs act in a manner much similar to that of Glycopeptide. | ++ |
There are several approaches to making hydrogels containing nanoparticles with antibacterial activity. One approach is to expose the hydrogel to different solution conditions that cause it to expand and contract continuously, leading to periodic repackaging of the silver nanoparticles within the gel. The resistance to E. coli of the nanosilver-loaded gel increased based on the number of cycles the hydrogel had to undergo34. Another approach is to use NPs during gelation35. Alternatively, silver nitrate can be directly reduced in the gel network36. Another effective method is the incorporation of antibacterial polymers during hydrogel synthesis. Most of these antibacterial polymers have intrinsic antibacterial activity and hence, when blended into the hydrogel matrix, they enhance the hydrogel’s antibacterial properties. Several strategies are used for the development of antibacterial hydrogels, including the integration of antibacterial nanoparticles, surface modification with antibacterial functionalities, encapsulation of antibacterial agents, and incorporation of antibacterial polymers during hydrogel synthesis. Each of these methods has certain advantages, making the field of application versatile and effective.
Other than the use of nanoparticles, antibiotics have long been considered the number-one priority in fighting infections. Although antibiotic resistance is a major drawback, the effectiveness of antibiotics is indisputable. In a rabbit open fracture model, a hydrogel containing gentamicin was shown to be effective in preventing S. aureus infection37. White blood cell counts in rabbit plasma, 7–18 days after surgery, were 12.3 × 10⁶ WBC/ml in the untreated group, compared to 8.9 × 10⁶ (Collagen Gen) WBC/ml in the treated group. A study by Boo et al. also demonstrated that this hydrogel, in combination with gentamicin and vancomycin, was effective against methicillin-resistant S. aureus in a large animal (sheep) model38. Vancomycin combined with hydrogel is crucial for treating MRSA infections, with effective dosing confirmed by a target trough concentration of 15–20 μg/ml or a 24-hour AUC of 400 μg·hour/ml. Silk sericin (SS)/poly (vinyl alcohol) (PVA) hydrogel containing azithromycin, synthesized by a freezing/thawing process, has also demonstrated effective antibacterial activity. This product accelerates healing of infected burns while reducing systemic effects such as liver and spleen enlargement compared with the control group39. Agarose containing minocycline or gentamicin has similar effects; it reduces burn depth and bacterial counts as effectively as silver sulfadiazine cream commonly used in large animal (pig) models40.
AMPs are among the most important first-line defense molecules, effective against a wide variety of microorganisms, especially antibiotic-resistant bacteria. Amphiphilic in nature, AMPs generally have a hydrophobic core and a polycationic surface that interacts with negatively charged bacterial membranes. AMPs are short chains of cationic amino acids produced by many organisms, contributing significantly to the innate immune response and showing promise in addressing antibiotic-resistant bacteria. In the work of Ehsan et al., AMP combined with hydrogel showed excellent antibacterial activity against Porphyromonas gingivalis. Furthermore, it supported autogenous bone growth following healing of defects in rat calf bones41. Incorporating antibacterial peptides into hydrogels is seen as a future trend because they are safe and avoid unwanted side effects42. In addition, the hydrogel itself may possess natural antibacterial properties. For example, chitosan is an antibacterial cationic polymer whose amino groups in an acidic environment can accept hydrogen ions (H+), rendering these groups positively charged. This positive charge can facilitate binding to negatively charged molecules such as DNA and proteins—useful for controlling drug release from chitosan hydrogels—and may also help repel bacteria in wound dressings due to the negative charge on bacterial membranes43. In addition, there are peptides, polymers, etc.42. The administration of antimicrobials alone lacks the inherent capability to directly facilitate wound healing; their primary function is to prevent infection, which in turn allows cell proliferation, differentiation, and migration to proceed. However, this process can be hampered if immune cells are preoccupied with fighting bacteria.
Promote wound healing
Wound healing depends largely on the proliferation and migration of cells at the wound surface. That is only possible when an extracellular matrix (ECM) is present to help anchor the cells. Hydrogels can serve that role. They possess several properties desirable for tissue engineering, and many are structurally similar to the ECM. One investigation showed that cells from a wound infiltrated the hydrogel matrix up to a depth of 100 µm within the first 24 hours44. Dextran-based hydrogels encouraged significant healing of deep burn wounds by facilitating new blood vessel formation and skin regeneration. Rapid revascularization was observed in mouse burn wounds treated with dextran hydrogel over a 3-week period. By day 7, endothelial cells had settled within the hydrogel, enhancing blood flow; by day 21, treated wounds had developed mature epithelial structures, including hair follicles and sebaceous glands. Within 5 weeks, skin thickness and morphology were nearly indistinguishable from normal mouse skin45.
A hydrogel can also act as a carrier for growth factors, releasing them into regenerating burn or wound tissue. Consequently, most hydrogels have a notable effect on tissue repair and regeneration. This effect can be further boosted by growth factors or cytokines from recombinant proteins or even by mesenchymal stem cells. These induce angiogenesis, skin cell interactions, extracellular matrix remodeling, and other related functions, playing a critical role in all phases of wound healing (inflammation, proliferation, and cellular migration). Examples include fibroblast growth factor, epidermal growth factor, human growth hormone, platelet-derived growth factor, insulin-like growth factor, and transforming growth factor. One recent study reported the preparation of a new chitosan hydrogel containing EGF-loaded CNCs, which accelerated wound healing by promoting granulation tissue formation with significant collagen deposition46. EGF (epidermal growth factor) plays a pivotal role in wound healing, acting as a chemoattractant that guides macrophages and fibroblasts to the wound site, thereby contributing substantially to the inflammatory response—an essential phase of wound healing47. EGF also exerts anti-inflammatory effects by inhibiting the inflammatory response and down-regulating TGF-β1 expression. It is an important modulator of collagen synthesis, thereby influencing healed tissue quality and minimizing scar formation. Its proliferative impact extends to endothelial and epithelial cells, stimulating their division and assisting their migration to the wound surface, actively contributing to wound contraction46, 48. Similar outcomes are reported with the addition of FGF39, PDGF49, PDGF50, 51, etc. The mechanism of action of a hydrogel, meanwhile, involves retaining growth factors and cytokines at the wound site for an extended period, due to its slow-release capability. This retention supports longer-term cell growth and differentiation, thereby augmenting wound healing and creating highly favorable conditions for native tissue regeneration and recovery. Such benefits help increase performance and durability in hydrogel-based medical applications.
Advances in the Application of Hydrogel Dressings in the Treatment of Burns
In just 10 years, the number of hydrogel studies related to burns has increased tenfold (Figure 2). This demonstrates the potential of hydrogels and their application to burn therapy as an area of increasing interest.
Technical and Preclinical Trials
The use of autologous skin in patients with severe burns for transplantation is limited because of the insufficient amount of healing skin. Moreover, culturing autologous skin takes several weeks before it can be used for transplantation. Such waiting times lead to higher infection rates and increased patient mortality. There are many approaches to wound healing, and one of them is direct aerosol spraying of a cell suspension onto the wound. A comparative study of direct aerosol delivery of a horn cell suspension with an in vitro fibrin delivery system showed that the aerosol approach was 20% more effective than fibrin. After seven days, aerosol-delivered cells expressed cytokeratin K6, indicating that the cells had begun to proliferate52. The same approach, when using a mixture of cells cultured on microporous biodegradable carriers distributed over the wound, also has a positive effect53. This method can be used on a large burn area without causing an immune response because the cells are autologous, and the hydrogel is inert. Patients also do not need anesthesia, and the procedure can be performed directly.
If the burn area is small, skin cells can be applied directly, and the process is quick—taking less than one hour—to produce a spray product, with cell survival rates of more than 75%54. This method can be performed in local hospitals and does not require highly advanced technical resources compared to other methods. The cells are evenly distributed over the wound, and their coverage is 100 times greater than the area from which they were harvested54, 55. If the burn area is large, the cells are cultured in the laboratory to increase their number, then mixed with hydrogel and applied. This process takes about 7–14 days. Another, more complex method is 3D skin generation, in which collagen is commonly used to create scaffolds for cell types. The proportions of substrates, fillers, and cells are accurately distributed by specialized software and machines, and the mechanical properties of this type of product can closely mimic human skin56. However, this approach has not become widespread because the machinery is expensive and requires a highly skilled workforce. In addition, such therapy is currently very costly.
Several preclinical and clinical studies have focused on the multidimensional roles of MSCs in enhancing the wound-healing process, suppressing inflammation, and preventing burn-related injuries. MSCs have also been shown to reduce scar development when burn wounds heal. It therefore follows that MSCs are important for overall improvements in wound healing and could offer potential therapeutic benefits in the management of burn-related complications57, 58. Consequently, using MSCs in combination with hydrogels is still gaining interest. Wei Zeng demonstrated that combining amniotic membranes with human amniotic membrane–derived mesenchymal stem cells improved corneal epithelium faster and with significantly less opacity than the control group in cases of corneal alkaline burns59. The combination of the amniotic membrane with bone marrow mesenchymal stem cells and keratinocytes has also shown a positive effect in a radiation burn model in piglets60. Because stem cells possess reparative and regenerative properties, they have gained much attention in skin care and restoration, especially in treating burns. However, direct stem cell application to injured skin in burn injuries still encounters major challenges due to the limited survival and maintained functionality of the cells in the compromised tissue environment. Important obstacles include ensuring stem cell survival and preserving their repair capacity under harsh conditions (e.g., high temperatures in burn injuries and sunlight exposure).
Advances in technology combined with hydrogel-based techniques have made cell survival in open wounds much more feasible. Nowadays, bioprinting combined with UV irradiation cures the material after printing, enabling the combination of stem cells, growth factors, and media in a spray form61. Using UV irradiation at 700 mW/cm² did not significantly affect cell viability compared to the control group without UV exposure (no statistically significant difference)61. This approach represents a promising future trend for burn treatment and potentially for other solid organs, particularly as therapy costs decrease and adoption becomes more widespread.
Clinical Trials
Clinical trials on treating burns with hydrogels were introduced in 1991 in Russia and Poland62, 63. These have been documented in clinical trials (Table 3). Hydrogel products are easier to apply practically than many drugs because of their biocompatibility and inertness. However, this also means that patented research is proprietary and not publicly accessible.
No | Hydrogel | Country | Class | Result | Note |
1 | Regencur 62 | Russia (1991) | II-IIIA | For burn areas from 1.5 to 20% of the body surface, using hydrogel has contributed to reducing pain, reducing dressing, and facilitating the healing process. | + |
2 | Hydrogel-type dressing materials (analysis) 63 | Polish (1991) | Diverse | The hygroscopic properties of hydrogel dressings and their covering power allow rapid wound protection from microorganisms. They can absorb antibiotics and drugs, contributing to the acceleration of the healing process and the growth of the epidermis. | + |
3 | Polyvinyl pyrrolidone-iodine 64 | Germany (2001) | Diverse | Early epithelialization and better wound healing with hydrogels. Besides, hydrogel maintains moisture and antiseptic superior to wounds treated with conventional antiseptic chlorhexidine gauze. | + |
4 | Hydrosome polyvinyl-pyrrolidone (PVP)-iodine 65 | Germany (2006) | Diverse | Hydrogel significantly enhanced epithelialization, reduced implant loss, and significantly improved healing (n=167, phase 3) | + |
5 | Liposome hydrogel with polyvinyl-pyrrolidone iodine 66 | Germany (2007) | Diverse | Hydrogel makes wounds heal faster with favorable cosmetic results.(n=43) | + |
6 | Hydrosome 67 | Germany (2007) | IIa | Hydrosome wound gel accelerated wound healing by 1.5-2 days compared to sulfadiazine cream. Faster epithelialization was observed with hydrosome wound gel treatment in 2/3 of the patients. hydrosome is safe against infection, well tolerated, and easy to use. (n=47) | + |
7 | rhGM-CSF hydrogel 68 | China (2008) | Diverse | Recombinant human granulocyte-macrophage colony-stimulating factor remarkably quickens wound healing. This novel therapy, in a study with 302 participants, is very promising. Although effective, there are certain safety issues related to the use of hydrogel loaded with rhGM-CSF. For sharp acceleration, patient safety again becomes a matter of concern and thus again is a point that will require further investigation. (n=302) | + |
8 | rhGM-CSF hydrogel 69 | China (2009) | IIa | The research revealed a statistically significant difference (p<0.01), indicating that the rhGM-CSF hydrogel significantly accelerated the healing of deep second-degree burn wounds. Importantly, no side effects were observed during the trial. (n=90) | + |
9 | SilvaSorb gel 70 | USA (2009) | II | SilvaSorb Gel is associated with less pain and more satisfied patients. | + |
10 | Procutase 71 | Italy (2010) |
This study showed that patients treated with Procutase had statistically significantly less pain and a shorter wound healing time. As a result, they can be used safely and effectively in patients with minor burns that do not require hospitalization.
|
+
|
|
11 | rhGM-CSF hydrogel 72 | China (2012) | II | rhGM-CSF hydrogel effectively promotes the healing of deep local thickness burns. In addition, no adverse drug reactions were observed during the study. | + |
12 | Aloe Vera gel 73 | Pakistan (2013) | Diverse | Burn wounds healed significantly faster than those treated with 1% silver sulfadiazine. All patients in the Aloe Vera group experienced earlier pain relief (n=50). | + |
13 | FLAMIGEL 74 | China (2013) | Diverse | FLAMIGEL can help to speed up the healing of burn wounds that have not yet fully healed and can also help to reduce the pain felt during dressing changes.(n=60) | + |
14 | CEAllo 75 | Korea (2014) | II | In the test region, the re-epithelialization was faster. No significant adverse reactions in the trial (n=15) | + |
15 | rhGM-CSF 76 | China (2015) | >II | The study's findings highlight the efficacy of rhGM-CSF hydrogel in facilitating the healing of residual wounds associated with extensive deep partial-thickness burns. Notably, the hydrogel exhibited antimicrobial properties, contributing to its overall therapeutic effectiveness. Furthermore, the study observed only mild local and general side reactions associated with the use of the hydrogel, indicating a favorable safety profile.(n=21) | + |
16 | Silicone hydrogel 77 | Australian (2015) | Diverse | The first and largest of its kind, provides mid-term insights into the outcomes of autologous limbal/conjunctival stem cell transplantation using an FDA-approved contact lens. The approach proved to be a viable, effective, and xeno-free alternative to existing transplantation methods, showcasing its potential as a promising strategy in the field.(n=16) | + |
17 | rhGM-CSF 78 | China (2016) | Diverse | External use of rhGM-CSF gel on deep partial-thickness burn wounds was found to promote wound healing and was deemed safe for clinical use based on the controlled and open trials. (n=68). | + |
18 | Hydrogel collagen with keratinocytes and fibroblasts 79 | Switzerland (2019) | Diverse | Achieve safe coverage for skin imperfections. The replacement skin heals steadily, and the skin quality is near normal. Histology at three months showed a stratified epidermis and a dermal cavity comparable to natural skin. The mean follow-up time was 15 months. | ++ |
19 | Hydrogel treprostinil 80 | France (2020) | Diverse | Hydrogel significantly increased blood flow through the skin in the legs (n=5) | + |
20 | Burnaid hydrogel dressing 81 | Australia (2021) | Diverse | The current study was designed to evaluate Burnaid hydrogel dressing as an analgesic adjunct to first aid in the management of acute pediatric burns. Seventy-two children were recruited and assigned randomly to either the intervention (n=37) or control group (n=35), with tabulation indicating no significant differences between the two groups in nursing or caregiver observational pain scores. No significant differences in means were found for child self-report of pain, heart rate, temperature, stress, and re-epithelialization rates. * This study did not determine any significant benefit to using Burnaid hydrogel dressing as an adjunctive analgesic to first aid in the treatment of acute burns in children. (n=72) | + |
21 | Hydrogel dressing + vaseline gauze 82 | China (2021) | >II | Hydrogel makes wounds heal faster, less painful, more comfortable. (n=168) | + |
22 | Burnaid hydrogel burn dressing (BHD) containing tea tree oil and silver sulfadiazine in pediatric burn patients 83 | Turkey (2024) | Prospective, observational, and cross-sectional study | Out of 64 participants with second-degree and mixed second-third degree burns, 62.5% received BHD, and 37.5% received silver sulfadiazine. BHD demonstrated significantly lower post-dressing pain scores compared to silver sulfadiazine, and fewer patients in the BHD group required additional analgesia. The study suggests that BHD may offer superior pain relief and reduced need for supplementary analgesics in pediatric burn patients, emphasizing its potential clinical benefits. | + |
The mentioned studies positively impact the wound healing process and show statistically significant differences compared to the control group. However, this is just the tip of the iceberg. Most studies on hydrogel dressings are commercialized, so their information is kept confidential within commercial companies.
Discussion
Current situation and challenges
With the world's increasing demands, numerous new hydrogel technologies are emerging. One of the interesting novelties in advanced hydrogel applications for wound healing is smart hydrogels. These can adhere to the wound and, upon stimulation by various stimuli (such as temperature, humidity, or chemical agents), they will contract. This property gives them exceptional suitability for different types of wounds, including variations in shape and thickness. They are designed based on materials with unique properties and specialized technical methods.
It can be envisioned that a smart hydrogel design would be very flexible, depending on changes in temperature, pH, or the presence of infection in the wound environment. Due to this responsiveness, they can adapt their cohesion mechanism according to the conditions and optimize it. H. Wang's research84 produced an innovative hydrogel, comprising pH-sensitive bromothymol blue and a near-infrared (NIR)-absorbing conjugated polymer integrated into thermosensitive chitosan, which allows for real-time, visual diagnosis and photothermal therapy of bacterial infections. It enables in situ detection of Staphylococcus aureus biofilm and infected wounds through visible color changes displayed by the hydrogel. Further, localized hyperthermia is sequentially triggered with NIR laser irradiation to ensure effective therapy. The hybrid hydrogel presents wide-range antibacterial efficacy with low cytotoxicity, making it a promising platform for the intelligent and convenient diagnosis and treatment of bacterial infection. Moreover, the pH-sensitive nature of the hydrogel enables control over the amount of drug released, as the acidity fluctuates at different stages of wound healing. Asmaa Ahmed Arafa et al.85 used pH-sensitive natural dye Curcuma longa extract (CLE) as a color indicator. Hydroxyethyl cellulose loaded with CLE and combined with itaconic acid produced a neutral and pH-sensitive dressing. This is highly useful, as the drug release from the dressing increases significantly at pH 7.4, suitable for treating many types of wounds.
In the case of increased temperatures—a clear indicator of inflammation—the hydrogel could self-regulate and deliver anti-inflammatory agents that would dampen inflammatory responses, facilitating an environment more conducive to tissue regeneration. Min et al.86 focus on a thermo-sensitive methylcellulose (MC) hydrogel containing silver oxide nanoparticles (Ag₂O). The drug release from this hydrogel is temperature-dependent. The silver oxide nanoparticle-loaded MC hydrogel exhibited very good antimicrobial activity and proved useful for treating burn wounds. For wounds susceptible to bacterial infection, such smart hydrogel bandages contain responsive elements capable of initiating the release of an antibacterial agent upon detecting the presence of bacteria. This provides not only a quick physical barrier to infection but also facilitates the general healing process by creating an environment less favorable to bacterial multiplication.
Hydrogel dressings play an important and promising role as biomaterials that act as protective barriers to replace damaged skin tissue, especially from burns. Hydrogel capabilities can also be enhanced by incorporating growth factors or stem cells they can carry. This flexibility is a great advantage of hydrogels, enabling them to be part of most lesion treatments caused by diseases, external factors, or tissue replacement. However, there are significant limitations when incorporating tissue engineering into burn treatment. Hydrogel dressings currently face several challenges, including high costs, low durability, and the risk of infection and allergies. Besides, although hydrogel dressings have proved their effectiveness, their universal efficacy in treating all categories of wounds has not been fully established. Moreover, despite their huge potential, hydrogel dressings are marketed heterogeneously and often require rigorous storage measures, resulting in high medical waste. This is further compounded by the fact that most hospitals and clinics are not equipped to manufacture hydrogel dressings at a clinical level. Therefore, distribution networks should be expanded, and hydrogel development must be done in an eco-friendly way to reduce waste. On the other hand, many studies are needed to address drawbacks such as drug compatibility issues, biofilm formation, and environmental effects—important safety and effectiveness concerns regarding hydrogels used in healthcare (Table 3 ).
Feature | Hydrogel dressing | Traditional gauze | Silicone dressing | Alginate dressing | Hydrocolloid dressing |
Moist environment | ++ | - | + | - | ++ |
Cools the wound | Yes | No | No | No | No |
Reduces scar formation | ++ | - | + | - | + |
Easy to use | ++ | + | - | + | + |
Safe | + | + | + | + | + |
Cost | - | ++ | - | + | + |
Easy to peel off | ++ | ++ | + | + | + |
Infection | + | + | ++ | ++ | ++ |
Allergy | + | ++ | ++ | ++ | ++ |
The major problem facing hydrogel dressings, which needs to be surmounted, is cost and availability through the healthcare system. First, hydrogel dressings are more expensive compared to other more conventional modes of treatment. This adds a financial burden on health facilities and influences patients’ choices. Another aspect is that hydrogel dressings must be applied effectively by healthcare service providers through training at hospitals and clinics. Third, evaluation related to hydrogel dressings needs to be considered from the patient’s perspective. Promotion of hydrogel dressings should be widened in terms of marketing campaigns. Focusing on the patient’s experience, their quality of life, and pain management outcomes in light of their satisfaction will assist in an appropriate study related to hydrogel dressings. All modes of treatment need to have the patient as the prime focus.
Future Research Directions
The Global Hydrogel Dressing Market is anticipated to achieve a valuation of $1.1 billion by 2028, with a projected Compound Annual Growth Rate (CAGR) of 4.2% during the forecast period87. This information demonstrates the increasing prevalence of hydrogel, concurrently showing its positive impact in treating various types of wounds. Overcoming current drawbacks—such as high costs, stability issues, and limited patient accessibility—will contribute to the growing popularity of hydrogel dressings.
Although hydrogel remains the top choice for maintaining moisture and causing minimal pain during dressing changes, it can be uncomfortable for patients. The development of hydrogel bandage designs that control drug release and undergo biodegradation (eliminating the need for frequent bandage changes) continues to be a trend in wound treatment in general, and burn treatment in particular. Personalized hydrogel therapies, in the form of sheets or injections containing the patient’s own cells, are also crucial. This involves developing hydrogel forms in which the growth and development of these cells are ensured under any given environmental conditions. Above all, the rise of smart hydrogel dressings is an inevitable trend. The following are emerging trends for hydrogels: (1) identifying bioactive, chemical, and metal particles that have antibacterial, anti-inflammatory, hemostatic functions, and accelerate wound healing; (2) developing or combining flexible materials to provide physical properties for hydrogels (such as tensile strength and pore size) for not only burn wounds but also all kinds of wounds; and (3) creating readily available hydrogel dressings for every household’s medicine cabinet, replacing traditional gauze dressings through competitive pricing.
As research in this field continues, hydrogels are likely to find even more applications in the future. New hydrogel technologies must keep demonstrating efficacy and safety through multiple clinical trials. The future of this industry is promising as these technologies mature and create products that resemble, or even replace, human skin. So far, we have witnessed only the beginning of this era of innovation and creativity. Researchers and physicians should continue fostering close collaboration between the laboratory and the clinic. This will speed up technology development and make it easier for new products to be implemented. The creation of artificial skin in particular, and artificial organs in general, depending on hydrogels, will soon become a reality.
Abbreviations
AMPs: Antimicrobial peptides; ECM: Extracellular matrix; EGF: Epidermal growth factor; rhGM-CSF: Recombinant human granulocyte-macrophage colony-stimulating factor; TGF-β: Transforming growth factor-beta
Acknowledgments
None.
Author’s contributions
Not applicable.
Funding
This article is funded by University of Science, VNU-HCM under grant number T2023-79.
Availability of data and materials
Not applicable.
Ethics approval and consent to participate
Not applicable.
Consent for publication
Not applicable.
Competing interests
The authors declare that they have no competing interests.
References
-
Yakupu
A.,
Zhang
J.,
Dong
W.,
Song
F.,
Dong
J.,
Lu
S.,
The epidemiological characteristic and trends of burns globally. BMC Public Health.
2022;
22
(1)
:
1596
.
PubMed Google Scholar -
James
S.L.,
Lucchesi
L.R.,
Bisignano
C.,
Castle
C.D.,
Dingels
Z.V.,
Fox
J.T.,
Epidemiology of injuries from fire, heat and hot substances: global, regional and national morbidity and mortality estimates from the Global Burden of Disease 2017 study. Injury prevention : journal of the International Society for Child and Adolescent Injury Prevention.
2020;
26
(Supp 1)
:
i36-i45
.
-
Church
D.,
Elsayed
S.,
Reid
O.,
Winston
B.,
Lindsay
R.,
Burn wound infections. Clinical Microbiology Reviews.
2006;
19
(2)
:
403-34
.
PubMed Google Scholar -
Barrett
L.W.,
Fear
V.S.,
Waithman
J.C.,
Wood
F.M.,
Fear
M.W.,
Understanding acute burn injury as a chronic disease. Burns and Trauma.
2019;
7
:
23
.
PubMed Google Scholar -
Lee
K.C.,
Joory
K.,
Moiemen
N.S.,
History of burns: the past, present and the future. Burns and Trauma.
2014;
2
(4)
:
169-80
.
PubMed Google Scholar -
Firlar
I.,
Altunbek
M.,
McCarthy
C.,
Ramalingam
M.,
Functional hydrogels for treatment of chronic wounds. Gels.
2022;
8
(2)
:
127
.
View Article Google Scholar -
A. Surowiecka,
J. Strużyna,
A. Winiarska,
T. Korzeniowski,
Hydrogels in burn wound management—A review. Gels.
2022;
8
(2)
:
122
.
View Article Google Scholar -
Goh
M.,
Du
M.,
Peng
W.R.,
Saw
P.E.,
Chen
Z.,
Advancing burn wound treatment: exploring hydrogel as a transdermal drug delivery system. Drug Delivery.
2024;
31
(1)
:
2300945
.
View Article PubMed Google Scholar -
Wood
F.M.,
Phillips
M.,
Jovic
T.,
Cassidy
J.T.,
Cameron
P.,
Edgar
D.W.,
Water First Aid Is Beneficial In Humans Post-Burn: Evidence from a Bi-National Cohort Study. PLoS One.
2016;
11
(1)
:
e0147259
.
View Article PubMed Google Scholar -
Wright
E.H.,
Tyler
M.,
Vojnovic
B.,
Pleat
J.,
Harris
A.,
Furniss
D.,
Human model of burn injury that quantifies the benefit of cooling as a first aid measure. British Journal of Surgery.
2019;
106
(11)
:
1472-9
.
PubMed Google Scholar -
Cuttle
L.,
Kempf
M.,
Liu
P.Y.,
Kravchuk
O.,
Kimble
R.M.,
The optimal duration and delay of first aid treatment for deep partial thickness burn injuries. Burns : journal of the International Society for Burn Injuries.
2010;
36
(5)
:
673-9
.
View Article Google Scholar -
Loo
Y.,
Wong
Y.C.,
Cai
E.Z.,
Ang
C.H.,
Raju
A.,
Lakshmanan
A.,
Ultrashort peptide nanofibrous hydrogels for the acceleration of healing of burn wounds. Biomaterials.
2014;
35
(17)
:
4805-14
.
PubMed Google Scholar -
George
B.,
Bhatia
N.,
Kumar
A.,
A
G.,
R
T.,
S K
S.,
Bioinspired gelatin based sticky hydrogel for diverse surfaces in burn wound care. Scientific Reports.
2022;
12
(1)
:
13735
.
PubMed Google Scholar -
Sawada
Y.,
Ara
M.,
Yotsuyanagi
T.,
Sone
K.,
Treatment of dermal depth burn wounds with an antimicrobial agent-releasing silicone gel sheet. Burns.
1990;
16
(5)
:
347-52
.
PubMed Google Scholar -
Jackson
J.,
Burt
H.,
Lange
D.,
Whang
I.,
Evans
R.,
Plackett
D.,
The design, characterization and antibacterial activity of heat and silver crosslinked poly (vinyl alcohol) hydrogel forming dressings containing silver nanoparticles. Nanomaterials.
2021;
11
(1)
:
96
.
View Article Google Scholar -
Gounden
V.,
Singh
M.,
Hydrogels and wound healing: Current and future prospects. Gels.
2024;
10
(1)
:
43
.
View Article Google Scholar -
Kazeminava
F.,
Arsalani
N.,
Ahmadi
R.,
Kafil
H.S.,
Geckeler
K.E.,
A facile approach to incorporate silver nanoparticles into solvent-free synthesized PEG-based hydrogels for antibacterial and catalytical applications. Polymer Testing.
2021;
101
:
106909
.
View Article Google Scholar -
Soto-Quintero
A.,
Romo-Uribe
Á.,
Bermúdez-Morales
V.H.,
Quijada-Garrido
I.,
Guarrotxena
N.,
3D-Hydrogel Based Polymeric Nanoreactors for Silver Nano-Antimicrobial Composites Generation. Nanomaterials (Basel, Switzerland).
2017;
7
(8)
:
209
.
PubMed Google Scholar -
Tao
B.,
Lin
C.,
Qin
X.,
Yu
Y.,
Guo
A.,
Li
K.,
Fabrication of gelatin-based and Zn2+-incorporated composite hydrogel for accelerated infected wound healing. Materials Today Bio.
2022;
13
:
100216
.
View Article PubMed Google Scholar -
Hwang
C.,
Choi
M.H.,
Kim
H.E.,
Jeong
S.H.,
Park
J.U.,
Reactive oxygen species-generating hydrogel platform for enhanced antibacterial therapy. NPG Asia Materials.
2022;
14
(1)
:
72
.
-
Martínez-Cornejo
V.,
Velázquez-Roblero
J.,
Rosiles-González
V.,
Correa-Duran
M.,
Avila-Ortega
A.,
Synthesis of poly (2-acrylamido-2-methylpropane sulfonic acid) and its block copolymers with methyl methacrylate and 2-hydroxyethyl methacrylate by quasiliving radical polymerization catalyzed by a cyclometalated ruthenium (II) complex. Polymers.
2020;
12
(8)
:
1663
.
View Article Google Scholar -
Kianpour
S.,
Ebrahiminezhad
A.,
Mohkam
M.,
Tamaddon
A.M.,
Dehshahri
A.,
Heidari
R.,
Physicochemical and biological characteristics of the nanostructured polysaccharide-iron hydrogel produced by microorganism Klebsiella oxytoca. Journal of Basic Microbiology.
2017;
57
(2)
:
132-40
.
PubMed Google Scholar -
Li
L.,
Cheng
X.,
Huang
Q.,
Cheng
Y.,
Advanced Healthcare Materials.
Sprayable Antibacterial Hydrogels by Simply Mixing of Aminoglycoside Antibiotics and Cellulose Nanocrystals for the Treatment of Infected Wounds. 2022;
11
(20)
:
2270123
.
View Article Google Scholar -
Boot
W.,
Foster
A.L.,
Guillaume
O.,
Eglin
D.,
Schmid
T.,
D'Este
M.,
An Antibiotic-Loaded Hydrogel Demonstrates Efficacy as Prophylaxis and Treatment in a Large Animal Model of Orthopaedic Device-Related Infection. Frontiers in Cellular and Infection Microbiology.
2022;
12
:
826392
.
View Article PubMed Google Scholar -
Hoque
J.,
Bhattacharjee
B.,
Prakash
R.G.,
Paramanandham
K.,
Haldar
J.,
Dual function injectable hydrogel for controlled release of antibiotic and local antibacterial therapy. Biomacromolecules.
2018;
19
(2)
:
267-78
.
View Article Google Scholar -
Wang
Y.,
Wang
J.,
Gao
R.,
Liu
X.,
Feng
Z.,
Zhang
C.,
Biomimetic glycopeptide hydrogel coated PCL/nHA scaffold for enhanced cranial bone regeneration via macrophage M2 polarization-induced osteo-immunomodulation. Biomaterials.
2022;
285
:
121538
.
View Article PubMed Google Scholar -
Chen
J.,
Luo
J.,
Tan
Y.,
Wang
M.,
Liu
Z.,
Yang
T.,
Effects of low-dose ALA-PDT on fibroblast photoaging induced by UVA irradiation and the underlying mechanisms. Photodiagnosis and Photodynamic Therapy.
2019;
27
:
79-84
.
View Article PubMed Google Scholar -
Wang
N.,
Xiao
W.,
Niu
B.,
Duan
W.,
Zhou
L.,
Zheng
Y.,
Highly efficient adsorption of fluoroquinolone antibiotics using chitosan derived granular hydrogel with 3D structure. Journal of Molecular Liquids.
2019;
281
:
307-14
.
View Article Google Scholar -
Agubata
C.O.,
Okereke
C.,
Nzekwe
I.T.,
Onoja
R.I.,
Obitte
N.C.,
Development and evaluation of wound healing hydrogels based on a quinolone, hydroxypropyl methylcellulose and biodegradable microfibres. European Journal of Pharmaceutical Sciences.
2016;
89
:
1-10
.
View Article PubMed Google Scholar -
Seo
H.S.,
Bae
J.Y.,
Kwon
K.,
Shin
S.,
Synthesis and assessment of amps-based copolymers prepared via electron-beam irradiation for ionic conductive hydrogels. Polymers.
2022;
14
(13)
:
2547
.
View Article Google Scholar -
Moorcroft
S.C.,
Roach
L.,
Jayne
D.G.,
Ong
Z.Y.,
Nanoparticle-loaded hydrogel for the light-activated release and photothermal enhancement of antimicrobial peptides. ACS applied materials & interfaces.
2020;
12
(22)
:
24544-54
.
View Article Google Scholar -
Nuñez
Y.A. Rodríguez,
Castro
R.I.,
Arenas
F.A.,
Preparation of hydrogel/silver nanohybrids mediated by tunable-size silver nanoparticles for potential antibacterial applications. Polymers.
2019;
11
(4)
:
716
.
View Article Google Scholar -
Binaymotlagh
R.,
Giudice
A. Del,
Green in situ synthesis of silver nanoparticles-peptide Hydrogel composites: Investigation of their antibacterial activities. Gels.
2022;
8
(11)
:
700
.
View Article Google Scholar -
Thomas
V.,
Yallapu
M.M.,
Sreedhar
B.,
Bajpai
S.,
Breathing-in/breathing-out approach to preparing nanosilver-loaded hydrogels: highly efficient antibacterial nanocomposites. Journal of Applied Polymer Science.
2009;
111
(2)
:
934-44
.
View Article Google Scholar -
Travan
A.,
Pelillo
C.,
Donati
I.,
Marsich
E.,
Benincasa
M.,
Scarpa
T.,
Non-cytotoxic silver nanoparticle-polysaccharide nanocomposites with antimicrobial activity. Biomacromolecules.
2009;
10
(6)
:
1429-35
.
View Article PubMed Google Scholar -
Thomas
V.,
Yallapu
M.M.,
Sreedhar
B.,
Bajpai
S.K.,
A versatile strategy to fabricate hydrogel-silver nanocomposites and investigation of their antimicrobial activity. Journal of Colloid and Interface Science.
2007;
315
(1)
:
389-95
.
View Article PubMed Google Scholar -
Ter Boo
G.A.,
Arens
D.,
Metsemakers
W.J.,
Zeiter
S.,
Richards
R.G.,
Grijpma
D.W.,
Injectable gentamicin-loaded thermo-responsive hyaluronic acid derivative prevents infection in a rabbit model. Acta Biomaterialia.
2016;
43
:
185-94
.
View Article PubMed Google Scholar -
Boot
W.,
Schmid
T.,
Este
M. D.,
Guillaume
O.,
Foster
A.,
Decosterd
L.,
A hyaluronic acid hydrogel loaded with gentamicin and vancomycin successfully eradicates chronic methicillin-resistant Staphylococcus aureus orthopedic infection in a sheep model. Antimicrobial agents and chemotherapy.
2021;
65
(4)
:
10-128
.
View Article Google Scholar -
Bakadia
B.M.,
Lamboni
L.,
Qaed Ahmed
A.A.,
Zheng
R.,
Ode Boni
B.O.,
Shi
Z.,
Antibacterial silk sericin/poly (vinyl alcohol) hydrogel with antifungal property for potential infected large burn wound healing: systemic evaluation. Smart Materials in Medicine.
2023;
4
:
37-58
.
View Article Google Scholar -
Grolman
J.M.,
Singh
M.,
Mooney
D.J.,
Eriksson
E.,
Nuutila
K.,
Antibiotic-Containing Agarose Hydrogel for Wound and Burn Care. Journal of Burn Care & Research ; Official Publication of the American Burn Association.
2019;
40
(6)
:
900-6
.
View Article PubMed Google Scholar -
Sani
E.S.,
Lara
R.P.,
Aldawood
Z.,
Bassir
S.H.,
Nguyen
D.,
Kantarci
A.,
An Antimicrobial Dental Light Curable Bioadhesive Hydrogel for Treatment of Peri-Implant Diseases. Matter.
2019;
1
(4)
:
926-44
.
View Article PubMed Google Scholar -
Norahan
M.H.,
Pedroza-González
S.C.,
Sánchez-Salazar
M.G.,
Álvarez
M.M.,
Trujillo de Santiago
G.,
Structural and biological engineering of 3D hydrogels for wound healing. Bioactive Materials.
2022;
24
:
197-235
.
View Article PubMed Google Scholar -
Raafat
D.,
Sahl
H.G.,
Chitosan and its antimicrobial potential\textemdasha critical literature survey. Microbial Biotechnology.
2009;
2
(2)
:
186-201
.
View Article PubMed Google Scholar -
Kumar
P.S.,
Lakshmanan
V.K.,
Anilkumar
T.,
C. Ramya,
P. Reshmi,
A.G. Unnikrishnan,
S.V. Nair,
R. Jayakumar,
Flexible and microporus chitosan hydrogel/nano-ZnO composite bandages for wound dressing: in vitro and in vivo evaluation. ACS applied materials & interfaces.
2012;
4
(5)
:
2618-29
.
View Article PubMed Google Scholar -
Sun
G.,
Zhang
X.,
Shen
Y.I.,
Sebastian
R.,
Dickinson
L.E.,
Fox-Talbot
K.,
Dextran hydrogel scaffolds enhance angiogenic responses and promote complete skin regeneration during burn wound healing. Proceedings of the National Academy of Sciences of the United States of America.
2011;
108
(52)
:
20976-81
.
View Article PubMed Google Scholar -
Mariia
K.,
Arif
M.,
Shi
J.,
Song
F.,
Chi
Z.,
Liu
C.,
Novel chitosan-ulvan hydrogel reinforcement by cellulose nanocrystals with epidermal growth factor for enhanced wound healing: in vitro and in vivo analysis. International Journal of Biological Macromolecules.
2021;
183
:
435-46
.
View Article PubMed Google Scholar -
Kondo
S.,
Kuroyanagi
Y.,
Development of a wound dressing composed of hyaluronic acid and collagen sponge with epidermal growth factor. Journal of Biomaterials Science. Polymer Edition.
2012;
23
(5)
:
629-43
.
View Article PubMed Google Scholar -
Kim
Y.S.,
Lew
D.H.,
Tark
K.C.,
Rah
D.K.,
Hong
J.P.,
Effect of recombinant human epidermal growth factor against cutaneous scar formation in murine full-thickness wound healing. Journal of Korean Medical Science.
2010;
25
(4)
:
589-96
.
View Article PubMed Google Scholar -
Wang
W.,
Lin
S.,
Xiao
Y.,
Huang
Y.,
Tan
Y.,
Cai
L.,
Acceleration of diabetic wound healing with chitosan-crosslinked collagen sponge containing recombinant human acidic fibroblast growth factor in healing-impaired STZ diabetic rats. Life Sciences.
2008;
82
(3-4)
:
190-204
.
View Article PubMed Google Scholar -
Xu
K.,
An
N.,
Zhang
H.,
Zhang
Q.,
Zhang
K.,
Hu
X.,
Sustained-release of PDGF from PLGA microsphere embedded thermo-sensitive hydrogel promoting wound healing by inhibiting autophagy. Journal of Drug Delivery Science and Technology.
2020;
55
.
View Article Google Scholar -
Liu
H.,
Wang
C.,
Li
C.,
A functional chitosan-based hydrogel as a wound dressing and drug delivery system in the treatment of wound healing. RSC advances..
2018;
8
(14)
:
7533-49
.
View Article Google Scholar -
Duncan
C.O.,
Shelton
R.M.,
Navsaria
H.,
Balderson
D.S.,
Papini
R.P.,
Barralet
J.E.,
In vitro transfer of keratinocytes: comparison of transfer from fibrin membrane and delivery by aerosol spray. Journal of Biomedical Materials Research. Part B, Applied Biomaterials.
2005;
73
(2)
:
221-8
.
View Article PubMed Google Scholar -
Seland
H.,
Gustafson
C.J.,
Johnson
H.,
Junker
J.P.,
Kratz
G.,
Transplantation of acellular dermis and keratinocytes cultured on porous biodegradable microcarriers into full-thickness skin injuries on athymic rats. Burns.
2011;
37
(1)
:
99-108
.
View Article PubMed Google Scholar -
Wood
F.M.,
Giles
N.,
Stevenson
A.,
Rea
S.,
Fear
M.,
Characterisation of the cell suspension harvested from the dermal epidermal junction using a ReCell kit. Burns.
2012;
38
(1)
:
44-51
.
View Article PubMed Google Scholar -
Jorgensen
A.M.,
Mahajan
N.,
Atala
A.,
Murphy
S.V.,
Advances in skin tissue engineering and regenerative medicine. Journal of Burn Care & Research.
2023;
44
(Supplement_1)
:
33-s41
.
View Article Google Scholar -
Skardal
A.,
Zhang
J.,
McCoard
L.,
Oottamasathien
S.,
Prestwich
G.D.,
Dynamically crosslinked gold nanoparticle–hyaluronan hydrogels. Advanced materials.
2010;
22
(42)
:
4736-440
.
View Article Google Scholar -
Hu
P.,
Yang
Q.,
Wang
Q.,
Shi
C.,
Wang
D.,
Mesenchymal stromal cells-exosomes: a promising cell-free therapeutic tool for wound healing and cutaneous regeneration. Burns & trauma.
2019;
7
:
38
.
View Article Google Scholar -
Williams
F.N.,
Herndon
D.N.,
Metabolic and Endocrine Considerations After Burn Injury. Clinics in Plastic Surgery.
2017;
44
(3)
:
541-53
.
View Article PubMed Google Scholar -
Zeng
W.,
Li
Y.,
Zeng
G.,
Yang
B.,
Zhu
Y.,
Transplantation with cultured stem cells derived from the human amniotic membrane for corneal alkali burns: an experimental study. Annals of Clinical and Laboratory Science.
2014;
44
(1)
:
73-81
.
PubMed Google Scholar -
Yan
G.,
Sun
H.,
Wang
F.,
Wang
J.,
Wang
F.,
Zou
Z.,
Topical application of hPDGF-A-modified porcine BMSC and keratinocytes loaded on acellular HAM promotes the healing of combined radiation-wound skin injury in minipigs. International Journal of Radiation Biology.
2011;
87
(6)
:
591-600
.
View Article PubMed Google Scholar -
Jorgensen
A.M.,
Yoo
J.J.,
Atala
A.,
Solid Organ Bioprinting: Strategies to Achieve Organ Function. Chemical Reviews.
2020;
120
(19)
:
11093-127
.
View Article PubMed Google Scholar -
Kassin
V.,
Gerasimova
L.I.,
Nikolaev
A.V.,
Shapiro
A.M.,
Slutskiĭ
L.I.,
Mukhamedzhanova
R.F.,
Use of Regenkur hydrogel in the local treatment of burns. Klinicheskaia Khirurgiia.
1991;
1
(4)
:
5-7
.
-
Misterka
S.,
[Clinical evaluation of hydrogel-type dressing materials after their 8-year use]. Polimery w Medycynie.
1991;
21
(1-2)
:
23-30
.
PubMed Google Scholar -
Homann
H.H.,
Rosbach
O.,
Moll
W.,
Vogt
P.M.,
Germann
G.,
Hopp
M.,
A liposome hydrogel with polyvinyl-pyrrolidone iodine in the local treatment of partial-thickness burn wounds. Annals of Plastic Surgery.
2007;
59
(4)
:
423-7
.
View Article PubMed Google Scholar -
Hauser
J.,
Rossbach
O.,
Langer
S.,
Vogt
P.,
Germann
G.,
Steinau
H.U.,
[Local therapy of grade IIa burns: efficacy and tolerability of a new hydrosome wound gel for the local treatment of grade IIa burns as compared with silver sulfadiazine ointment]. Der Unfallchirurg.
2007;
110
(11)
:
988-94
.
View Article PubMed Google Scholar -
Wang
Z.Y.,
Zhang
Q.,
Liao
Z.J.,
Han
C.M.,
Lv
G.Z.,
Luo
C.Q.,
Effect of recombinant human granulocyte-macrophage colony stimulating factor on wound healing in patients with deep partial thickness burn. Chinese journal of burns.
2008;
24
(2)
:
107-10
.
-
Glat
P.M.,
Kubat
W.D.,
Hsu
J.F.,
Copty
T.,
Burkey
B.A.,
Davis
W.,
Randomized clinical study of SilvaSorb gel in comparison to Silvadene silver sulfadiazine cream in the management of partial-thickness burns. Journal of Burn Care & Research ; Official Publication of the American Burn Association.
2009;
30
(2)
:
262-7
.
View Article PubMed Google Scholar -
Grippaudo
F.R.,
Carini
L.,
Baldini
R.,
Procutase versus 1% silver sulphadiazine in the treatment of minor burns. Burns.
2010;
36
(6)
:
871-5
.
View Article PubMed Google Scholar -
Yan
H.,
Chen
J.,
Peng
X.,
Recombinant human granulocyte-macrophage colony-stimulating factor hydrogel promotes healing of deep partial thickness burn wounds. Burns.
2012;
38
(6)
:
877-81
.
View Article PubMed Google Scholar -
Shahzad
M.N.,
Ahmed
N.,
Effectiveness of Aloe Vera gel compared with 1% silver sulphadiazine cream as burn wound dressing in second degree burns. JPMA. The Journal of the Pakistan Medical Association.
2013;
63
(2)
:
225-30
.
PubMed Google Scholar -
Yang
H.Z.,
Wang
W.K.,
Yuan
L.L.,
Wang
S.B.,
Luo
G.X.,
Wu
J.,
Multi-center clinical trial of FLAMIGEL (hydrogel dressing) for the treatment of residual burn wound. Chinese Journal of Burns.
2013;
29
(2)
:
177-80
.
-
Yim
H.,
Yang
H.T.,
Cho
Y.S.,
Kim
D.,
Kim
J.H.,
Chun
W.,
A clinical trial designed to evaluate the safety and effectiveness of a thermosensitive hydrogel-type cultured epidermal allograft for deep second-degree burns. Burns.
2014;
40
(8)
:
1642-9
.
View Article PubMed Google Scholar -
Yuan
L.,
Minghua
C.,
Feifei
D.,
Runxiu
W.,
Ziqiang
L.,
Chengyue
M.,
Study of the use of recombinant human granulocyte-macrophage colony-stimulating factor hydrogel externally to treat residual wounds of extensive deep partial-thickness burn. Burns.
2015;
41
(5)
:
1086-91
.
View Article PubMed Google Scholar -
Bobba
S.,
Chow
S.,
Watson
S.,
Girolamo
N. Di,
Clinical outcomes of xeno-free expansion and transplantation of autologous ocular surface epithelial stem cells via contact lens delivery: a prospective case series. Stem Cell Research & Therapy.
2015;
6
(1)
:
23
.
View Article PubMed Google Scholar -
Liu
J.,
Liao
Z.J.,
Zhang
Q.,
Phase Ⅳ clinical trial for external use of recombinant human granulocyte-macrophage colony-stimulating factor gel in treating deep partial-thickness burn wounds. Chinese journal of burns..
2016;
32
(9)
:
542-8
.
-
Meuli
M.,
Hartmann-Fritsch
F.,
Hüging
M.,
Marino
D.,
Saglini
M.,
Hynes
S.,
A Cultured Autologous Dermo-epidermal Skin Substitute for Full-Thickness Skin Defects: A Phase I, Open, Prospective Clinical Trial in Children. Plastic and Reconstructive Surgery.
2019;
144
(1)
:
188-98
.
View Article PubMed Google Scholar -
Guigui
A.,
Mazet
R.,
Blaise
S.,
Cracowski
C.,
Beau-Guillaumot
M.,
Kotzki
S.,
Treprostinil Hydrogel Iontophoresis in Systemic Sclerosis-Related Digital Skin Ulcers: A Safety Study. Journal of Clinical Pharmacology.
2020;
60
(6)
:
758-67
.
View Article PubMed Google Scholar -
Holbert
M.D.,
Kimble
R.M.,
Chatfield
M.,
Griffin
B.R.,
Effectiveness of a hydrogel dressing as an analgesic adjunct to first aid for the treatment of acute paediatric burn injuries: a prospective randomised controlled trial. BMJ Open.
2021;
11
(1)
:
e039981
.
View Article PubMed Google Scholar -
Shang
N.S.,
Cui
B.H.,
Wang
C.,
Gao
H.,
Xu
B.,
Zhao
R.,
A prospective randomized controlled study of the application effect of hydrogel dressings on deep partial-thickness burn wounds after dermabrasion and tangential excision. Chinese Journal of Burns.
2021;
37
(11)
:
1085-9
.
-
Çelik
E.,
Akelma
H.,
Hydrogel burn dressing effectiveness in burn pain. Burns.
2024;
50
(1)
:
190-6
.
-
Vogt
P.M.,
Hauser
J.,
Rossbach
O.,
Bosse
B.,
Fleischer
W.,
Steinau
H.U.,
Polyvinyl pyrrolidone‐iodine liposome hydrogel improves epithelialization by combining moisture and antisepis. A new concept in wound therapy. Wound Repair and Regeneration.
2001;
9
(2)
:
116-22
.
View Article Google Scholar -
Vogt
P.M.,
Reimer
K.,
Hauser
J.,
Rossbach
O.,
Steinau
H.U.,
Bosse
B.,
PVP-iodine in hydrosomes and hydrogel - novel concept in wound therapy leads to enhanced epithelialization and reduced loss of skin grafts. Burns.
2006;
32
(6)
:
698-705
.
View Article PubMed Google Scholar -
Zhang
L.,
Chen
J.,
Han
C.,
A multicenter clinical trial of recombinant human GM‐CSF hydrogel for the treatment of deep second‐degree burns. Wound repair and regeneration.
2009;
17
(5)
:
685-9
.
View Article Google Scholar -
Wang
H.,
Zhou
S.,
Guo
L.,
Wang
Y.,
Intelligent hybrid hydrogels for rapid in situ detection and photothermal therapy of bacterial infection. ACS Applied Materials & Interfaces.
2020;
12
(35)
:
39685-94
.
View Article Google Scholar -
Arafa
A.A.,
Nada
A.A.,
Ibrahim
A.Y.,
Zahran
M.K.,
Hakeim
O.A.,
Greener therapeutic pH-sensing wound dressing based on Curcuma Longa and cellulose hydrogel. European Polymer Journal.
2021;
159
:
110744
.
View Article Google Scholar -
Kim
M.H.,
Park
H.,
Nam
H.C.,
Park
S.R.,
Jung
J.Y.,
Park
W.H.,
Injectable methylcellulose hydrogel containing silver oxide nanoparticles for burn wound healing. Carbohydrate Polymers.
2018;
181
:
579-86
.
View Article PubMed Google Scholar -
Research
K.B.V.,
Global Hydrogel Dressing Market Size, Share & Industry Trends Analysis Report By Application, By End-use, By Product, By Regional Outlook and Forecast, 2022–2028. 2022.
.
Comments
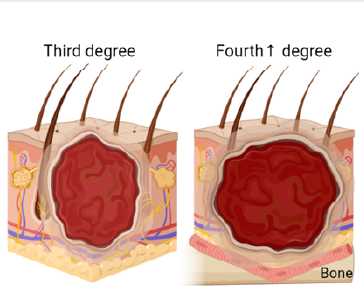
Article Details
Volume & Issue : Vol 12 No 3 (2025)
Page No.: 7207-7223
Published on: 2025-03-31
Citations
Copyrights & License

This work is licensed under a Creative Commons Attribution 4.0 International License.
Search Panel
- HTML viewed - 476 times
- PDF downloaded - 148 times
- XML downloaded - 24 times